ABSTRACT
Since the initial isolation of few-layer graphene, a plethora of two-dimensional atomic crystals has become available, covering almost all known materials types including metals, semiconductors, superconductors, ferro- and antiferromagnets. These advances have augmented the already existing variety of two-dimensional materials that are routinely realized by quantum confinement in bulk-semiconductor heterostructures. This review focuses on the type of material for which two-dimensional realizations are still being actively sought: magnetoelectrics. We present an overview of current theoretical expectation and experimental progress towards fabricating low-dimensional versions of such materials that can be magnetized by electric charges and polarized electrically by an applied magnetic field – unusual electromagnetic properties that could be the basis for various useful applications. The interplay between spatial confinement and magnetoelectricity is illustrated using the paradigmatic example of magnetic-monopole fields generated by electric charges in or near magnetoelectric media. For the purpose of this discussion, the image-charge method familiar from electrostatics is extended to solve the boundary-value problem for a magnetoelectric medium in the finite-width slab geometry using image dyons, i.e. point objects having both electric and magnetic charges. We discuss salient features of the magnetoelectrically induced fields arising in the thin-width limit.
Graphical abstract
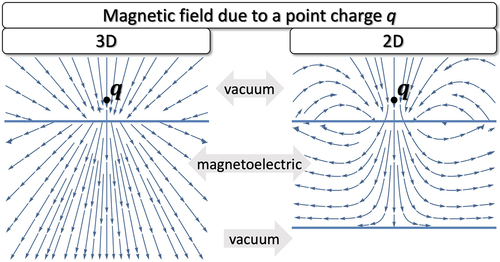
Disclosure statement
No potential conflict of interest was reported by the author(s).
Correction Statement
This article has been corrected with minor changes. These changes do not impact the academic content of the article.
Notes
1. Despite being sometimes loosely linked with magnetoelectricity, the current-induced magnetization [Citation63] that is possible in gyrotropic conductors and the chiral magnetic effect exhibited by Weyl semimetals [Citation28,Citation29] are fundamentally different from the equilibrium-magnetoelectric responses focused on here.
2. The first scenario where the application of a magnetic field causes the quadrupolar equilibrium currents that are the basis for magnetoelectricity technically constitutes a field-induced (i.e. higher-order) magnetoelectric effect [Citation60,Citation132]. Note also that the mechanism for magnetoelectricity to occur via equilibrium quadrupolar currents in a ferromagnetic-semiconductor quantum well is fundamentally different from the magnetoelectric response arising from the charge-density tunability of the spontaneous magnetization discussed in Sec. 2.3.
3. To be precise, even-layer MnBiTe
is actually antiferromagnetic and therefore not a time-reversal-invariant topological insulator but an axion insulator [Citation27]. This distinction matters because the fate of axion electrodynamics in the 2D limit of nonmagnetic topological-insulator slab geometries is rather intricate [Citation25].
4. Strictly speaking, the magnetoelectric response of CrO
is not isotropic but uniaxial, i.e. described by a tensor
with
[Citation155–157]. In our model for a realistic isotropic magnetoelectric, we use the value of
[Citation22].