Abstract
The mitochondrial genomes of three North American stygobiont amphipods Stygobromus tenuis potomacus, S. foliatus and S. indentatus collected from Caroline County, VA, were sequenced using a shotgun sequencing approach on an Illumina NextSeq500 (Illumina Inc., San Diego, CA). All three mitogenomes displayed 13 protein-coding genes, 22 tRNAs and two rRNAs typical of metazoans. While S. tenuis and S. indentatus displayed identical gene orders similar to the pancrustacean ground pattern, S. foliatus displayed a transposition of the trnL2-cox2 genes to after atp8-atp6. In addition, a short atp8 gene, longer rrnL gene and large inverted repeat within the Control Region distinguished S. foliatus from S. tenuis potomacus and S. indentatus. Overall, it appears that gene order varies considerably among amphipods, and the addition of these Stygobromus mitogenomes to the existing sequenced amphipod mitogenomes will prove useful for characterizing evolutionary relationships among various amphipod taxa, as well as investigations of the evolutionary dynamics of the mitogenome in general.
Introduction
The mid-Atlantic United States harbours a diverse array of stygobiont fauna associated with shallow groundwater habitats with over 14 species of amphipods within the genus Stygobromus described from within this region (Holsinger et al. Citation2011; Culver et al. Citation2012). Because these species occupy shallow groundwater habitats, they are particularly vulnerable to habitat degradation, and some species are threatened with extirpation.
Numerous amphipod mitogenomes have been sequenced recently revealing differences amongst distantly related species (Krebes & Bastrop Citation2012). Less common are mitogenomic comparisons among amphipods of the same genus. Pons et al. (Citation2014) found that gene order was mostly conserved among seventeen amphipod species of the genus Metacrangonyx, whereas Stokkan et al. (Citation2015) found a high level of rearrangement of protein coding genes among three amphipod species of the genus Pseudoniphargus. To provide additional insight into amphipod mitogenomic structure and evolution, we sequenced the mitogenomes of three species of amphipods in the genus Stygobromus to include Stygobromus tenuis potomacus, S. indentatus and S. foliatus and performed a brief comparative analysis with other amphipod mitogenomes.
Methods
Stygobromus tenuis potomacus (38.1691 °N–77.2781°W), S. indentatus (38.0987 °N–77.1476 °W) and S. foliatus (38.1995 °N–77.2905 °W) were collected by hand from within leaf litter at seepage springs at Fort A.P. Hill, Caroline County, VA, in March 2014, and field preserved in 95% ethanol. Species identification was performed using taxonomic keys and published species descriptions presented in Holsinger (Citation1967, Citation1969, Citation1978) and Holsinger et al. (2011). Specimens are stored at the US Geological Survey Leetown Science Center, Kearneysville, WV. Libraries were prepared from whole genomic DNA using the TruSeq Nano library preparation kit (Illumina, San Diego, CA) for 2 × 150bp paired-end sequencing on an Ilumina NextSeq500 sequencer (Illumina, San Diego, CA).
The annotated mitochondrial genomes of 26 amphipods were used for comparative analyses (). The location of protein coding genes was determined using the online program DOGMA (Wyman et al. Citation2004). Annotation of start and stop codons was based on extensive comparisons of candidate protein coding genes to the annotation of the same genes in other amphipod mitogenomes. Annotation of the large ribosomal subunit (rrnL) was defined as all base pairs between the adjacent tRNAs. In contrast, the ends of the small ribosomal subunits (rrnS) were determined through alignment with other amphipod rrnS genes to look for conserved regions indicating the likely start of rrnS, as well as the end of rrnS and the start of the control region. The location and secondary structures of the tRNA genes were identified through the automated online mitogenome annotation software MITOS (Bernt et al. Citation2013). A maximum likelihood phylogenetic tree with 500 bootstrap replications was created in the program MEGA7 (Kumar et al. Citation2016) using a MUSCLE alignment (Edgar Citation2004) generated in the program TranslatorX (Abascal et al. Citation2010) of thirteen protein coding genes from 23 other selected members of the order Amphipoda.
Table 1. Species names, GenBank accession numbers and mitogenome length of 26 amphipod mitogenomes used for comparative analyses with Stygobromus tenuis, S. foliatus and S. indentatus.
Results and discussion
The complete mitogenomes of S. tenuis potomacus (14,915 bp, GenBank accession no. KU869712) and S. indentatus (14,638 bp, GenBank accession no. KU869711) were obtained along with the nearly complete mitogenome of S. foliatus (GenBank accession no. KU869713). The mitogenome of S. foliatus contained an inverted repeat of the control region, of which 365 bp were sequenced in each direction (bps 13,225–14,075) with the un-sequenced remainder annotated as a gap of unknown length. All mitogenomes consisted of 13 protein-coding genes, an rrnS and rrnL gene, and 22 tRNA genes typical of metazoans. In S. tenuis potomacus and S. indentatus, 23 genes were encoded on the + strand and 14 on the – strand, whereas S. foliatus had 22 genes on the + and 15 on the – strand. The same four protein-coding genes (nad5, nad4, nad4l and nad1) were on the – strand in all three species, which is a feature of the pancrustacean ground pattern (Boore et al. Citation1998). The AT% of S. tenuis potomacus and S. indentatus was 69%, which is typical of values observed in other amphipods. Results of the phylogenetic analysis () suggest that S. tenuis potomacus, S. indentatus and S. foliatus form a distinct well-supported clade.
Figure 1. Phylogenetic tree indicating the relationships of Stygobromus tenuis potomacus, S. indentatus and S. foliatus with 23 other members of the order Amphipoda generated using the maximum-likelihood method based on 13 complete protein-coding genes from the mitochondrial genome. GenBank accession numbers are in parentheses next to the species names.
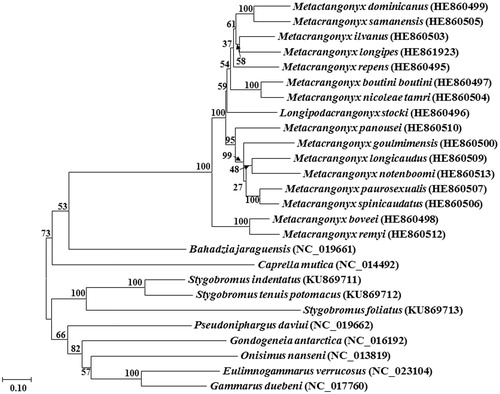
Stygobromus foliatus had substantially more intergenic spacers (n = 23), which were often between protein coding genes, than was observed in S. tenuis potomacus (n = 9) or S. indentatus (n = 7) or any other examined amphipod mitogenome. In S. foliatus, we hypothesize these numerous intergenic spacers are a consequence of numerous transpositions and reverse transpositions of tRNAs.
Both S. tenuis potomacus and S. indentatus had identical gene orders, which were most similar to the pancrustacean ground pattern with the exception of transposition of some tRNAs (Boore et al. Citation1998; Pons et al. Citation2014), while S. foliatus displayed some unique rearrangements. Stygobromus tenuis potomacus and S. indentatus share the conserved gene block order of cox1–trnL2–cox2 with other examined amphipods. In contrast, S. foliatus displays a transposition of trnL2–cox2 downstream after atp8–atp6. Stygobromus foliatus also departed from the pancrustacean gene order of nad5–trnH–nad4–nad4l, with transposition of the trnH downstream between nad2 and trnG. In addition, S. foliatus exhibited numerous reverse transposed tRNAs compared with the pancrustacean ground pattern and other examined amphipods.
Most protein-coding genes utilized ATN start codons, with ATG and ATA being the most frequent. Unconventional start codons include a TTG for nad1 in S. tenuis potomacus, and a GTG start codon for the nad4 gene in S. foliatus, which have not been used for these genes in other compared amphipod mitogenomes. Stop codons were most frequently TAA or TAG, although several genes ended with an incomplete T– – or TA–. These abbreviated stop codons are common in amphipods (Pons et al. Citation2014), and it is assumed that these are formed into complete terminal codons through post-transcriptional polyadenylation (Ojala et al. Citation1981).
Numerous deviations in length and overlap at some protein coding genes within S. tenuis potomacus, S. indentatus, and S. foliatus were observed. The cox1 gene in S. foliatus was 20 bp longer than within S. tenuis potomacus and S. indentatus, with extra nucleotides near the start and the end of the gene accounting for the length difference. In all three species, the cox1 gene began with two consecutive ATT-ATV start codons, which did not overlap with the adjacent tRNA, and which we annotated the first as the beginning of cox1. Several species of the genus Metacrangonyx have an extra candidate start codon for cox1 overlapping the adjacent tRNA by 1 bp, but are annotated as beginning with the second start codon. Similarly other species such as Onisimus nanseni and Gondogeneia antarctica have alternate candidate start ATC start codons between the adjacent tRNA and their existing annotated start. The atp8 gene within S. foliatus (105 bp) was substantially shorter than within S. tenuis potomacus (186 bp) and S. indentatus (177 bp), as well as in comparison with atp8 within the other compared amphipod mitogenomes (range =156–162 bp). While no other described amphipods to date have such a short atp8 gene as S. foliatus, Zhang et al (Citation2013) described two species of rice planthoppers (subphylum Hexapoda) that possessed conspicuously short atp8 genes of 99 and 102 bp, but the significance of a shortened atp8 gene in any of these species is unknown. Another feature of the S. foliatus atp8 gene was that it only overlapped with the adjoining atp6 by one bp, whereas S. tenuis and S. indentatus had overlaps of 41 bp with atp6. These large overlaps between atp6 and atp8 have not yet been reported to our knowledge for any amphipod.
The S. tenuis potomacus, S. foliatus and S. indentatus mitogenomes all encoded 22 tRNA genes typical of metazoan mitochondrial genomes (Wolstenholme Citation1992). Stygobromus tenuis potomacus and S. indentatus possessed the same 14 tRNAs on the heavy strand, and eight tRNAs on the light strand. Stygobromus foliatus had 13 tRNAs on the heavy strand, and nine on the light strand, of which five were on different strands than within S. tenuis potomacus and S. indentatus. The lengths of the tRNAs ranged from 50 to 66 bps, and all formed typical cloverleaf-like secondary structures, though both trnS1 and trnS2 lacked the DHU arm, and trnQ lacked the TΨC stem.
The rrnL gene within S. foliatus when aligned with other amphipods possessed large overhangs on either end, and was longer than within S. tenuis potomacus, and S. indentatus. In the amphipod mitogenomes used for comparison in this study as well as in S. tenuis potomacus and S. indentatus, rrnL is flanked by trnL1 and trnV, whereas in S. foliatus it is flanked by trnS and trnQ. Because the 3′ and 5′ ends of the rrnL gene are not well conserved, we have tentatively retained these overhangs in the annotation of rrnL in S. foliatus. In contrast to S. foliatus, the S. indentatus and S. tenuis potomacus rrnL genes were similar in length to rrnL within other amphipods.
An alignment of the rrnS gene among S. foliatus, S. indentatus, S. tenuis potomacus and other amphipod mitogenomes revealed a relatively conserved 5′ end. This resulted in annotation of a 46 bp spacer between the adjacent trnI and start of the rrnS gene in S. foliatus. On the 3′ end of the rrnS gene, S. tenuis potomacus and S. indentatus were annotated as ending at the start of a string of several T’s as was done for annotations of rrnS in Gammarus duebeni (Krebes & Bastrop Citation2012) and various species of Metacrangonyx (Pons et al. Citation2014). This string of T’s is interpreted as the beginning of the Control Region. In contrast, the rrnS gene in S. foliatus did not terminate at a string of T’s, but at an inversion of the control region. 365 bp were sequenced from both ends of the inversion, which was nearly perfect with greater than 99% sequence similarity when the ends were aligned. Large inverted repeats of the control region are characteristic of some amphipods as noted by Pons et al. (Citation2014).
Conclusions
Here, we have presented the complete mitogenomes of the stygobiont amphipods S. tenuis potomacus and S. indentatus, along with the nearly complete mitogenome of S. foliatus, adding to the growing number of available amphipod mitogenomes. While all three mitogenomes possessed the typical suite of genes found in other amphipods, some unique gene arrangements such as the transposition of trnL2–cox2 downstream after atp8–atp6 in S. foliatus has not been observed yet in any other amphipod, and differs from the pancrustacean ground pattern. While these rearrangements of protein-coding genes at the genus level are rare, they have been observed in other amphipod genera (Stokkan et al. Citation2015). Overall, these additional mitogenomes will prove useful for investigating phylogenetic relationships among various amphipod groups, and contribute to studies of mitogenome evolution in general.
Acknowledgements
The authors would like to thank Robert H. Floyd, Jason R. Applegate, Brian W. Josey and Stefanie Ferrazzano from Fort A.P. Hill for coordinating access and providing assistance with field activities. Roger M. Moberg, Jr., David S. Powars, and Michael Eackles of the U.S. Geological Survey also assisted with collection of water and geologic samples. Use of trade, product, or firm names does not imply endorsement by the U.S. government.
Disclosure statement
The authors declare that they have no competing or conflicting interests.
Funding information
Funding for this study was provided by the U.S. Army, Fort A.P. Hill Environmental Directorate.
References
- Abascal F, Zardoya R, Telford MJ. 2010. TranslatorX: multiple alignment of nucleotide sequences guided by amino acid translations. Nucleic Acids Res. 38:7–13.
- Bernt M, Donath A, Jühling F, Externbrink F, Florentz C, Fritzsch G, Pütz M, Middendorf M, Stadler PF. 2013. MITOS: improved de novo metazoan mitochondrial genome annotation. Mol Phylogenet Evol. 69:313–319.
- Boore JL, Lavrov DV, Brown WM. 1998. Gene translocation links insects and crustaceans. Nature. 392:667–668.
- Culver DC, Holsinger JR, Feller D. 2012. The fauna of seepage springs and other shallow subterranean habitats in the Mid-Atlantic Piedmont and Coastal Plain. Northeast Nat. 19:1–42. (Monograph)
- Edgar RC. 2004. MUSCLE: multiple sequence alignment with high accuracy and high throughput. Nucleic Acids Res. 32:1792–1797.
- Holsinger JR. 1967. Systematics, speciation, and distribution of the subterranean amphipod genus Stygonectes (Gammaridae). B US Nat Mus. 259:1–176.
- Holsinger JR. 1969. Systematics of the North American subterranean amphipod genus Apocrangonyx (Gammaridae), with remarks on ecology and zoogeography. Am Midl Nat. 81:1–28.
- Holsinger JR. 1978. Systematics of the subterranean amphipod genus Stygobromus (Crangonyctidae), Part 2: Species of the eastern United States. Sm C Zool. 266:1–144.
- Holsinger JR, Ansell LM, Shafer J. 2011. Four new species of the subterranean amphipod genus Stygobromus (Amphipoda: Crangonyctidae) from shallow groundwater habitats on the Coastal Plain and eastern margin of the Piedmont in Maryland and Virginia, USA. Zootaxa. 2972:1–21.
- Krebes L, Bastrop R. 2012 . The mitogenome of Gammarus duebeni (Crustacea Amphipoda): a new gene order and non-neutral sequence evolution of tandem repeats in the control region. Comp Biochem Physiol Part D: Genomics Proteomics. 7:201–211.
- Kumar S, Stecher G, Tamura K. 2016. MEGA7: molecular evolutionary genetics analysis version 7.0 for bigger datasets. Mol Biol Evol. [Epub ahead of print]. doi: 10.1093/molbev/msw054.
- Ojala D, Montoya J, Attardi G. 1981. tRNA punctuation model of RNA processing in human mitochondria. Nature. 290:470–474.
- Pons J, Bauzà-Ribot MM, Jaume D, Juan C. 2014. Next-generation sequencing, phylogenetic signal and comparative mitogenomic analyses in Metacrangonyctidae (Amphipoda: Crustacea). BMC Genom. 15:566.
- Stokkan M, Jurado-Rivera J, Carlos J, Damià J, Pons J. 2015. Mitochondrial genome rearrangements at low taxonomic levels: three distinct mitogenome orders in the genus Pseudoniphargus. Mitochondrial DNA. 27:3579–3589.
- Wolstenholme DR. 1992. Animal mitochondrial DNA: structure and evolution. Int Rev Cytol. 141:173–216.
- Wyman SK, Jansen RK, Boore JL. 2004. Automatic annotation of organellar genomes with DOGMA. Bioinformatics. 20:3252–3255.
- Zhang K, Zhu W, Rong X, Zhang Y, Ding X, Liu J, Chen D, Du Y, Hong X. 2013. The complete mitochondrial genomes of two rice planthoppers, Nilaparvata lugens and Laodelphax striatellus: conserved genome rearrangement in Delphacidae and discovery of new characteristics of atp8 and tRNA genes. BMC Genomics. 14:417.