ABSTRACT
Anemophilous species depend on wind for sexual reproduction. When plants do not receive enough pollen and/or pollen has poor quality, they may experience pollen limitation. This can reduce the production of fruits and seeds with negative consequences on population dynamics. The self-compatibility system also plays an important role on the likelihood of a species suffering pollen limitation as it determines if plants can produce fruits and seeds in the absence of compatible mates. Here, we studied the breeding system of the Andean anemophilous tree Polylepis incarum, a critically endangered species endemic to Lake Titicaca. We conducted controlled experimental pollination experiments and florescence microscopy analysis to assess whether pollen limitation and the incompatibility system constrain fruit and seed production. Results showed that the seed set is limited by pollen availability, that P. incarum exhibits a pre-zygotic compatibility system and early inbreeding depression manifested during seed development. Therefore, self-pollination is a factor that could be partially associated with the production of non-viable seeds.
Graphical abstract
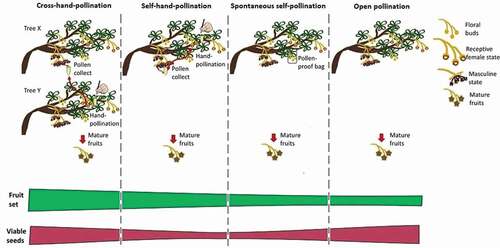
Resumen
Las especies anemófilas dependen del viento para la reproducción sexual. Cuando las plantas no reciben suficiente polen y/o éste es de baja calidad, pueden experimentar limitación de polen. Esto puede reducir la producción de frutos ysemillas con consecuencias negativas en la dinámica de las poblaciones. El sistema de compatibilidad también juega un papel importante en la probabilidad de que una especie sufra limitación de polen, ya que determina si las plantas pueden producir frutos ysemillas en ausencia de plantas compatibles. Estudiamos el sistema de reproducción del árbol anemófilo andino Polylepis incarum, una especie en peligro critico de extinción yendémica de el Lago Titicaca. Realizamos experimentos controlados de polinización experimental yanálisis de microscopía de fluorescencia para evaluar si la limitación del polen yel sistema de incompatibilidad están involucrados en la producción de frutos ysemillas. Los resultados mostraron que la producción de semillas está limitada por la disponibilidad de polen, que P.incarum exhibe un sistema de compatibilidad pre-cigótico yuna depresión endogámica temprana manifestada durante el desarrollo de la semilla. Por lo tanto, la autopolinización es un factor que podría estar parcialmente asociada con la producción de semillas inviables.
Introduction
Anemophilous species depend on wind to transport pollen grains to stigmas for sexual reproduction. However, an inadequate quantity or quality of pollen can reduce plant reproductive success, manifested in a lower production of fruits and seeds (i.e. pollen limitation) [Citation1–3]. Although pollen limitation has been studied extensively in the last decades [Citation4], there are still large knowledge gaps regarding if the extent of pollen limitation is the product of temporal variability of the pollen recipient, resource availability or if it is the product of adaptations to stochastic pollinator environments [Citation1,Citation2,Citation5–7]. Furthermore, the increasing tendencies of land-use intensification, habitat degradation, and climate change add urgency to understanding how anthropogenic perturbations impact pollen limitation and its long-term consequences on reproduction, abundance, and distribution of plant species [Citation8–12].
The self-compatibility system also plays an important role on reproductive output and, as a consequence, on the degree of pollen limitation [Citation13]. For example, in the absence of compatible mates, self-incompatible species are less likey to produce offspring. In this sense, anemophilous species rely on wind for pollen dispersal but also on the availability of compatible mates [Citation14,Citation15]. As a consequence, anemophilous self-incompatible species are comparatively more likely to suffer from pollen limitation than self-compatible species, if potential mates are scarce or unavailable [Citation1,Citation3].
The subtribe Sanguisorbinae (Sanguisorbeae, Rosoideae) contrasts with most of Rosaceae species as flower morphology suggest that anemophily is the main pollination mating system in the group [Citation16,Citation17]. Flowers characterized by being small, tetramerous or trimerous and commonly unisexual, with absent petals [Citation16,Citation17]. Additionally, stamens are long and gynoecia is composed by a single carpel with a dry feathery style. These characteristics have been found in Acaena, Cliffortia, Margyricarpus, Polylepis, and Sanguisorba. Comparative studies have also suggested that anemophily evolved independently in the group and it could be advantageous in areas where animal pollinators are scarce and thus enhance reproductive success of the species [Citation18,Citation19].
Polylepis Ruiz & Pavon, commonly known as queñua or quewiña, is distributed across the Andean highlands in small and fragmented woodlands [Citation20,Citation21]. The genus includes between 20 and 40 species of small trees and shrubs [Citation22,Citation23] from which a large majority are undergoing some degree of population decline, mostly associated with anthropogenic activities and climate change [Citation20,Citation24–26]. High production of non-viable seeds has been commonly found in the genus, which has been attributed to pollen limitation and parthenocarpy as well as to habitat degradation and resource limitation [Citation27–29]. However, the breeding system of Polylepis has been studied in very few species [Citation30,Citation31] and it could include a diversity of breeding systems, from obligate outcrossing species to autonomously selfing species. Here, we focus on understating the reproductive system of Polylepis incarum (Bitter) M. Kessler & Schmidt-Leb by testing if seed production is limited by pollen availability and by characterizing the incompatibility system of the species.
In this study, we investigated the breeding system of the Andean anemophilous tree P. incarum using controlled experimental pollination and florescence microscopy analysis of post-pollinated flowers. We selected P. incarum because the species is endemic to Lake Titicaca, and the few remaining woodlands are threatened by land-use intensification [Citation32–34]. We hypothesized that if pollen limitation is taking place, we would expect to find a lower fruit set in openly pollinated flowers in comparison to hand pollinated flowers. If the species is self-incompatible, we would expect to find a lower seed set and possibly lower pollen germination and pollen tubes reaching the style and ovary in self-pollinated flowers in comparison to cross-pollinated flowers.
Methods
Study area
The study was carried out in Kopacati, near Lake Titicaca, La Paz, Bolivia. The region is cold and semi-arid with a mean annual temperature of 8 °C and a total annual precipitation of 758 mm [Citation34]. Daily temperature ranges between 7 and 15 °C and it can decrease up to 0 °C at night. The dry season occurs between April and November and the wet season between December and March, when 65–78% of the annual precipitation falls.
We selected one P. incarum woodland (16°34ʹ24 “S, 68°04ʹ52.5” W), located between 3860–4082 m asl with an area of 1.73 ha. The vegetation is characteristic of the humid Puna, where P. incarum is the dominant tree species, but planted trees of Budleja coriacea (native) and the Eucalyptus globulus (exotic) are common. The understory of the P. incarum woodlands is composed of shrubs (Baccharis tola, Chersodoma jodopappa and Chuquiragua jussieui), tussock grasses (Jarava ichu, Festuca spp.), and small herbs (Luminus microphyllus, Lachemilla pinnata, Bartsia crenaloba) [Citation35]. Polylepis forests are commonly used as areas for small-scale agriculture, grazing areas for sheep, and as a source of firewood [Citation34,Citation36].
Study species
Polylepis incarum includes small trees and shrubs. The species is distributed exclusively around Lake Titicaca in Peru and Bolivia [Citation23]. P. incarum is commonly found in small groups of individuals along farming plots and as isolated trees near houses. In Bolivia, it has been estimated that the species covers only 45 ha, including only five woodland fragments [Citation34]. Plants produce pendant inflorescences, bearing up to eight flowers. Flowers are bisexual, small, lack petals, possess inconspicuous sepals, large anthers and a gynoecium comprising a large, dry stigma, and an ovary that bears a single ovule. Flowers are protogynous. The flowering season takes place between the end of the dry season and the beginning of the rainy season (July to October) [Citation37]. Fruits are winged achenes that contain a single seed, dispersed by wind from December to January [Citation37]. Seeds germinate after 7–15 days.
Pollen viability
To assess the pollen viability of P. incarum, 30 trees were randomly selected during the peak of the flowering season (September 2016). We extracted pollen from four inflorescences from each tree, after 24 hours pollen grains were stained using a tetrazolium and a sucrose solution [Citation38]. Pollen grains were observed under a microscope with 40x magnification. We quantified pollen viability by randomly selecting fields of view in a slide, until we counted a total 100 pollen grains. We quantified pollen viability on four slides (replicates) for each sample. Pollen grains were considered viable when they were stained red.
Reproductive system
To determine if fruit set of P. incarum was limited by pollen availability, we conducted field experiments during the flowering season in September of 2016. During the peak production of floral buds (August 2016), we marked four inflorescences from each of 15 trees and we covered them with pollen-proof bags (glassine shoot bags manufactured by the Seedburo Equipment Company, IL, USA). Once the flowers were mature, specifically during the receptive female reproductive phase, we applied four pollination treatments to each plant. In “Hand self-pollination”, we used pollen from a different flower from the same plant. In “Hand cross-pollination”, we used pollen from a different plant randomly selected within the same population. In “Open pollination”, flowers were left exposed. And in “spontaneous self-pollination”, floral buds were bagged without pollination and left intact until the end of the experiment. Flowers from the hand self-, cross-, and open pollination were carefully emasculated by removing the stamens using a pair of tweezers and mini dissecting scissors and covered them with pollination bags until they developed fully ripen fruits (February 2017).
Mature fruits were stored in paper bags and taken to the Instituto de Biología Molecular (Universidad Mayor de San Andres, La Paz, Bolivia). We dissected fruits to determine the presence of seeds and seed viability. The determination of the seed viability was carried out following the International Seed Testing Association protocol (ISTA) [Citation39]. Each seed was soaked in 0.5% tetrazolium (2,3,5 triphenyl chloride) for 24 hours in the dark. Seeds were subsequently dissected and observed under a stereo microscope at 20X magnification and classified into one of the following categories: 1) seed with viable embryo and endosperm present, 2) seed with endosperm and aborted embryo, 3) seed without endosperm and nonviable embryo and 4) seed without endosperm and aborted embryo [Citation40] ().
Pollen tube growth test
To assess stigmatic receptivity in P. incarum, we conducted an additional hand pollination experiment. We performed the same pollination treatments (open, self, and cross-pollination) on the same 15 trees described above. Subsequently, inflorescences were collected after 48 hours and fixed in a 3:1 solution of acetic acid and formaldehyde for 2 hours and transferred to 70% alcohol for permanent storage.
Since P. incarum flowers have a coriaceous consistency, each flower was soaked in 1 N HCl solution for 30 minutes to soften the tissues and facilitate dissection [Citation41]. Subsequently, they were washed with distilled water, immersed in a phosphate buffer pH 9.5 and stained with 1% aniline blue. We dissected each flower, separated the gynoecium and stored it in a refrigerator for 24 hours. We observed carpels under a Leica LB2 microscope with fluorescent light and a UV-2A violet filter [Citation42]. For each flower, we quantified: 1) number of grains on the stigma surface, 2) number of germinated pollen grains, 3) number of pollen tubes and 4) number of pollen tubes that entered the ovule.
Data analysis
To determine the reproductive incompatibility system of P. incarum, we calculated the Index of self-incompatibility (ISI) by dividing the average number of seeds produced by hand self-pollination to the average number of seeds produced by hand cross-pollination [Citation1]. This index is a criterion that estimates self-incompatibility from fruit production [Citation43]. The obtained values were interpreted as follows: ISI> 1, self-compatible; 1> ISI> 0.2, partially self-compatible; ISI <0.2, mostly self-incompatible; or 0, completely self-incompatible.
We applied a one-way ANOVA to determine if there were differences in the total number of fruits produced between hand pollination and open pollination treatments. Additionally, we carried out an ANOVA to assess if there were differences in the percentage of viable seeds between hand pollination and open pollination treatments. A one-way ANOVA was applied to determine if there were differences in the percentage of germinated pollen grains, pollen tubes in the style, and pollen tubes reaching ovary between open and hand pollination treatments. Additionally, a linear regression was performed to identify the relationship between the number of pollen grains in the stigma and the proportion of pollen tubes in the style. All analyzes were performed in R 3.5.1.
Results
Pollen viability
A total of 12,000 pollen grains of P. incarum were evaluated. Pollen viability was relatively high as 69.56% of the pollen grains were viable ().
Pollen limitation
The addition of pollen increased seed set in P. incarum (F2, 87 = 7.75, P < 0.0001; and ). Open pollinated flowers produced a lower percentage of seeds (84.4 ± 1.8 SD) in comparison to flowers that were hand self-pollinated (96.2 ± 8.4) and hand cross-pollinated (97.5 ± 9.4). Spontaneous self-pollinated flowers also produced a significantly lower percentage of seeds (84.4 ± 3.3) in comparison to hand pollinated flowers.
Figure 3. Percentage of fruit set following cross-hand-pollination (CP), open pollination (OP) self-hand-pollination (SP) and spontaneous self-pollination (SPO) of Polylepis incarum. Different letters indicate significant differences (P < 0.01)
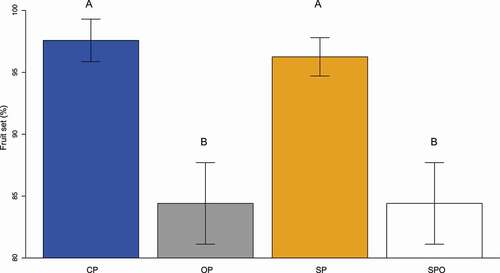
Table 1. Percentage of viable seeds (Mean ± SD) produced by cross-hand-pollinated, open-pollinated, self-pollinated, and spontaneous self-pollinated flowers produced by Polylepis incarum in western Bolivia
All the fruits contained seeds, but the percentage of viable seeds was significantly different between treatments (F2,132 = 3.116, P < 0.001; and ). Open-pollinated flowers (55.4 ± 12.8) and hand cross-pollinated flowers (56.9 ± 13.4) exhibited a significantly higher percentage of viable seeds than hand self-pollinated flowers (33. ± 17.97) and spontaneous self-pollinated flowers (41.3 ± 19.47). We found a large percentage of non-viable seeds (83.28 %), regardless of the pollination treatment, characterized by containing endosperm with a dry, non-viable or absent embryo ().
Figure 4. Percentage of viable and non-viable seeds following cross-hand-pollination (CP), open pollination (OP), self-hand-pollination (SP) and spontaneous self-pollination (SSP) of Polylepis incarum. Asterisks indicate significant differences among treatments at P < 0.01
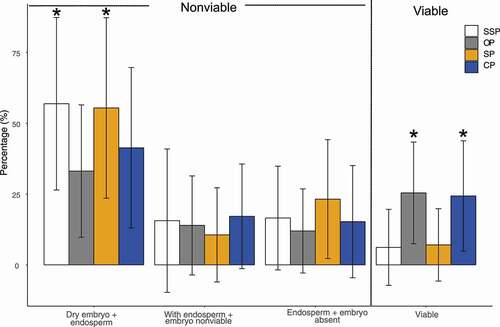
Stigmatic receptivity
The ISI of P. incarum was 0.9, categorizing the species as self-compatible. Epifluorescence analysis showed a similar percentage of germinated grains on the stigma surface between treatments (F2,132 = 1.3, P = 0.26) (). The percentage of pollen tubes that penetrated the style (F2,132 = 1.3, P = 0.26) and the ovule (F2,132 = 1.3, P = 0.26) were also similar between treatments ().
Discussion
We conducted a first comprehensive study on the reproductive biology of Polylepis incarum (Bitter) M. Kessler & Schmidt-Leb. The results provide evidence suggesting that the production of seeds is limited by pollen availability, that the species exhibits a pre-zygotic compatibility system, and early inbreeding depression manifested during seed development.
Pollen viability of P. incarum was approximately 70%, similar to those reported in P. australis (86%) [Citation30]. The results suggest that pollen viability is not associated with a large production of non-viable seeds in P. incarum. Some authors attribute a decrease in pollen germination or in the formation of viable fruits to gametophytic incompatibility mechanisms that delay or prevent fertilization, or increase inbreeding depression [Citation30,Citation44,Citation45].
Pollen supplementation increased seed set in hand pollinated flowers, regardless of the source of the pollen, as hand self- and cross-pollinated flowers produced a higher percentage of seeds than openly pollinated flowers. Our results contrast with studies in P. australis and P. tomentella where pollen supplementation did not increase seed set [Citation30,Citation31]. Despite P. incarum possess several demographic and reproductive strategies that could ensure fertilization of female flowers, such as high density of reproductive individuals in the same population and high synchronization of flowering during the dry season, our results suggest that the amount of pollen available may not be sufficient to guarantee sexual reproduction [Citation34,Citation37]. The lower production of seeds by openly pollinated flowers could be associated with the small area currently covered by P. incarum woodlands and habitat degradation of the remaining populations, associated with land-use intensification, particularly small-scale farming, overgrazing, and firewood extraction.
The results of the hand pollination and the stigmatic receptivity experiments in P. incarum also showed evidence of prezygotic compatibility, which has also been previously reported in P. australis and P. tomentella [Citation30,Citation31]. The flowers set fruits and seeds whether they were pollinated with pollen from the same plant or pollen from another plant. Self-fertility seems to be an advantageous reproductive strategy as it ensures fruit production in populations with a low density of reproductive individuals, in populations with individuals that have similar genotypes, and in isolated individuals [Citation46]. Additionally, a high percentage of the pollen grains from the same plant germinated on the stigma surface and developed pollen tubes that reached the style, but only a small percentage were able to penetrate the ovary, this results could be explained because the adequate conditions as humidity have not exist and pollen could loss viability in few days.
P. incarum also exhibits evidence of early inbreeding depression as a low percentage of seeds produced by self-pollinated flowers were viable, compared to seeds produced by cross- and open pollination. Most of the non-viable seeds from selfed seeds contained endosperm and an aborted embryo. In P. australis, selfing also decreased seed germination [Citation30], but no evidence has been found in P. tomentella as fruit size was similar between self- and cross-pollinated flowers [Citation31].
Despite P. incarum is able to secure seed set through selfing, self-pollination does not seem to be as efficient as cross-pollination due to lower seed set and lower seed quality. The large number of non-viable seeds produced by spontaneous selfing, characterized by seeds with an aborted embryo, suggests that embryo development was aborted before seeds reached maturity. Selfing produces seeds with embryos genetically identical to the maternal genotype and cross-pollination produces zygotic embryos genetically different from the maternal genotype. Consequently, their genetic make-up may affect other physiological properties of the seeds and there may be a competition between hybrid and selfed embryos which could affect their development. However, in order to confirm this hypothesis, detailed ontogenic studies should be conducted to compare seed development in selfed and hybrid fruits.
Finally, pollen addition experiments showed that P. incarum produces a low percentage of viable seeds, since only 18% of the seeds contained endosperm and a viable embryo. Low seed viability has also been reported in several Polylepis species (P. australis, P. neglecta, P. pacensis, and P. tomentella) [Citation27,Citation28,Citation40,Citation47]. Parthenocarpy and resource limitation are considered the main causes associated with low seed production [Citation28,Citation30], but there are still no studies that have developed a systematic evaluation of embryonic development of fruits and seeds.
In P. incarum, the low production of viable seeds cannot be attributed exclusively to fecundity and pollen limitation because ontogenic and genetic factors could also be playing an important role. To ensure the preservation of remnant forest patches, conservation efforts should go beyond the traditional emphasis on reforestation. Assisted restoration should also involve activities to increase connectivity between woodlands and isolated plants to ensure pollen movement and seed dispersal. Additionally, improving habitat quality will help to increase seedling recruitment and survival of reproductive plants.
Disclosure of potential conflicts of interest
No potential conflict of interest was reported by the author(s).
Author contributions
CL, AID and EG conceived and designed the study; CL and CM conducted field experiments; CL and JQ performed the epifluorescence analysis. CL and AID analyzed the data and wrote a preliminary version of the manuscript. EG, JQ, CM, and SG revised the manuscript thoroughly.
Acknowledgments
We thank Victor López, Yara Fernández, and Lucia Blanco for field assistance. We also thank Sergio Gutierrez and Volga Iñiguez for the logistic support. This study was funded by a grant awarded to Cecilia Lopez from the Instituto de Ecología and to Jorge Quezada for the project “Germination of Polylepis species for seedling production to reforest green urban areas of La Paz, Bolivia” awarded Universidad Mayor de San Andrés. We also thank to reviewers for their helpful comments.
Additional information
Funding
References
- Burd M. Pollen limitation is common—should it be? (a comment on Rosenheim et al., “Parental optimism versus parental pessimism in Plants: how common should we expect pollen limitation to be?”). Am Nat. 2016;187:388–396.
- Ashman T-L, Knight TM, Steets JA, et al. Pollen limitation of plant reproduction: ecological and evolutionary causes and consequences. Ecology. 2004;85:2408–2421.
- Knight TM, Steets JA, Vamosi JC, et al. Pollen limitation of plant reproduction: pattern and process. Annu Rev Ecol Evol Syst. 2005;36:467–497.
- Bennett JM, Steets JA, Burns JH, et al. GloPL, a global data base on pollen limitation of plant reproduction. Sci Data. 2018;5:180249.
- Haig D, Westoby M. On limits to seed production. Am Nat. 1988;131:757–759.
- Burd M, Ashman T-L, Campbell DR, et al. Ovule number per flower in a world of unpredictable pollination. Am J Bot. 2009;96:1159–1167.
- Rosenheim JA, Williams NM, Schreiber SJ. Parental optimism versus parental pessimism in plants: how common should we expect pollen limitation to be? Am Nat. 2014;184:75–90.
- Knight TM. The effects of herbivory and pollen limitation on a declining population of Trillium grandiflorum. Ecol Appl. 2004;14:915–928.
- Law W, Salick J, Knight TM. The effects of pollen limitation on population dynamics of snow lotus (Saussurea medusa and S. laniceps, Asteraceae): threatened Tibetan medicinal plants of the eastern Himalayas. Plant Ecol. 2010;210:343–357.
- Ehrlen J, Eriksson O. Pollen limitation and population growth in an herbaceous perennial legume. Ecology. 1995;76:652–656.
- Wang S-Q. Inbreeding and inbreeding depression of Paeonia decomposita (Paeoniaceae), a threatened endemic plant to China. Bot Stud. 2019;60:1–10.
- Van Etten ML, Tate JA, Anderson SH, et al. The compounding effects of high pollen limitation, selfing rates and inbreeding depression leave a New Zealand tree with few viable offspring. Ann Bot. 2015;116:833–843.
- Morgan MT, Wilson WG. Self‐fertilization and the escape from pollen limitation in variable pollination environments. Evolution. 2005;59:1143–1148.
- Agren J. Population size, pollinator limitation, and seed set in the self‐incompatible herb Lythrum salicaria. Ecology. 1996;77:1779–1790.
- Luijten SH, Dierick A, Gerard J, et al. Population size, genetic variation, and reproductive success in a rapidly declining, self‐incompatible perennial (Arnica montana) in The Netherlands. Conserv Biol. 2000;14:1776–1787.
- Kalkman C. The phylogeny of the Rosaceae. Bot J Linn Soc. 1988;98:37–59.
- Eriksson T, Hibbs MS, Yoder AD, et al. The phylogeny of Rosoideae (Rosaceae) based on sequences of the internal transcribed spacers (ITS) of nuclear ribosomal DNA and the trnL/F region of chloroplast DNA. Int J Plant Sci. 2003;164:197–211.
- Goodwillie C. Wind pollination and reproductive assurance in Linanthus parviflorus (Polemoniaceae), a self‐incompatible annual. Am J Bot. 1999;86:948–954.
- Culley TM, Weller SG, Sakai AK. The evolution of wind pollination in angiosperms. Trends Ecol Evol. 2002;17:361–369.
- Fjeldså J, Kessler M. Conserving the biological diversity of Polylepis woodlands of the highland of peru and Bolivia: a contribution to sustainable natural resource management in the andes. Copenhagen: NORDECO; 1996.
- Kessler M. The genus Polylepis (Rosaceae) in Bolivia. Candollea. 1995;50:131–171.
- Boza T, Taxonomic Studies in Polylepis (Rosaceae). Ph.D. dissertation. University of Zurich, 2020.
- Kessler M, Schmidt-Lebuhn AN. Taxonomical and distributional notes on Polylepis (Rosaceae). Org Divers Evol. 2006;6:67–70. .
- Cuyckens GAE, Christie DA, Domic AI, et al. Climate change and the distribution and conservation of the world’s highest elevation woodlands in the South American Altiplano. Glob Planet Change. 2016;137:79–87.
- Domic AI, Camilo GR, Capriles JM. Small-scale farming and grazing reduce regeneration of Polylepis tomentella (Rosaceae) in the semiarid Andes of Bolivia. Biotropica. 2014;46:106–113.
- Cierjacks A, Salgado S, Wesche K, et al. Post-fire population dynamics of two tree species in high-altitude Polylepis forests of central Ecuador. Biotropica. 2008;40:176–182.
- Seltmann P, Leyer I, Renison D, et al. Variation of seed mass and its effects on germination in Polylepis australis: implications for seed collection. New For. 2007;33:171–181.
- Renison D, Hensen I, Cingolani AM. Anthropogenic soil degradation affects seed viability in Polylepis australis mountain forests of central Argentina. For Ecol Manag. 2004;196:327–333.
- Renison D, Cingolani AM, Suarez R, et al. The restoration of degraded mountain woodlands: effects of seed provenance and microsite characteristics on Polylepis australis seedling survival and growth in Central Argentina. Restor Ecol. 2005;13:129–137.
- Seltmann P, Cocucci A, Renison D, et al. Mating system, outcrossing distance effects and pollen availability in the wind-pollinated treeline species Polylepis australis BITT. (Rosaceae). Basic Appl Ecol. 2009;10:52–60. .
- Domic AI, Bernhardt P, Edens-Meier R, et al. Pollination ecology of Polylepis tomentella (Rosaceae), an Andean anemophilous tree presenting a potential floral fungal infection. Int J Plant Sci. 2017;178:512–521.
- Arrázola S, Coronado I, Torrico L, et al. Polylepis incarum (Bitter). In: Kessler M, Scmidt-Leb, editors. Libro Rojo de la Flora Amenazada de Bolivia. Vol. I. Zona Andina, Ministerio de Medio Ambiente y Agua, p. 600/La Paz; 2012.
- Montesinos-Tubée DB, Pinto ÁC, Beltrán DF, et al. Vegetación de un bosque de Polylepis incarum (Rosaceae) en el distrito de Lampa, Puno, Perú. Rev Peru Biol. 2015;22:87–96.
- Domic AI, Palabral-Aguilera AN, Gómez MI, et al. Polylepis incarum (Rosaceae) una especie En Peligro Crítico en Bolivia: propuesta de reclasificación en base al área de ocupación y estructura poblacional. Ecol En Boliv. 2017;52:116–131.
- Hurtado R, Palabral-Aguilera AN, Domic AI, et al. Estudios etnobotánicos y florísticos de los bosques amenazados de Polylepis incarum y Polylepis pacensis (Rosaceae) en Bolivia. Bonplandia. 2018;27:113–126.
- Vega CK, Villegas CG, Rocabado PA, et al. Biología reproductiva de tres especies de Polylepis (P. neglecta, P. incarum y P. pacensis), con énfasis en su comportamiento germinativo. Ecol Austral. 2018;28:310–324.
- López-Alipaz CL, Domic AI, Mayta C, et al. Fenología reproductiva de la queñua (Polylepis incarum, Rosaceae) durante un ciclo anual en la puna mesofítica de La Paz. Bolivia Ecol Austral. 2018;28:301–309.
- Cavusoglu A, Sulusoglu M. In vitro pollen viability and pollen germination in medlar (Mespilus germanica L.). Int Res J Biol Sci. 2013;2:49–53.
- de França-Neto JB, Krzyzanowski FC, de França-Neto JB, et al. Tetrazolium: an important test for physiological seed quality evaluation. J Seed Sci. 2019;41:359–366. .
- Vega Krstulovic C, Quezada Portugal J, Rocabado Koya P, et al. Assessment of seed quality and germination response in the species of the genus Polylepis. In: Jimenez-Lopez JC, editor. Seed dormancy and germination. IntechOpen; 2020. p. 1–15.
- Vance NC, Bernhardt P, Edens RM. Pollination and seed production in Xerophyllum tenax (Melanthiaceae) in the Cascade Range of central Oregon. Am J Bot. 2004;91:2060–2068.
- Bernhardt P, Knox RB, Calder DM. Floral biology and self-incompatibility in some Australian mistletoes of the genus Amyema (Loranthaceae). Aust J Bot. 1980;28:437–451.
- Zapata TR, Arroyo MTK. Plant reproductive ecology of a secondary deciduous tropical forest in Venezuela. Biotropica. 1978;10(3):221–230.
- Ishimizu T, Shinkawa T, Sakiyama F, et al. Primary structural features of rosaceous S-RNases associated with gametophytic self-incompatibility. Plant Mol Biol. 1998;37:931–941.
- Ushijima K, Sassa H, Dandekar AM, et al. Structural and transcriptional analysis of the self-incompatibility locus of almond: identification of a pollen-expressed F-box gene with haplotype-specific polymorphism. Plant Cell. 2003;15:771–781.
- Richards AJ. Plant Breeding Systems. Garland Sci. 1997;2:544.
- Domic AI, Capriles JM, Camilo GR. Evaluating the fitness effects of seed size and maternal tree size on Polylepis tomentella (Rosaceae) seed germination and seedling performance. J Trop Ecol. 2020;36:115–122.