Abstract
The authors carried out 1.5 T MRI examination without removing the CI magnet in two children with a cochlear implant (CI). In the first child, a conventional MRI scanning technique was used, which produced an image that was not clinically useful due to artifact size. He/she was scanned again using the ‘propeller technique’. This scan had a reduced artifact and was clinically useful. The second child was scanned using the propeller technique while asleep. The image was clinically useful. The authors conclude that the propeller technique is safe and of benefit to clinical diagnosis because it enables clinically useful images to be generated in a 1.5 T MRI without necessitating CI magnet removal and reimplantation surgeries.
1. Introduction
A cochlear implant (CI) enables users to perceive sound by converting acoustic sound signals into electrical signals and stimulating the auditory nerve fibers inside the cochlea. Two important parts of a CI are the (externally worn) audio processor and the (internal) implant, which are connected via magnets. For stable transcutaneous signal transmission. it is important that the externally worn audio processor coil is always located exactly over the implant. If a CI user needs to have Magnetic Resonance Imaging (MRI), this can be problematic because magnetic forces in the MRI chamber drag on the implant magnet which can cause it to move. This is potentially dangerous for patients and for MRI machines. Not only can the implant magnet demagnetize (thus making it useless), it also generates an imaging artifact which blurs anatomical structures in the vicinity of the implant (thus making the image potentially clinically useless). Therefore, surgical removal of the implant magnet prior to an MRI scan is often considered (e.g. [Citation1]). However, there are several reasons against pre-MRI implant magnet removal:
Removing the internal magnet necessitates surgery. This typically requires general anesthesia for children [Citation1,Citation2]. With adults, local anesthesia is also possible [Citation3]. If repeated MRI scans over several days are necessary, the implant magnet is replaced by a non-magnetic spacer. When the MRI(s) have been completed, another surgery is necessary to replace the spacer with the magnet. Thus, having an MRI requires two general anesthesia operations and repeated scalp incisions, which are invasive. After these surgical interventions, the patient cannot wear a processor coil and thus temporarily loses their ability to hear and therefore, to some extent, communicate [Citation1].
Forces applied to the implant during magnet removal may damage the implant or cause it to displace. If displacement occurs, revision surgery is necessary. Furthermore, as with every surgery, there is also the risk of infection [Citation4–7].
Surgical removal of the implant magnet needs planning and coordination between a hospital’s ENT department and radiology department, and this requires time. Precious time elapses before an MRI can be performed, and this may be a strong counterargument for urgent MRI scans.
Finally, there are CI models available which are safe for MRI scanning under certain conditions but do not have an implant magnet which can be surgically removed.
Thus, there are several reasons for a ‘non-invasive MRI examination’ for CI users. To meet this need, the authors carried out 1.5 T MRI examination without removing the CI magnet. This was done in accordance with the CI manufacturer’s instruction manual [Citation8] and the consensus of Chinese experts on the safety management of MRI imaging [Citation9]. Considering the area to be inspected was only around the implant and the artifact range may affect diagnostic possibility, a new technique called the ‘propeller technique’ was used [Citation10,Citation11]. This study compares the range difference of the artifacts generated by MRI using the propeller technique with those generated via a conventional technique.
2. Methods
2.1. Patients
Two patients participated in this study:
Patient A was 11 years old, had congenital bilateral sensorineural hearing loss, and had been a unilateral CI user for 8 years (right ear, SONATA Ti100, MED-EL). His/her motor coordination had been deteriorating for 2 months, so an MRI was performed to determine the cause.
Patient B was two years old, had congenital bilateral sensorineural hearing loss, and had been a bilateral CI user for 4 months (both ears, CONCERTO Mi1000, MED-EL). He/she was not developing age-appropriate hearing and speech, so an MRI was performed to determine the cause.
2.2. Process
Both patients underwent a 1.5 T MRI. Patient 1, who was awake, underwent two MRI scans (both HDxt 1.5 T, General Electric, Boston, MA, USA): firstly, with the conventional scanning technique and secondly with the propeller technique. Patient 2 underwent one MRI scan (Signa Explorer 1.5 T, General Electric), in which the propeller technique was used because of his/her young age and to get the best possible images while he/she was sleeping.
2.2.1. Conventional MRI technique
Conventional sequences consist of Fast Spin Echo (FSE), Inversion Recovery FSE (IR FSE), and DW-EPI. To be specific, T2-weighted imaging is based on the FSE sequence (T2WI FSE), T1 Flair and T2 Flair are based on the IR FSE sequence, and Diffusion-weighted imaging is based on the DW-EPI sequence (DWI). All of them, but especially DWI, are sensitive to metal and magnet artifacts, which cause a great loss of imaging signals.
Detailed parameters:
T2 FSE: TR 2000-3000ms, TE 102ms, Bandwidth 31.25kHz, FOV 24cm*24cm, 5.0thk/1.0sp, Matrix 320*224/1NEX
T1 Flair: TR 1750ms, TE 24ms, TI 750ms, Bandwidth 25Hz, FOV 24cm*24cm, 5.0thk/1.0sp, Matrix 320*224/2NEX
T2 Flair: TR 8400ms, TE 145ms, TI 2200ms, Bandwidth 25Hz, FOV 24cm*24cm, 5.0thk/1.0sp, Matrix 256*224/2NEX + ZIP512
DWI EPI: TR 4000ms, TE Minimum, FOV 24cm*24cm, 5.0thk/1.0sp, Matrix 128*128, b = 1000sec/mm2, NEX = 2
2.2.2. Propeller technique
The propeller technique is based on the spiral spokes signal acquisition mode of FSE.
This MRI technique uses a set of radially directed strips to sample the k-space in a rotating fashion. This leads to an oversampling of the center of the k-space, which results in a high signal amplitude and a high image contrast. Oversampling also provides a redundancy of information thereby allowing the detection and correction of movement artifacts (Figure ).
Figure 1. Propeller sequences, the echoes fulfill the k-space in a screw propeller-like way, providing possibility of correcting motion and metal-magnetic artifacts.
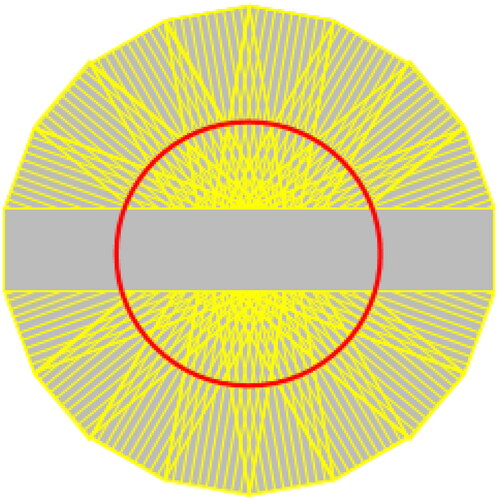
Detailed parameters:
Propeller T2: TR 3000ms, TE 82ms, Bandwidth 50Hz, FOV 24cm*24cm, 5.0thk/1.0sp, Matrix 320*320/1.5NEX
Propeller DWI: DW/Propeller, TR 3500ms, TE 84ms, Bandwidth 83Hz, FOV 24cm*24cm, 5.0thk/1.0sp, Matrix 128*128/1.5NEX, b Value = 1000sec/mm2, Fat Saturation Yes
2.3. Safety
The safety of each MRI technique was assessed via patient or clinical reports of pain during or up to 3 days after the conclusion of the scan(s).
3. Results
Patient A: No demagnetization or pole reversal occurred in the CIs. He/She reported no discomfort or pain and the CI processor could be used immediately after examination.
The scan using the conventional technique resulted in an image that was not clinically usable because the artifact blocked the image of the lesion (See Figure ). To create a usable image, a second MRI was conducted, this time using the propeller technique. The images this scan created had a smaller artifact. In axial scans the maximum diameter of the signal void area reduced by about 20%, and this made the images clinically usable (see Figure ). Patient B: No demagnetization or pole reversal occurred in the CIs. He/She reported no discomfort or pain. CI processors could be used immediately after examination.
Figure 2. A: Conventional T2 sequence: the magnet artifacts covered up most of the lesion areas. B: Propeller T2 sequence: the magnet artifacts decreased significantly. The lesions presented high signal in Propeller T2 sequence C: Conventional DWI sequence: Because the DWI is an EPI sequence, it is very sensitive to the local magnetic field inhomogeneity caused by metal. This results in severe deformation of conventional DWI, heavy metal artifacts, and completely blocks the image of the lesion. D: Propeller DWI sequence reducing metal artifacts. The image is basically without deformation, although there is interference of metal artifacts in cochlea, it can still be observed that the lesions presented high signal.
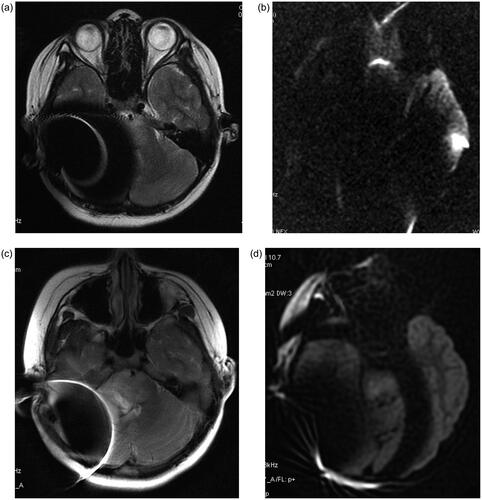
The images were clinically usable although they did contain an artifact.
A comparison of the conventional MRI and propeller scanning techniques within this patient was not obtained. However, compared to the images obtained from Patient A with a conventional technique, it is obvious that the range of artifacts was much smaller with propeller technique and that the lesion could be clearly seen (Figure ).
4. Discussion
Conducting an MRI on a patient with a CI always requires special attention and care. Many potential hazards arise from interactions between the implant magnet and the powerful magnetic fields present in an MRI scanner. These include the risk of magnet dislocation, with potential of pain and discomfort; tissue injury; and implant damage. With modern CIs, safe MRI scanning is possible without explanting the implant magnet if the manufacturer’s MRI safety guidelines are followed.
For MRI scans of the brain, however, the artifact generated by the magnet can negatively affect the image quality and render some scans clinically useless. If it is possible to keep the imaging artifact small enough, then surgical magnet removal with all its burden and risks can be avoided. Avoiding surgical magnet removal is advantageous, regardless of if the implant magnet is removable or not.
While a reduced artifact is a benefit for CI users of all ages, it is even more important in children for two reasons. Firstly, they usually have smaller heads than adults and therefore the artifact typically covers a larger anatomical region, thus the effect of a reduced artifact is even higher. Secondly, an MRI without surgical intervention allows the patient to resume using the CI immediately after the MRI. This could be advantageous for CI users who are developing their language skills because it prevents the extension of the time of little to no auditory input.
Trying to keep the susceptibility artifact of a CI as small as possible has been a goal since MRI scans of the head have been done on people with a CI. Selecting sequences which are more robust against magnetic field inhomogeneities should be selected (e.g. using spin echo sequences instead of gradient echo sequences). Adjusting sequence parameters can also help (e.g. change repetition time TR). The most benefit can be achieved when using metal artifact reduction sequences [Citation12,Citation13]. Such techniques also can successfully reduce the imaging artifacts active bone-conduction implants which have even larger implant magnets [Citation14,Citation15].
When comparing the images obtained with propeller sequences to those with conventional sequences from Patient A, it is clear that using the propeller technique can significantly reduce the range of artifacts caused by CIs. Also the images of the bilaterally implanted two-year-old Patient B obtained with propeller sequences show consistently small artifacts compared to those obtained using a conventional technique in the 11-yearold Patient A. Images obtained with the propeller technique for both patients still had a susceptibility artifact but were of diagnostic value. This is despite of the fact that both patients were children and that Patient B had bilateral CIs.
One possible reason for having smaller artifact images with the propeller technique could be different scanning parameters which influence susceptibility artifacts (e.g. slice thickness or receiver bandwidth) for the two acquisition techniques. Another reason could be due to the shape of the susceptibility artifact: it is typically larger in the phase coding axis than in the frequency coding direction. With a conventional technique this results in a certain artifact for a given slice orientation. With the propeller technique the orientation of the readout gradient changes permanently, and it can generally lead to a smaller artifact than is obtained when using a conventional technique. As scans obtained via the propeller technique do not have less diagnostic value than those obtained via conventional techniques, we can recommend using it for head scans of CI users, if possible.
As shown here, using the propeller technique can substantially reduce CI-related artifacts. This, however, does not necessarily mean that the propeller technique shows a significantly reduced range of artifacts for all types of sequences, for example, susceptibility weighted images (SWI) (which are routinely used in neuroimaging and which use a flow-compensated, long echo, 3 D gradient echo pulse sequence to acquire images) are much too susceptible to local field disturbances of a CI. Therefore, SWI, even with 3 D Short-Axis Propeller (SAP)-EPI, may likely not have any diagnostic value with CI users. Further limitations of the propeller technique include that it has an increased image acquisition time due to the strong oversampling around the k-space center; currently, it is only available in 2 D imaging; and it has limited robustness against through-plane motions.
There is a ‘smoothing effect’ on the images of the T2 propeller, meaning the borders of the anatomical structures in the brain melt slightly together. This makes it difficult to see subtle signal changes e.g. in the basal ganglia and in the gray white matter junction.
Whether the MRI artifact could be sufficiently reduced to allow for a diagnosis must be evaluated case by case. The artifact may still affect the region of interest. There may be situations when it would be advisable to extract the removable implant magnet, which can be performed without having to remove the electrode array from the cochlea. However, in CIs which do not have a removeable magnet, doing an MRI would necessitate the compete removal of the CI with electrode array, followed by reimplantation. Performing repeated explantations/implantations carries the potential chance of perioperative damage to inner ear structures and therefore an increased risk of loss of residual hearing and/or worsening of speech understanding. Also, the time until an implant user can hear again with their new implant is longer than if the magnet was only temporarily taken out. In such cases, accepting some degree of artifact may be preferable.
In summary, this case report shows the possible benefit of reducing susceptibility artifacts arising from an implant magnet when using the propeller technique instead of a conventional technique. Although the propeller technique was originally developed to reduce motion artifacts, it can also reduce susceptibility artifacts. The propeller technique is worth further study to solve the problem of artifacts around CIs which affect the clinical diagnoses for cerebral diseases. Further study is especially relevant because new CIs enable MRI scans to be conducted with the magnet in situ.
Informed consent
Authors confirm that consent was obtained from the patients for this study.
Acknowledgments
The authors thank Michael Todd (MED-EL) who provided English language help on a version of this manuscript and Martin Zimmerling for his contribution.
Disclosure statement
Author Zhi Shu is, as noted on the title page, a MED-EL employee. Otherwise the authors report no conflicts of interest.
References
- Helbig S, Stöver T, Burck I, et al. Cranial MRI in a young child with cochlear implants after bilateral magnet removal. Int J Pediatr Otorhinolaryngol. 2017;103:1–4.
- Zhen E, Kuthubutheen J, Misso D, et al. 3 Tesla MRI brain scanning under general anaesthesia in a paediatric 3 tesla-compatible cochlear implant recipient, first reported case: clinical considerations and implications for future practice. Int J Pediatr Otorhinolaryngol. 2020;133:110015.
- Wieser S, Igerc I, Hausegger K, et al. Worldwide 1st MED-EL Mi1200 SYNCHRONY cochlear implant magnet removal for MRI image artifact reduction. Otolaryngology Case Reports. 2018;9:41–44.
- Leinung M, Loth A, Gröger M, et al. Cochlear implant magnet dislocation after MRI: surgical management and outcome. Eur Arch Otorhinolaryngol. 2020;277(5):1297–1304.
- Hassepass F, Stabenau V, Maier W, et al. Revision surgery due to magnet dislocation in cochlear implant patients: an emerging complication. Otol Neurotol. 2014;35(1):29–34.
- Tam Y, Lee J, Gair J, et al. Performing MRI scans on cochlear implant and auditory brainstem implant recipients: review of 14.5 years experience. Otol Neurotol. 2020;41(5):e556–e562.
- Walker B, Norton S, Phillips G, et al. Comparison of MRI in pediatric cochlear implant recipients with and without retained magnet. Int J Pediatr Otorhinolaryngol. 2018;109:44–49.
- MED-EL. Medical Procedures for MED-EL CI/ABI Systems. (undated). Available at: https://www.medel.com/docs/default-source/isi-important-safety-information/languages/en-english/ci-cochlear-implants/v4/aw33289_80_manual-medical-procedures-ci-abi-systems-(en-english).pdf?sfvrsn=43eb6842_2. (accessed 22-Jun-2020).
- Chinese expert consensus on safety management of MRI[J]. Chinese Journal of Radiology. 2017;(10)
- Hirokawa Y, Isoda H, Maetani YS, et al. MRI artifact reduction and quality improvement in the upper abdomen with PROPELLER and prospective acquisition correction (PACE) technique. AJR Am J Roentgenol. 2008;191(4):1154–1158.
- Pipe JG. Motion correction with PROPELLER MRI: application to head motion and free-breathing cardiac imaging. Magn Reson Med. 1999;42(5):963–969.
- Edmonson HA, Carlson ML, Patton AC, et al. MR imaging and cochlear implants with retained internal magnets: reducing artifacts near highly inhomogeneous magnetic fields. Radiographics. 2018;38(1):94–106.
- Schwarz-Nemec U, Dahm V, Arnoldner C. Letter to the editor regarding worldwide 1st MED-EL Mi1200 SYNCHRONY cochlear implant magnet removal for MRI image artifact reduction by wieser et al. Otolaryngology Case Reports. 2019;10:43–44.
- Wimmer W, Hakim A, Kiefer C, et al. MRI metal artifact reduction sequence for auditory implants: first results with a transcutaneous bone conduction implant. Audiol Neurootol. 2019;24(2):56–64.
- Utrilla C, Gavilán J, García-Raya P, et al. MRI after bonebridge implantation: a comparison of two implant generations. Eur Arch Otorhinolaryngol. 2021;278(9):3203–3209.