Abstract
The complete mitochondrial genomes of four fish species of the commercially important family Latidae were sequenced using the Illumina MiSeq, thereby significantly increasing the mitogenomic resources for the family. Whole mitogenome-based phylogenetic analysis supports the monophyly of the genus Lates and more generally the family Latidae. The mitogenome sequences from this study will be useful for future assessments of the diversity within and between Lates species and studies of phylogenetic relationships within the diverse and taxonomically challenging perciform fishes.
Keywords:
The family Latidae currently contains 13 extant perch-like fishes from three genera, Hypopterus Gill, 1861, Lates Cuvier, 1828 and Psammoperca Richardson, 1848, which are found in a range of freshwater, brackish, and marine environments across Asia, Africa, and the Western Pacific and Indian oceans (Eschmeyer et al. Citation2016; Nelson et al. Citation2016). Despite low diversity, the family contains a number of commercially important species including the Asian sea bass or barramundi, Lates calcarifer and the Nile Perch, L. niloticus.
Psammoperca waigiensis or the Waigieu sea perch is the only member of the genus Psammoperca (Froese & Pauly Citation2016) and ranges widely across the Indo-West Pacific, from the Bay of Bengal to Japan to the northern and western coasts of Australia (Randall et al. Citation1990). In contrast, L. japonicus or akame or the Japanese lates has a relatively restricted range in the north western Pacific around Japan (Masuda et al. Citation1984). It is morphologically similar to both P. waigiensis and L. calcarifer, and was only declared a distinct species in 1984 (Katayama & Yasuhiko Citation1984). Unlike most of the other members of the family above, L. niloticus or the Nile perch is a purely freshwater species from north Africa that is of both economic importance and conservation concern as it has been introduced to several African lakes including the biological diverse Lake Victoria (Pringle Citation2005).
With the exception of L. calcarifer (Lin et al. Citation2006; Vij et al. Citation2014), genomic resources for the family Latidae are still fairly limited. In addition, from an evolutionary and taxonomic perspective, the status and relationships of the family to other Perciform groups is uncertain or unresolved (Vij et al. Citation2014; Harrington et al. Citation2016).
The sample of L. japonicus (Shi04) used for this study was collected from Shiomi River, Miyazaki Prefecture, Japan as described elsewhere (Takahashi et al. Citation2015). The sample of P. waigiensis was collected from Vesteys Beach, Darwin, in the Northern Territory, Australia (12.436° S, 130.829° E) with tissue and matched voucher specimen held at the Museum and Art Gallery of the Northern Territory (catalogue number: S.16708-010). The samples of the commercially important L. calcarifer and L. niloticus were obtained as muscle (fillets) from fish markets in Darwin, Australia sourced from the Northern Territory and Lake Victoria (imported), respectively.
Genomic DNA (gDNA) was extracted from approximately 50 mg fin clip or muscle tissue using the EZDNA Tissue extraction kit (OmegaBioTek, Norcross, GA). Approximately 100–200 ng of gDNA was sheared to 500 bp using a M220 focused-ultrasonicator (Covaris, Woburn, MA), prepped using NEBNext Ultra DNA library prep kit and subsequently sequenced on the MiSeq desktop sequencer (Illumina, San Diego, CA) located at the Monash University Malaysia Genomics Facility. Mitogenome assembly was performed using MITObim (Hahn et al. Citation2013). For L. niloticus, L. japonicas, and P. waigiensis, the COX1 gene fragment for each species was used as the bait for iterative mapping assembly while for the L. calcarifer sample, the complete mitogenome already available for the species (GenBank: DQ010541) (Lin et al. Citation2006) was used instead. Each mitogenome was recircularized based on the presence of flanking repeat sequence as previously described (Gan et al. Citation2014) and annotated using MitoAnnotator (Iwasaki et al. Citation2013). For the construction of maximum-likelihood tree, 13 protein-coding genes and 2 ribosomal RNA genes were extracted, individually aligned (Abascal et al. Citation2010; Katoh & Standley Citation2014) and concatenated to form a super-alignment. The best-fit partitioning scheme was calculated using ModelFinder (Nguyen et al. Citation2015). Based on the optimum partitioning scheme, a maximum-likelihood (ML) tree was constructed using IQ-TREE with the 1000 ultrafast bootstrap approximation option to assess branch supports (Nguyen et al. Citation2015). The consensus tree was visualized and graphically edited using FigTree v 1.4.2 (http://tree.bio.ed.ac.uk/software/figtree/).
Partial genome sequencing successfully recovered the complete mitogenomes of all fish species obtained for this study, increasing the number of complete mitogenome for species of the family Latidae from one species (L. calcarifer) to a total of four. The latid mitogenomes have the typical structure organization of a fish mitogenome and consist of the typical 13 protein-coding genes, 22 transfer RNA genes and 2 ribosomal subunit genes, and a non-coding putative control region.
The mitogenome length and AT content ranges from 16,510 to 16,684 bp and 53.19 to 55.27%, respectively. The recovered mitogenomes exhibit high nucleotide similarity (> 95%) to the partial mitochondrial genes of members of the same species available on NCBI, providing support to their species designation based on morphology criteria or labelling as fish products. For example, our sample of L. niloticus was a fillet obtained from a fish market, but it had a 99% sequence similarity to the partial COX1 gene of L. niloticus isolate FLN210 (GenBank: KJ443713), which was sampled in Egypt, the type locality for L. niloticus confirming the sample was true to label.
At the whole mitogenome level, P. waigiensis is the most divergent member of the Latidae sequenced with an average pairwise nucleotide identity of 79.8% (79.4–80.0%) to members of the genus Lates. The sample of L. calcarifer sequenced in this study is more similar to that of the Australian L. calcarifer isolate P12C03 (99.6%) at the COX1 gene compared to that of Asian L. calcarifer (98.6%), which is consistent with its stated origin from near Darwin, Australia. Within the genus Lates our sample of L. calcarifer exhibits a similar pair-wise nucleotide identity (whole mitogenome alignment) to L. japonicus and L. niloticus (86.7% and 86.2%, respectively).
ModelFinder identified four partitions as the best-fit partitioning scheme for the 13 PCG and 2 rRNA genes utilized for phylogenetics inference as follows: Partition1 = 12S and 16S; Partition2= ATP6, CYTB, COX3, ND3, COX1, COX2; Partition3= ATP8, ND3, ND3 ND4L, ND2; Partition4= ND6). Phylogenetic analysis using maximum likelihood, based on the final partitioned nucleotide alignment consisting of 14,270 DNA characters, supports the monophyly of the genus Lates and to a certain extent the monophyly of the families Latidae, Carangidae, and Xiphiidae (). However, within the Latidae a sister grouping between L. japonicus and L. calcarifer was only weakly supported (ultrafast bootstrap support =56%) and the relationships among all three species of Lates should be considered pending further taxon and gene sampling. In general, the evolutionary relationships among the different families included in the study are poorly supported and may similarly benefit from a more extensive taxon and gene sampling, which is beyond the scope of this study.
Figure 1. Phylogenetic tree depicting the evolutionary relationships within the order, inferred from maximum-likelihood estimation based on 13 mitochondrial protein-coding genes and two ribosomal RNA genes with best-fit partitioning scheme. Members from the family Acanthuridae were used as an outgroup and a selection of species from families thought to be related to the Latidae were included for comparative purposes. Circles next to tip labels indicate sequence reported in this study. NCBI accession numbers were provided next to tip labels (in red). Numbers at nodes indicate Ultrafast Bootstrap support and branch lengths indicate number of substitutions per site.
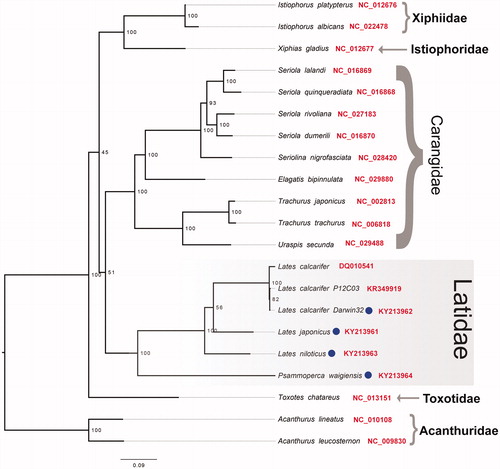
Acknowledgements
Funding for this study was provided by the Monash University Malaysia Tropical Medicine and Biology Multidisciplinary Platform.
Disclosure statement
The authors report no conflicts of interest and the authors alone are responsible for the content and writing of the paper.
Additional information
Funding
References
- Abascal F, Zardoya R, Telford MJ. 2010. TranslatorX: multiple alignment of nucleotide sequences guided by amino acid translations. Nucleic Acids Res. 38:W7–13.
- Eschmeyer WN, Fricke R, Van Der Laan R. 2016. Catalog of fishes: Genera, Species, References [Internet]. [cited 2016 Nov 17]. Available from: http://researcharchive.calacademy.org/research/ichthyology/catalog/fishcatmain.asp
- Froese R, Pauly D. 2016. FishBase (version Aug 2016). In: Roskov Y, Abucay L, Orrell TM, Nicolson D, Flann C, Bailly N, Kirk P, Bourgoin T, Dewalt RE, DECOCK W, de Wever A, editors. Species 2000 & ITIS catalogue of life. Naturalis, Leiden, the Netherlands: Species 2000.
- Gan HM, Schultz MB, Austin CM. 2014. Integrated shotgun sequencing and bioinformatics pipeline allows ultra-fast mitogenome recovery and confirms substantial gene rearrangements in Australian freshwater crayfishes. BMC Evol Biol. 14:19.
- Hahn C, Bachmann L, Chevreux B. 2013. Reconstructing mitochondrial genomes directly from genomic next-generation sequencing reads–a baiting and iterative mapping approach. Nucleic Acids Res. 41:e129.
- Harrington RC, Faircloth BC, Eytan RI, Smith WL, Near TJ, Alfaro ME, Friedman M. 2016. Phylogenomic analysis of carangimorph fishes reveals flatfish asymmetry arose in a blink of the evolutionary eye. BMC Evol Biol. 16:224.
- Iwasaki W, Fukunaga T, Isagozawa R, Yamada K, Maeda Y, Satoh TP, Sado T, Mabuchi K, Takeshima H, Miya M, Nishida M. 2013. MitoFish and MitoAnnotator: a mitochondrial genome database of fish with an accurate and automatic annotation pipeline. Mol Biol Evol. 30:2531–2540.
- Katayama M, Yasuhiko T. 1984. Lates japonicus, a New Centropomid Fish from Japan. JpnJ Ichthyol. 30:361–367.
- Katoh K, Standley DM. 2014. MAFFT: iterative refinement and additional methods. Methods Mol Biol. 1079:131–146.
- Lin G, Lo LC, Zhu ZY, Feng F, Chou R, Yue GH. 2006. The complete mitochondrial genome sequence and characterization of single-nucleotide polymorphisms in the control region of the Asian seabass (Lates calcarifer). Mar Biotechnol (NY). 8:71–79.
- Masuda H, Amaoka K, Araga C, Uyeno T. 1984. The fishes of the Japanese Archipelago. Tokyo, Japan: Tokai University Press.
- Nelson JS, Grande TC, Wilson MVH. 2016. Fishes of the world. 5th ed. Hoboken (NY): John Wiley & Sons.
- Nguyen LT, Schmidt HA, Von Haeseler A, Minh BQ. 2015. IQ-TREE: a fast and effective stochastic algorithm for estimating maximum-likelihood phylogenies. Mol Biol Evol. 32:268–274.
- Pringle RM. 2005. The Origins of the Nile Perch in Lake Victoria. BioScience. 55:780–787.
- Randall JE, Allen GR, Steene RC. 1990. Fishes of the Great Barrier Reef and Coral Sea. Honolulu, Hawaii: University of Hawaii Press.
- Takahashi H, Takeshita N, Tanoue H, Ueda S, Takeshima H, Komatsu T, Kinoshita I, Nishida M. 2015. Severely depleted genetic diversity and population structure of a large predatory marine fish (Lates japonicus) endemic to Japan. Conserv Genet. 16:1155–1165.
- Vij S, Purushothaman K, Gopikrishna G, Lau D, Saju JM, Shamsudheen KV, Kumar KV, Basheer VS, Gopalakrishnan A, Hossain MS, et al. 2014. Barcoding of Asian seabass across its geographic range provides evidence for its bifurcation into two distinct species. Front Mar Sci. [Internet]. Available from: http://journal.frontiersin.org/article/10.3389/fmars.2014.00030/full