ABSTARCT
Leaf traits are analyzed as essential drivers for the evolutionary and ecological role of plant defense mechanisms. Plants show leaf trait variation as a response to the diverse environmental conditions, like different successional stages. Those variations can impact leaf herbivory rates and drive changes in the allocation of plant resources. In this study, we aimed at comparing the expression of leaf defenses in established plants over different successional stages in a tropical dry forest to understand how these defenses modify the plant–herbivore interactions based on herbivory rates. We analyzed physical leaf traits (specific leaf area, thickness, and density), nutrient content (N, P, and K), total phenolic compounds, and leaf herbivory, of the native tree species Aspidosperma pyrifolium and Cenostigma pyramidale, in early- and late-successional stage areas. Results showed that the plant investment in defenses varies according to the successional stage and that both species have similar defense strategies, confirming the resource availability hypothesis. Individuals from the early stage adopt a strategy to lower sclerophylly, higher nutrient content and less phenolic compounds, while the late-stage individuals showed an opposite behaviour. For both species in this study, the average percentage of leaf herbivory observed was 40% higher in the early compared to the late-stage area. Our data indicate that plant defenses are tightly coupled to sclerophylly and investments in secondary metabolites, and the environmental conditions of different successional stage drive that plasticity in such leaf traits.
Introduction
Plant–insect interactions are essential for maintaining the biodiversity and the function in ecosystems [Citation1]. The production of phenolic compounds and a higher leaf sclerophylly are examples of the many traits that plants exhibit as defenses, aimed to reduce the loss of photosynthetic tissue due to possible herbivore attacks [Citation2,Citation3]. These characteristics are mainly influenced by environmental conditions, such as nutrient and soil water availability [Citation4–6].
Many hypotheses that describe the plant resource allocation and defense patterns have been postulated, with the “Resource Availability” being one of the most well known and mediated by the leaf economics spectrum [Citation7–9]. In pioneer plant species, plants invest more resources for growth, despite defensive leaf traits, making the replacement of tissue lost to herbivory more beneficial [Citation4,Citation7]. On the other hand, plant species in late successional stages often adopt a conservative (or slow maturation) growth approach by allocating more resources to the formation of tissues that have a higher longevity and providing additional protection against herbivores [Citation7]. Distinct ecological strategies are considered positive in the same successional stage, but usually the establishment of a plant species in different succession stages is limited by trade-offs [Citation10–12].
In the tropics, huge forest areas are used for farming and cattle raising, while other already-deforested areas are abandoned after usage, creating patches of forests under different successional stages [Citation13]. This anthropic disturbance has been widely observed in tropical dry forests, especially in South America [Citation14]. In Brazil, 80% of dry forests are in regeneration and 40% of these are maintained in the initial stages by constant land use [Citation15]. In areas undergoing natural regeneration, the plasticity displayed on leaf traits is an important strategy for plant acclimatization and establishment [Citation5]. These traits can be understood as morpho-physiophenological characteristics arising from the plants response to the environmental conditions [Citation2,Citation16,Citation17]. Variation in leaf traits has been shown to be involved in countless plant processes, including plant growth, and can also impact the herbivory rates observed along the succession stages [Citation2,Citation7]. Furthermore, through morphological and physiological adjustments, herbivore-inflicted pressure can also be considered an important factor for the development and evolution of these traits, including physical and chemical defenses, and tolerance of future herbivore attacks [Citation17,Citation18].
Among the leaf traits that have been widely used in studies about ecological attributes and plant defense we find at least six distinctive examples: a) leaf area (LA) which represents the balance between carbon assimilation and water loss through transpiration; b) specific leaf area (SLA), a trait that reflects the leaf building cost – especially in carbon – and is directly related to plant function [Citation18]; c) thickness and density, scleromorphic characteristics that have important relationships the leaf mechanical properties and are related to the leaf life span and plant–herbivore interactions [Citation19–21]; d) nutrient composition, such as nitrogen and phosphorus; e) secondary compounds – essential to protect the leaf epidermis against high light irradiance and herbivore attack [Citation22]. Thus, it is expected that the ecological strategies adopted by plant species under different circumstances can be inferred based on these leaf traits [Citation23,Citation24].
Studies linking the effects of herbivory-inflicted leaf damage with the different traits of the plants that manage to persist in different succession stages are still scarce. This is even more evident in the case of tropical dry forests. As herbivory can have a direct influence on forest ecosystems’ function and structure, it is crucial to learn how these interactions act in a landscape scale, as this information is useful for restoration and conservation strategies [Citation25]. Thus, the current study aims to compare the expression of leaf defenses of two tree species established over different successional stages and to investigate how these defenses modify the plant–herbivore interactions based on herbivory rates. We hypothesize that the leaf damage inflicted by herbivore insects in a tropical dry forest would be higher in early-successional plants due to a higher investment in growth than in the development of leaf defenses.
Material and methods
Study area
This study was conducted at Fazenda Tamanduá (06°59ʹ13” to 07°00ʹ14” S e 37°18ʹ08” to 37°20ʹ38” W, 240 m above sea level), a farm in the countryside of Santa Terezinha, Paraíba, Brazil (). With 3,073 ha, an average annual temperature of 32.8°C and an average annual precipitation of 600 mm. The rainfall episodes show an irregular pattern, which usually is condensed between February and May. During 2016 and 2017, the accumulated rainfall until April was, respectively, 590.3 mm and 531.6 mm (). The Lithic Neosol (i.e. soil class of little developed and shallow, reaching up to 50 cm in depth, being normally associated with rocky outcrops) is the predominant type in this area [Citation26].
Figure 1. Map of Brazil locating Paraiba state and the study area map (Fazenda Tamanduá). In details a google map of Fazenda Tamandua with the successional stages sites points. Historical series of annual rainfall (mm), accumulated precipitation from January to April (mm) and annual average air temperature (°C) over the last 10 years in the tropical dry forest (Santa Terezinha, Paraíba, Brazil)
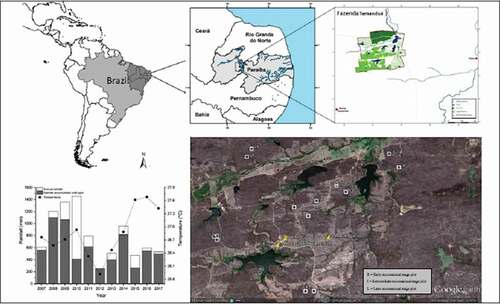
The farm has one of the biggest Caatinga conservation and protection areas in the Brazilian Northeast, with almost 1,000 ha of land (one-third of its total area) comprising a Legal Reserve with 614 ha, and a Private Natural Reserve – RPPN Tamanduá (350 ha) recognized by IBAMA, the country’s Federal body in charge of environment and natural resources [Citation27].
Nowadays, the land area is the property of Fazenda Tamanduá; therefore, it was originally composed by many small properties that cultivated perennial cotton plants and were abandoned during a widespread infestation by the cotton boll weevil in the 1980s [Citation27,Citation28]. The abandoned areas comprise a mosaic of different successional stages of tropical dry forests. The land-use history is well known, and there are no records of fertilizer usage in these places [Citation5,Citation6,Citation28]. Our research group established five permanent plots since 2006 in each different stages of ecological succession (early, intermediate and late) when all the trees (DBH > 3 cm) were identified and got an aluminum labels to mark each tree with one code (number and letter). The early-successional stage has a history of anthropic disturbance such as clearcutting, cotton cultivation, and cattle pasture, undergoing natural regeneration for approximately the last 20 years. It also displays a high prevalence species of herbs during the rainy season alongside species of shrubs and trees with a relatively sparse distribution. The richness of trees in the early area is represented by seven species and three families, among them Aspidosperma pyrifolium Art. & Zucc, Cenostigma pyramidale Tull. and Bauhinia cheilantha (Bong.) Steud. The mean shoot biomass production for the early succession stage area was 29.9 Mg ha−1 [Citation15,Citation28]. On the other hand, the late succession stage area () is remarkably free of clearcutting and signals of anthropic action or big disturbance since the 1950s. This area is inside a Private Natural Reserve, and the vegetation is highly diverse when compared to the early successional stage, displaying many late-successional species. The richness is represented by 21 tree species distributed in 12 families, among them Anadenanthera colubrina (Vell.) Brenan, A. pyrifolium, C. pyramidale and Commiphora leptophloeos (Mart.) J.B. Gillett. The mean shoot biomass production for the late-successional stage area was 49.4 Mg ha−1 [Citation15,Citation28].
Collection and sampling of the target tree species
A concomitant presence in both succession stages was the main criterion for the selection of model species and was based on information by Cabral and collaborators [Citation15]. Two pioneer tree species were selected in this study: Aspidosperma pyrifolium (Apocynaceae) (), a native species with a massive presence in Brazilian tropical dry forests, such as Caatinga and Cerrado. These trees are deciduous and can grow up to 8 m, displaying straight trunks with smooth bark and alternate simple leaves [Citation29,Citation30] and Cenostigma pyramidale (Fabaceae) (), a semi-deciduous species endemic to the Caatinga. Individuals grow up from 4 to 6 m and display bipinnate leaves, light grey trunk, and high wood density [Citation31]. The sampling criteria were defined as trees with a diameter at breast height (DBH) > 7 cm, and at least 10 individuals/species in each collection area.
In order to obtain an estimation of the accumulated effect (i.e. damage) caused by herbivores during the leaf lifespan, the sampling was performed at the end of the rainy season, in April 2016 and 2017. We collected and measured leaves from the two species located in the early- and late- succession stage areas. Each collection area has 2,000 m2 and is fenced with barbed wire to avoid the presence of livestock.
Leaf herbivory
The herbivory percentage was obtained from a set of 20 leaves of each tree for both species. Sample collections were performed in two consecutive years, always at the end of the rainy season, guaranteeing the estimate of the damage accumulated during leaf’s lifespan. Leaves were scanned for damage measurement. We considered damage as the removal of the leaf blade caused by folivores (chewers and cutters leaves). We used ImageJ [Citation32] to quantify the total leaf area for each leaf, as well as the areas removed by the folivores. Each image was converted to a binary file (black and white) from which the herbivory percentage was obtained using the following formula: [(area lost to herbivory/total leaf area) * 100]. The herbivory percentage was subsequently analyzed individually for each tree.
Leaf traits
Ten leaves from trees that satisfied the sampling criteria for each species in both successional stages were collected in two consecutive years and used for leaf trait measurements. We used the same trees for measurements every year. Leaf thickness was obtained using a digital calliper at leaf’s widest section while avoiding the midrib, as described by Pérez-Harguindeguy et al. [Citation33]. Specific leaf area (SLA) was calculated using leaf area divided by leaf dry matter. The leaves were digitalized individually using a scanner, and each leaf area was obtained using ImageJ [Citation32]. Subsequently, the same leaves were oven-dried individually at 70°C for 72 hours and the respective weights were obtained using a precision scale (HR-200, E, Tokyo, Japan) [Citation34]. Leaf density was determined by the ratio between specific leaf mass and thickness [Citation35].
The nitrogen (N), phosphorus (P), and potassium (K) content in leaves were obtained using 250 mg of oven-dry and ground leaf mass. This dry matter underwent acid digestion (H2SO4) in a digestion block at 350°C for extraction. Total N content was quantified from titration of the digested plant extract with HCl after the addition of boric acid and a coloured indicator [Citation36]. Spectrophotometry was used for the analysis of P content (Spectrophotometer 600S, FEMTO, São Paulo, BR) following the methodology described by Murphy and Riley [Citation37]. The N:P ratio was also calculated. The content of K was derived by flame photometry (DM-62, Digimed, São Paulo, BR) using a standard solution of 5 ppm of K [Citation38].
We used 20 mg of dry leaf material for the determination of total phenolic compounds, boiling it in methanol 80% for extraction and further submitting it to a reaction with Folin-Ciocalteu 10% as described by Amorim et al. [Citation39]. Results were derived by interpolating samples’ absorbance against a standard curve, which was obtained projecting tannic acid (0.1 mg ml−1) in a spectrophotometer (Genesys 10S UVVIS, Thermo Scientific, Waltham, EUA) adjusted to a wavelength of 760 nm. The results from all measured traits were averaged individually for each sampled tree from both species.
Data analysis
The leaf trait variations in this study (specific leaf area, thickness, density, nutrient content, phenolic compounds, and herbivory rates) were analyzed using repeated measures MANOVA, with the succession stage set as an independent variable (). Averages were further compared using the Bonferroni test (5%). The analyses were carried out using R 2.15 [Citation40]. A principal component analysis (PCA) was performed for each tree species to identify which leaf traits were more responsive to successional stage, using software Fitopac 2.1 [Citation41].
Table 1. Multivariate analysis of variance (MANOVA) of functional leaf traits: specific leaf area (SLA), leaf density (DEN), leaf thickness (THICK), total phenolic compounds (PC), nutrient content (N, P, K, N:P), and leaf tissue lost to herbivory (HERB) in tropical dry forest (Santa Terezinha, Paraíba, Brazil)
Results
The average percentage of leaf loss due to herbivory in A. pyrifolium was 32% higher in the early-successional area compared to the late stage (P < 0.05, ). C. pyramidale trees also showed higher herbivory rates in the early stage (P < 0.05), with an increase of about 42% with respect to the values observed in the late stage (). The highest percentage of herbivory was exhibited by A. pyrifolium.
Figure 3. Leaf tissue lost to herbivory for Aspidosperma pyrifolium (a) and Cenostigma pyramidale (b) individuals from early and late succession stages of tropical dry forest over two consecutive years (Santa Terezinha, Paraíba, Brazil). Bars ± standard error followed by similar letters indicate a non-significant difference according to the Bonferroni test (5%), n = 10
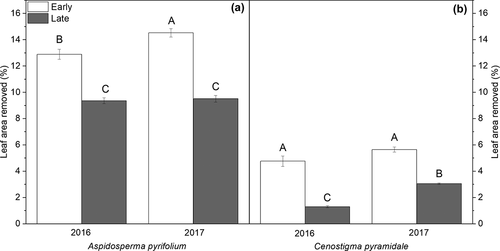
Leaves from A. pyrifolium and C. pyramidale individuals in the early succession stage had lower levels of carbon-based defenses, as observed through sclerophylly results (i.e. contrary to SLA). SLA was significantly higher in the early stage for both species and in the two sampled years. This difference between the stages represented a minimum increase of 25% in the SLA in the early area (P < 0.05, ). The highest value of density between species was observed in C. pyramidale in 2016 and 2017. However, A. pyrifolium and C. pyramidale trees from the late succession stage showed higher leaf density values (in general, this increase was over 80%) in the two sampled years (P < 0.05; ). Leaf thickness showed the same trend, with values up to 42% higher for individuals from both species in the late succession stage (P < 0.05; ). In turn, A. pyrifolium leaves had higher values of thickness. The species A. pyrifolium and C. pyramidale showed a similar behavior concerning the concentration of phenolic compounds, indicating a significant increase from early to late succession stages in the two sampling years (). Total phenolic compounds in A. pyrifolium and C. pyramidale had a difference of approximately 1.4 and 1.7 times between the areas, respectively.
Figure 4. Leaf traits for Aspidosperma pyrifolium and Cenostigma pyramidale individuals from early and late succession stages of tropical dry forest over two consecutive years (Santa Terezinha, Paraíba, Brazil). (a; b) specific leaf area; (c; d) leaf density; (e; f) leaf thickness; (g; h) phenolic compounds. Bars ± standard error followed by similar letters indicate a non-significant difference according to the Bonferroni test (5%), n = 10
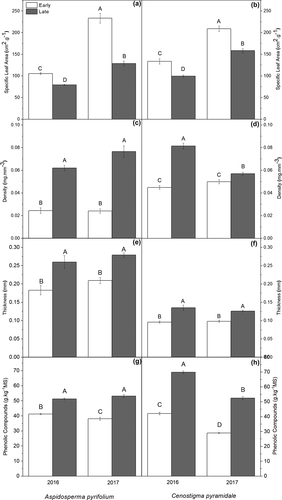
Leaf N and P contents decreased from the early to the late succession stage for both species (P < 0.05, ). This was observed in both years of sampling. Leaf K content was higher for A. pyrifolium individuals from the early succession stage (P < 0.05, ). On the other hand, C. pyramidale trees did not show any differences in K content (P > 0.05, ). We observed an increase in the N:P ratio for A. pyrifolium and C. pyramidale showed for both succession stages (P < 0.05, ).
Table 2. Leaf nitrogen, phosphorus and potassium content, and N:P ratio for Aspidosperma pyrifolium and Cenostigma pyramidale individuals from early and late succession stages of tropical dry forest over two consecutive years (Santa Terezinha, Paraíba, Brazil). Averages ± standard error followed followed by similar letters indicate a non-significant difference according to the Bonferroni test (5%), n = 10
The PCA shows that year of collection and successional stage are the main drivers of the identified groups. In A. pyrifolium, the analysis showed a greater relationship between the successional stage to axis 1 (50.43%), while axis 2 was more related to the annual variation of attributes (32.91%). Leaf traits such as the total phenolic content, thickness, and density, with higher values in the late area, explained more strongly the differences presented between the areas in axis 1. In parallel, axis 2 was explained by the increase in the mobilization of nutrients, especially N and SLA (). For C. pyramidale, the successional stages also formed evident groups and more related to axis 1 of the PCA (63.68%), especially due to characteristics such as density, thickness and phenolic content. The variation in axis 2 was explained by 21.90% and also due to these interannual differences ().
Figure 5. Principal component analysis of the data collected from Aspidosperma pyrifolium (a) and Cenostigma pyramidale (b) in the tropical dry forest (Santa Terezinha, Paraíba, Brazil). Early stage (circle), late stage (square). The size of the symbols represents leaf tissue lost to herbivory. Specific leaf area (SLA), leaf density (DEN), leaf thickness (THICK), total phenolic compounds (PC) and nutrient content (N, P, K, N:P)
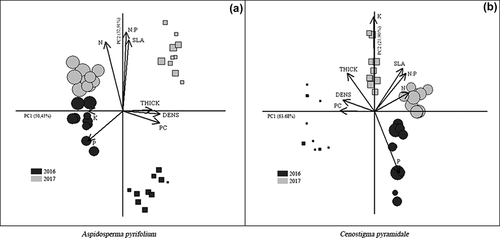
Discussion
The percentage of plant tissue lost to herbivory found for the species in this study is within the average values observed for tropical dry forests [Citation42]. For instance, Fonseca et al. [Citation2] found leaf herbivory rates between 2.5% and 4.4% in a tropical dry forest in Minas Gerais, Brazil. Aquino et al. [Citation43] found leaf damage rates between 8.8% and 12% for Aspidosperma pyrifolium. Variations in herbivory percentages between species reflect the use of different strategies, such as physical and chemical defenses by plants, their characteristics, present environmental conditions, or even its tolerance to herbivory [Citation18,Citation44,Citation45]. In our study, we observed a higher percentage of herbivory in A. pyrifolium. In general, the species showed a lower leaf density, which may have contributed to this difference. In addition, deciduous species have less investment in other defenses types such as chemical compounds that demand higher metabolic costs (i.e. tannins and alkaloids), generating herbivory percentages up to 2.8 times higher than those reported in perennial species for dry forests [Citation46]. Our results corroborate this premise. Dourado et al. [Citation47] studying herbivory in Caatinga also reported more than twice the leaf loss in deciduous species and with less leaf hardness, compared to perennial species. The herbivory increment between the analyzed years for the two species can also be justified by the increase in the leaf traits, such as SLA and nitrogen content and the decrease in phenolic compounds. All of these are associated with the plant’s palatability [Citation18,Citation33,Citation43]
In general, the distinct responses shown by the measured leaf traits in the different stages (early and late) for both tree species corroborate predictions based on the resource availability hypothesis [Citation4,Citation7]. This hypothesis postulates that there is a higher investment in growth than in the production of defenses, as the costs of building leaves and replacing the tissue lost to herbivores are lower due to nutrient quantities and high availability of light in the early succession stages. Similar responses are also documented in other studies in tropical dry forests [Citation2,Citation48,Citation49]. The area lost to herbivores in leaves for both species from the late stage showed a decrease of over 40% when compared to individuals from the early stage ().
SLA increments in the early stages for both species are directly linked to plants photosynthetic capacity and efficiency in resource usage – like nutrients and light – lowering the overall cost of replacing tissues lost to herbivores [Citation3,Citation33,Citation50]. These leaves are more vulnerable to herbivory, as they usually are tenderer and less resistant [Citation48]. Increases in thickness and density in the late stage may have played a role in reducing herbivory’s incidence [Citation51], but also elevate leaves’ production costs and often their lifespan time [Citation22,Citation44,Citation52]. The leaf density is also considered a type of initial defense against many kinds of generalist herbivores, rendering harder leaves, with higher biomechanical resistance and reducing the palatability and/or tissue nutrition content [Citation3,Citation44,Citation53]. In the early stage, an increase in nutrient content and a significant decrease in the content of phenolic compounds favor higher palatability and represent a higher metabolic activity when compared to leaves with opposing traits [Citation3,Citation10,Citation53].
Individuals from the early stage adopt a strategy of low-cost investment (lower sclerophylly, higher nutrient content and less phenolic compounds), while the late-successional stage individuals allocate resources in long-lived tissue (higher sclerophylly, lower nutrient content) with higher levels of phenolic compounds, that are frequently related to higher protection against herbivores [Citation10,Citation44]. These results for both species suggest the same trend in leaf trait changes, possibly influenced by environmental differences between stages, for example, the light interception, the amount of soil nutrients, and water availability. Leaf traits can vary due to numerous environmental pressures, such as changes in SLA due to water conservation, but these adaptations can also favor the plant’s defenses against herbivores [Citation44,Citation54,Citation55]. Furthermore, while leaf traits are prevalent over the inflicted herbivory percentage [Citation56], higher levels of herbivory pressure could also be playing an essential role to elevate the herbivory rates observed in the early-successional stages [Citation57]. In the same area of the current study, Vieira [Citation58] found a higher abundance of arthropods in the early stage during wet and dry seasons when compared to the late-successional stage, and Silva et al. [Citation59] found higher levels of richness and abundance of herbivore insects in early succession stage in tropical dry forests.
The tendency of producing simpler and lower cost tissue is corroborated by the higher concentrations of leaf N and P in trees from the early stage [Citation4,Citation7]. The increment in these nutrients also indicates a nitrogen mobilization for protein synthesis, in particular for proteins linked to photosynthesis as Rubisco, with the energy used to higher production of leaf biomass [Citation6,Citation60,Citation61]. This perspective is correct if we analyze the increase in SLA together with the increase in N content during the years of sampling in this study. On the other hand, the lower content of N and P found in the plant species from the late succession stage might indicate a shift of energy use to metabolize phenolic compounds through a limitation in protein synthesis [Citation61,Citation62]. In fact, the increase observed in N content for species in the late stage in 2017 was followed by a decrease in the phenolic compounds, especially in C. pyramidale. The N:P ratio for both tree species also corroborates these data. These features reinforce the behavior of producing leaves that are costly and have more defense compounds, and they were really efficient, given the lower values of herbivory found in the late stage.
It is well known that the content of secondary metabolite, nutrients, and physical traits are central to how the herbivore perceives the quality of the plant [Citation8,Citation63,Citation64]. For example, the negative relationship between phenolic compounds content and leaf herbivory has been pointed out by many authors, including in tropical dry forests, and indicates the important role these substances play on providing the plant with effective defensive mechanisms [Citation7,Citation18,Citation53,Citation65]. The higher content of N in the leaf seems to be a fundamental aspect for leaf consumption. This relationship has been observed in many ecosystems, including tropical dry forests [Citation58,Citation66–68]. The Principal Component Analysis showed the formation of groups in relation to successional stages; thus, the herbivory rate indicates to be higher in environments where the investment in defensive traits was lower and the nutritional quality of the leaves was higher [Citation2,Citation44].
Conclusions
Our results show that A. pyrifolium and C. pyramidale showed different strategies related to herbivore defense depending on successional stage. On the one hand, there is the production of low-cost leaves in the early stage that resulted in higher percentages of herbivory. On the other hand, there is allocation of resources in tissues with a higher life span, in addition to an increase in defense metabolites, which require more energy from the plant, but result in lower percentages of herbivory. In summary, we observed that plant defenses are strongly linked to the leaf economic spectrum. That is, chemical (nutrient and phenolic content) and structural (SLA, density, and thickness) properties in leaves determine how plants may adopt a growth-pattern that runs from quick to slow return on investments of nutrients and dry mass in leaves.
The understanding of how plants allocate resources according to the needs imposed by herbivory, and also by the abiotic environment, can contribute to determine which species are a priority for assisted restoration programs in degraded areas.
Acknowledgments
We gratefully acknowledge for Instituto Fazenda Tamanduá for logistical support and Mr Pierre Landolt for allowing us to develop research at Fazenda Tamanduá. Claudio Magalhães to manuscript English first review. Seu Jorge to field assistant. The authors thank FACEPE and Capes (financial code 001) for the research grant. The authors acknowledge the Brazilian Conselho Nacional de Desenvolvimento Científico e Tecnológico (CNPq –PQ 309965/2016-0), for scientific productivity fellowship. We would also like to thank the two anonymous reviewers for commenting on manuscript.
Disclosure statement
No potential conflict of interest was reported by the author(s).
Additional information
Funding
References
- Endara MJ, Coley PD. The resource availability hypoth- 520 esis revisited: a meta-analysis. 2011). Funct Ecol. 2011;25(2):389–398.
- Fonseca MB, Silva JO, Falcão LA, et al. Leaf damage and functional traits along a successional gradient in Brazilian tropical dry forests. Plant Ecol. 2018;219(4):403–415. .
- Chauvin KM, Asner GP, Martin RE, et al. Decoupled dimensions of leaf economic and anti-herbivore defense strategies in a tropical canopy tree community. Oecologia. 2018;186(3):765–782. .
- Coley PD, Bryant JP, Chapin FS. Resource availability and plant anti-herbivore defense. Science. 1985;230(4728):895–899.
- Lande R. Adaptation to an extraordinary environment by evolution of phenotypic plasticity and genetic assimilation. J Evol Biol. 2009;22(7):1435–1446.
- Falcão HM, Medeiros CD, Silva BL, et al. Phenotypic plasticity and ecophysiological strategies in a tropical dry forest chronosequence: a study case with Poincianella pyramidalis. For Ecol Manage. 2015;340:62–69.
- Poorter L, Van de Plassche M, Willems S, et al. Leaf traits and herbivory rates of tropical tree species differing in successional status. Plant Biol. 2004;6(6):746–754. .
- Defossez E, Pellissier L, Rasmann S. The unfolding of plant growth form‐defence syndromes along elevation gradients. Ecol Lett. 2018;21(5):609–618.
- Wright IJ, Reich PB, Westoby M, et al. The worldwide leaf economics spectrum. Nature. 2004;428(6985):821–827. .
- Westoby M, Wright I. Land-plant ecology on the basis of functional traits. Trends Ecol Evol. 2006;21(5):261–268.
- Poorter L, Bongers F. Leaf traits are good predictors of plant performance across rain forest species. Ecology. 2006;87(7):1733–1743.
- Laughlin DC. The intrinsic dimensionality of plant traits and its relevance to community assembly. J Ecol. 2013;102(1):186–193.
- Chazdon RL, Guariguata MR. Natural regeneration as a tool for large-scale forest restoration in tropics: prospects and challenges. Biotropica. 2016;48(6):716–730.
- Martínez-Garza C, Pena V, Ricker M, et al. Restoring tropical biodiversity: leaf traits predict growth and survival of late-successional trees in early-successional environments. For Ecol Manage. 2005;217(2–3):365–379. .
- Gal C, Evsb S, JS A-C. Spatial structure and aboveground biomass in different caatinga succession stages, in Santa Terezinha, Paraiba. Revista Brasileira De Geografia Física. 2013;6:566–574.
- Violle C, Navas ML, Vile D, et al. Let the concept of trait be functional! Oikos. 2007;116(5):882–892. .
- Karban R, Shiojiri K, Ishizaki S. Plant communication–why should plants emit volatile cues? J Plant Interact. 2011;6(2–3):81–84.
- Fürstenberg-Hägg J, Zagrobelny M, Bak S. Plant defense against insect herbivores. Int J Mol Sci. 2013;14:10242–10297.
- Vendramini F, Díaz S, Gurvich DE, et al. Leaf traits as indicators of resource-use strategy in flora with succulent species. New Phytol. 2002;154(1):147–157. .
- Wright IJ, Cannon K. Relationships between leaf lifespan and structural defences in a low-nutrient, sclerophyll flora. Funct Ecol. 2001;15(3):351–359.
- Meyer S, Cerovic ZG, Goulas Y, et al. Relationships between optically assessed polyphenols and chlorophyll contents, and leaf mass per area ratio in woody plants: a signature of the carbon-nitrogen balance within leaves? Plant Cell Environ. 2006;29(7):1338–1348. .
- Jg H, Montserrat-Martí G, Charles M, et al. Is leaf dry matter content a better predictor of soil fertility than specific leaf area?. Ann Bot. 2011;108(7):1337–1345.
- Diaz S, Hodgson JG, Thompson K, et al. The plant traits that drive ecosystems: evidence from three continents. J Veg Sci. 2004;15(3):295–304. .
- Katabuchi M, Kurokawa H, Davies SJ, et al. Soil resource availability shapes community trait structure in a species-rich dipterocarp forest. J Ecol. 2012;100(3):643–651. .
- Schuldt A, Bruelheide H, Durka W, et al. Plant traits affecting herbivory on tree recruits in highly diverse subtropical forests. Ecol Lett. 2012;15(7):732–739. .
- Empresa Brasileira de Pesquisa Agropecuária. Centro Nacional de Pesquisa de Solos. Sistema brasileiro de classificação de solos. 2 ª ed Rio de Janeiro (RJ): Embrapa solos; 2006.
- Lyra-Neves RM, Telino-Júnior WR. Aves da Fazenda Tamanduá. 1ª ed. Vinhedo: Avis Brasilis; 2010.
- Freitas ADS, Sampaio EVSB, Silva BLR, et al. How much nitrogen is fixed by biological symbiosis in tropical dry forests? Nutr Cycl Agroecosystems. 2012;2(2–3):181–192. .
- Hermuche PM, Felfili JM. Relação entre NDVI e florística em fragmentos de floresta estacional decidual no Vale do Paranã, Goiás. Cienc Florest. 2011;21(1):41–52.
- Maia-Silva C, Silva CID, Hrncir M, et al. Guia de plantas visitadas por abelhas da caatinga. 1ª ed. Fortaleza: Fundação Brasil Cidadão; 2012.
- Castello ACD, Pereira ASS, Simões AO, et al. Aspidosperma in Flora do Brasil em construção. 2019 - [ Cited 2019 Sept 19]. Available in: http://floradobrasil.jbrj.gov.br/reflora/floradobrasil/FB15551.
- WS R. ImageJ. Bethesda, Maryland (USA): US National Institutes of Health; 2010.
- Perez-Harguindeguy N, Diaz S, Garnier E, et al. New handbook for standardised measurement of plant functional traits worldwide. Aust J Bot. 2013;61:167–234.
- Garnier E, Shipley B, Roumet C, et al. A standardized protocol for the determination of specific leaf area and leaf dry matter content. Funct Ecol. 2001;15(5):688–695.
- Witkowski ETF, Lamont BB. Leaf specific mass confounds leaf density and thickness. Oecologia. 1991;88(4):486–493.
- Thomas RL, Sheard RW, Moyer JR. Comparison of conventional and automated procedures for Nitrogen, Phosphorus, and Potassium analysis of plant material using a single digestion 1. Agron J. 1967;59(3):240–247.
- Murphy J, Riley JP. A modified single solution method for the determination of phosphate in natural waters. Anal Chim Acta. 1962;27:31–36.
- Silva CS, Silva-Filho FC, Santos AD, et al. Manual das análises químicas de solos, plantas e fertilizantes. Brasilia (DF): Embrapa Informação Tecnológica; 2009.
- Amorim ELC, Nascimento JE, Monteiro JM, et al. A simple and accurate procedure for determination of tannin and flavonoid levels and some applications in Ethnobotany and Ethnopharmacology. Funct Ecosyst Comm. 2008;2:88–94.
- R Development Core Team. R: a language and environment for statistical computing. Vienna, Austria: R Foundation for Statistical Computing; 2015. Accessed September 2019. http://www.R-project.org
- Fitopac SGJ. Versão 2.1. São Paulo (SP): departamento de Botânica, Universidade Estadual de Campinas; 2010.
- Filip V, Dirzo R, Maass JM, et al. Within-and among-year variation in the levels of herbivory on the foliage of trees from a Mexican tropical deciduous forest. Biotropica. 1995;27(1):78–86. .
- Aquino RE, Falcão HM, Almeida-Cortez JS. Variations in the concentrations of phenolic compounds and herbivory rates in Aspidosperma pyrifolium Mart. in disturbed Caatinga’s áreas. J Environ Anal Prog. 2017;2(1):61–71.
- González-Esquivel JG, Cuevas-Reyes P, González-Rodríguez A, et al. Functional attributes of two Croton species in different successional stages of tropical dry forest: effects on herbivory and fluctuating asymmetry patterns. Trop Ecol. 2019;60(2):1–14. .
- Kost C, Tremmel M, Wirth R. Do leaf cutting ants cut undetected? Testing the effect of Ant-Induced plant defences on foraging decisions in Atta colombica. PLoS One. 2011;6(7):1–8.
- Dirzo R, Boege K. Patterns of herbivory and defence in tropical dry and rain forest. In: Carson WP, Schnitzer SA, editors. Tropical forest community ecology. Chichester: Wiley-Blackwell; 2008. p. 63–78.
- Dourado ACP, Sa-Neto RJ, Gualberto SA, et al. Herbivoria e características foliares em seis espécies de plantas da Caatinga do nordeste brasileiro. R Bras Bioci. 2016;14(3):145–151.
- Lohbeck M, Poorter L, Lebrija-Trejos E, et al. Successional changes in functional composition contrast for dry and wet tropical forest. Ecology. 2013;94(6):1211–1216. .
- Derroire G, Powers JS, Hulshof CM, et al. Contrasting patterns of leaf trait variation among and within species during tropical dry forest succession in Costa Rica. Sci Rep. 2018;8(1):285. .
- Shipley B, Vile D, Garnier E, et al. Functional linkages between leaf traits and net photosynthetic rate: reconciling empirical and mechanistic models. Funct Ecol. 2005;19(4):602–615.
- Firn J, McGree JM, Harvey E, et al. Leaf nutrients, not specific leaf area, are consistent indicators of elevated nutrient inputs. Nat Ecol Evol. 2019;3(3):400–406. .
- Kitajima K, Llorens AM, Stefanescu C. How cellulose-based leaf toughness and lamina density contribute to long leaf lifespans of shade-tolerant species. New Phytol. 2012;195(3):640–652.
- Coley PD, Endara MJ, Kursar TA. Consequences of interspecific variation in defenses and herbivore host choice for the ecology and evolution of Inga, a speciose rainforest tree. Oecologia. 2018;187(2):361–376.
- Garibaldi LA, Kitzberger T, Chaneton EJ. Environmental and genetic control of insect abundance and herbivory along a forest elevational gradient. Oecologia. 2011;167(1):117–129.
- Alvarez‐Añorve MY, Quesada M, Sánchez‐Azofeifa GA, et al. Functional regeneration and spectral reflectance of trees during succession in a highly diverse tropical dry forest ecosystem. Am J Bot. 2012;99(5):816–826. .
- Stiling P, Moon DC. Quality or quantity: the direct and indirect effects of host plants on herbivores and their natural enemies. Oecologia. 2005;142(3):413–420.
- Neves FS, Silva JO, Espírito‐Santo MM, et al. Insect herbivores and leaf damage along successional and vertical gradients in a tropical dry forest. Biotropica. 2014;46(1):14–24. .
- Vieira AL. 2018. Effect of a dry forest regeneration on the diversity of litter arthropods [master’s thesis]. Recife (PE): Federal Rural University of Pernambuco.
- Silva JO, Espírito-Santo MM, Melo GA. Herbivory on Handroanthus ochraceus (Bignoniaceae) along a successional gradient in a tropical dry forest. Arthropod Plant Interact. 2012;6(1):45–57.
- Reich PB, Oleksyn J, Wright IJ. Leaf phosphorus influences the photosynthesis–nitrogen relation: a cross-biome analysis of 314 species. Oecologia. 2009;160(2):207–212.
- Zhu SD, Song JJ, Li RH, et al. Plant hydraulics and photosynthesis of woody species from different successional stages of subtropical forests. Plant Cell Environ. 2013;36(4):879–891. .
- Cartelat A, Cerovic ZG, Goulas Y, et al. Optically assessed contents of leaf polyphenolics and chlorophyll as indicators of nitrogen deficiency in wheat (Triticum aestivum L.). Field Crops Res. 2005;91(1):5–49. .
- Dyer LA, Singer MS, Lill JT, et al. Host specificity of Lepidoptera in tropical and temperate forests. Nature. 2007;448(7154):96–699. .
- Massad TJ, Dyer LA. A meta-analysis of the effects of global environmental change on plant-herbivore interactions. Arthropod Plant Interact. 2010;4(3):181–188.
- Aboshi T, Ishiguri S, Shiono Y, et al. Flavonoid glycosides in Malabar spinach Basella alba inhibit the growth of Spodoptera litura larvae. Biosci Biotechnol Biochem. 2018;82(1):9–14. .
- Meloni F, Lopes NP, Varanda EM. The relationship between leaf nitrogen, nitrogen metabolites and herbivory in two species of Nyctaginaceae from the Brazilian Cerrado. Environ Exp Bot. 2012;75:268–276.
- Silva JO, Espírito-Santo MM, Morais HC. Leaf traits and herbivory on deciduous and evergreen trees in a tropical dry forest. Basic Appl Ecol. 2015;16(3):210–219.
- Njovu HK, Peters MK, Schellenberger CD, et al. Leaf traits mediate changes in invertebrate herbivory along broad environmental gradients on Mt. Kilimanjaro, Tanzania. J Anim Ecol. 2019;88(11):1777–1788.