Abstract
The present study investigated the single nucleotide variants (SNVs) in mitochondrial DNA (mtDNA) of 13 paired gastric cancer tissue samples and seven gastric cancer cell lines using direct sequencing analysis of the MTND5 region. Results showed that nuclear mitochondrial pseudogenes (NUMTs) and mitochondrial copy number affected the detection of the SNV frequency in gastric cancer tissue and cell line samples using high-throughput sequencing technique. The heteroplasmic point mutation C12474T and G12835A happened in AGS and BGC823 cell lines, respectively. A total of seven SNVs were found in three paired gastric cancer tissue samples, including five heteroplasmic point mutations (A12406G, C12705T, T12882C, G12501A, and A12584G) and two homoplasmic point mutations (G12561A and C13590T). Gastric cancer tissue sample 16 exhibited the highest SNVs frequency with four SNVs (np 12406, np 12705, np 12882, and np 12501), whereas no SNVs or SNPs were detected in the tissue sample 4. SNP 12705 turned out to be an SNV in gastric cancer tissue sample 16. SNV 12338 detected by exome sequencing approach appeared to be an SNP in this study.
Introduction
Mitochondrion is one of the most important organelle in cells that plays important roles in numerous biological activities (Zamzami and Kroemer Citation2001). For example, it has been confirmed that mitochondrion takes charge of supplying cellular energy through generating adenosine triphosphate (ATP) in cells. Additionally, reactive oxygen species (ROS), one of the most important signalling molecules, are produced and utilized by mitochondria and other organelles in eukaryotes (Sena and Chandel Citation2012; Kim et al. Citation2017). More importantly, mitochondria exert an essential role in cellular differentiation and cell death (Galluzzi et al. Citation2012; Márquez-Jurado et al. Citation2018).
The mitochondrial genome investigation has suggested that mitochondrion consists of a 16,569 bp circular double-stranded DNA sequence that could encode two rRNAs, 13 respiratory enzyme complex proteins, and 22 tRNAs (Anderson et al. Citation1981). Reactive oxygen species generated in cells during cell stress could induce damage to cell structures through cleaving lipid, DNA, RNA, and protein (Holzerová and Prokisch Citation2015). Compared to nuclear DNA (nDNA) in cells, mitochondrial DNA (mtDNA) lacks protective histones and exhibits deficiency on DNA repairing mechanisms (Croteau and Bohr Citation1997). Therefore, ROS in cells could result in more damage on mtDNA than nDNA, which could cause the mutations of mtDNA (Gu et al. Citation2016). The accumulation of the mtDNA mutations in cells can decrease its electron transport capacity, which can further decrease the ATP production and increase the level of the ROS. Such mutations could lead to disorders in cell metabolism and thus cause various human diseases (Wallace Citation2012; Dhillon and Fenech Citation2014; Lightowlers et al. Citation2015; Kim et al. Citation2016). For example, diseases caused by mitochondrial dysfunctions include several types of cancer, Kearns–Sayre syndrome, MELAS syndrome, Parkinson’s disease, Leigh syndrome, myelodysplastic syndrome, diabetes mellitus, and Leber’s hereditary optic neuropathy (Chatterjee et al. Citation2006; Connolly et al. Citation2010; Gupta et al. Citation2013; Montiel-Sosa et al. Citation2013; Eckenweiler et al. Citation2015; Coxhead et al. Citation2016; Kytövuori et al. Citation2016; Xu et al. Citation2017).
Gastric cancer is one of the most common cancers all over the world, and it has been confirmed to be the second most common cancer that causes a high mortality rate (Thun et al. Citation2010; Deans et al. Citation2011). Gastric cancer incidence has become an increasing trend to Asian population, and it has shown a high mortality rate in China nowadays (Chen et al. Citation2013). Our preliminary study has revealed that a high mutation rate took place in MTND5 gene in gastric cancer paired clinical tissues using exome sequencing technique, indicating that the MTND5 mutation could be used as an indicator to estimate the incidence of gastric cancer. Therefore, the present study aimed to validate our hypothesis through analysing mitochondrial single nucleotide variants (SNVs) in gastric cancer tissue and cell lines using polymerase chain reaction (PCR) and Sanger sequencing approaches. The findings from this study could provide practical reference on tumour molecular marker for gastric cancer.
Materials and methods
DNA extraction of gastric cancer cell lines and tissue
Gastric cancer cell lines used in this study included BGC823, MGC803, SGC7901, MKN45, AGS, N87, and GES1. The cell lines BGC823, MGC803, and SGC7901 were provided from the Tissue Bank of Shanghai (Shanghai, China). The cell line GES1 was received from the Peking University Cancer Hospital and Institute (Beijing, China). The cell lines MKN45, N87, and AGS were purchased from the American Type Culture Collection (Manassas, VA). These cell lines were cultured in the DMEM medium supplemented with 10% foetal bovine serum in an incubator with 37 °C and 5% CO2 condition. Additionally, a total of 13 human gastric cancer specimens and their corresponding adjacent normal tissue samples were provided from the Peking University Cancer Hospital and Institute (Beijing, China). These cancer and adjacent normal tissue samples were collected from the patients during the surgery at the Peking University Cancer Hospital and Institute (Beijing, China). Both gastric cancer cell lines and tissues were extracted using QIAamp DNA Mini Kit to collect genomic DNA according to the manufacture’s instruction (QIAGEN, Hilden, Germany). The genomic DNA (total DNA) in these cell lines and tissues consisted of mtDNA and nDNA. These genomic DNA samples after extraction were immediately stored at –80 °C prior to further analysis.
Polymerase chain reaction and DNA sequencing
Four primer pairs were designed to amplify the entire length of the mitochondrial ND5 (MTND5) gene using Primer 5.0 Software (Primer Premier, Palo Alto, CA) regarding the NCBI GenBank revised Cambridge Reference Sequence (rCRS) (Anderson et al. Citation1981; Andrews et al. Citation1999) and our previous MTND5 mutations exome sequencing data (Table S1). The detailed information is listed in . These primers were synthesized from AuGCT Biotech Company (Beijing, China). A total 100 ng DNA amount from each sample was used as a template for the PCR analysis in a 50-µL reaction volume. The PCR condition was carried out as follows: initial denaturation at 94 °C for 5 min, 30 cycles at 94 °C for 30 seconds, annealing at 60 °C for 30 seconds, an extension for MTND5-1, 2, 3 and 4 at 72 °C for 30 seconds and 90 seconds for MTND5 (1–3) and MTND5 (3–4), and a final extension at 72 °C for 10 min. The PCR products were then analysed using agarose gel electrophoresis and purified through QIAquick PCR Purification Kit according to the manufacture instruction (QIAGEN, Hilden, Germany). The DNA fragments were analysed using direct sanger sequencing under Applied Biosystem 3730XL automatic DNA sequencer (Thermo Fisher Scientific, Waltham, MA) from AuGCT Biotech Company (Beijing, China).
Table 1. Primer sequences detecting MTND5 gene.
Results
Nuclear mitochondrial pseudogenes
Table S1 shows the preliminary exome sequencing results from 10 paired gastric cancer tissue samples. It was found that a total of 20 SNVs appeared in these five paired samples. Meanwhile, the sequencing results revealed that the SNV sites in the mtDNA were more than that in the nDNA. In the present study, we amplified the entire MTND5 gene from the total DNA in the gastric cancer cell lines and 13 gastric cancer tissue samples. After the PCR amplification, the PCR products were then sequenced. These sequences were searched against the GenBank database. It was found that the MTND5 from the tissue sample 4 exhibited a 100% and 93% identity with the rCRS and Chr 5 (NC_000005.10, 134926459–134924648) according to the GenBank database, respectively ().
Figure 1. In the sequencing map, SNP and SNV in gastric cancer tissue and adjacent normal tissue. (A) The nucleotide at np 12338 is SNP in present study. In gastric cancer tissue and adjacent normal tissue of No. 14 or No. 8, the nucleotide at np 12338 was the same. There were different nucleotides at np 12338 in No. 14 and No. 8 normal tissue samples. (B) In the sequencing map, double peaks were detected at np 12406, 12882, 12705, 12501. They were SNVs. In No. 16 gastric cancer tissue sample, there are two kinds of nucleotides A and G at np 12406, and there are two kinds of nucleotides C and T at np 12882; T and C were detected at np 12705 in No. 16 gastric cancer tissue; G and A are detected at np 12501 in No. 16 gastric cancer tissue samples; G is detected at np 12501 in normal tissue. (C) It is an SNV at np 13590 in No. 13 paired tissue samples (sequencing map of complementary DNA strand), all of A at np 13590 in normal tissue mutate to A in gastric cancer tissue; in No. 5 paired tissue samples (sequencing map of complementary DNA strand), only A is detected in both of gastric cancer tissue and normal tissue. it is a wildtype at np 13590; G is detected at np 12561 in No. 13 adjacent tissue sample, and whole G mutate to A in gastric cancer tissue sample. G and A are detected at np 12584 in No. 9 gastric cancer tissue samples; A is detected at np 12584 in normal tissue. Part of A at np 12584 mutate to G in gastric cancer tissue sample.
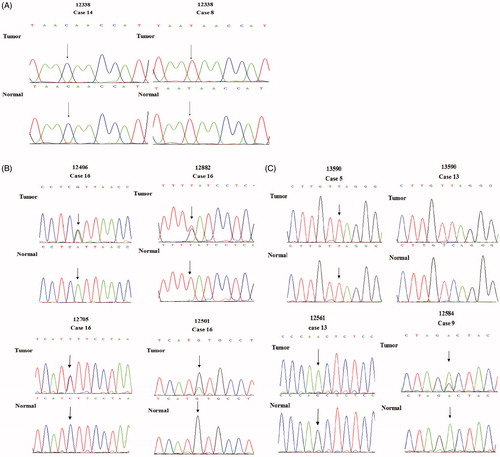
It should be worth noting that different nucleotides between the MTND5 and Chr 5 (134926459–134924648) were interspersed throughout the MTND5 gene. However, no large nucleotide segments were found to be different. Therefore, we further designed the specific primers in the low sequence similarity region between the MTND5 and Chr 5 to amplify an error-free MTDN5 gene through the PCR technique. The entire MTND5 gene was screened through merging these two MTND5 (1–3) and MTND5 (3–4) segments. In this process, only the MTND5 gene, rather than the Chr 5 (134926459–134924648) gene, was able to be amplified through the primer. In a case that the primers fail to pair the MTND5 gene during the PCR process, the PCR products could still be distinguished and eliminated since they exhibit different gene length compared to the MTND5 gene.
A previous study has suggested that nuclear mitochondrial pseudogenes (NUMTs) could result in the heteroplasmic mutations (Parr et al. Citation2006). Our amplification method through these primer pairs could ensure the reliable MTND5 PCR products. It should be noted that the total DNA was used for the exome sequencing analysis. Therefore, false positive results could not be avoided in the tissue samples. This was because since the tissue samples contained large amounts of the mtDNA and nDNA and they could yield high SNVs frequencies due to the similarity of the MTND5 and Chr 5 sequences. Additionally, the MTND5 also showed high similarity with the Chr 7, Chr 10, Chr 2, and Chr 4. However, it has been reported that the mtDNA abundance in the tissue samples was much higher than the nDNA, which could cause a higher SNVs frequency in the mtDNA than nDNA (Todorov and Todorov Citation2009). Therefore, the improvement on the sequencing depth could significantly enhance the reliability of the sequencing results.
Mitochondrial copy number effect on SNVs frequency
In the present study, we divided the MTND5 gene into four segments, including the MTND5-1, 2, 3, and 4. Afterwards, each fragment was amplified using the PCR technique. A BLAST search was applied to the MTND5-2 primers and found that the primers exhibited a 100% identity with the MTND5 sequence. The MTND5-2 belongs to a segment of the MTND5 (MT genome 12743–13052), and it showed high identity with the Chr 5 (134926053–134925744). Meanwhile, their amplified products exhibited the same length. The primer ND5-2F has only one nucleotide difference with the Chr 5 (134926053–134926032), and thus annealing temperature could play an important role in avoiding amplification of the Chr 5 during the PCR process. In the present study, we checked the double peak presence in the sequencing analysis. It was found that some different nucleotide sites were specifically identified by the BLAST search between the MTND5-2 and Chr 5 (134926053–134925744). This could help distinguish these genes on the sequencing analysis.
It has been accepted that same amount of the mtDNA and nDNA could produce same amount of the MTND5-2 and NUMTs after the PCR. At the same nucleotide position, the different nucleotides from the MTND5-2 and Chr 5 (134926053–134925744) could produce two peaks on the sequencing analysis. When the amount of the mtDNA in the sample was much greater than the nDNA, the PCR products through the mtDNA template could reach the platform stage with 40 cycles. The NUMTs using the nDNA template could be amplified through 40 cycles during the PCR process. In this study, we used the BGC823 cells as a template, and conducted 40 cycles during the PCR process using the ND5-2F and ND5-2R primers, followed by the PCR products sequencing analysis (Figure S2). It was found that the sequencing result exhibited a 100% similarity to the mtDNA and only one peak was observed in the sequencing analysis. This indicated that the mtDNA accounted for much higher percentage in the total DNA of the BGC823 cells than the nDNA, which resulted in more mtDNA SNVs when the total DNA was used as the template under the exome sequencing approach.
Single nucleotide polymorphisms (SNPs) in gastric cancer paired tissue samples
Single nucleotide polymorphisms are ubiquitous in gastric cancer tissue and normal tissue (McLean and El-Omar Citation2014). Therefore, we applied the PCR to amplify the MTND5 gene from the samples, and then sequenced the PCR products using Sanger sequencing to detect the low frequency SNPs (Table S2 and ). It should be noted that the exome sequencing indicated that the np 12338 was an SNV. In the present study, this np 12338 was also detected. The nucleotide at the same position of the MTND5 gene in the gastric cancer tissue sample 14 and the adjacent normal tissue was cytosine. This indicated that no mutation took place at this nucleotide in the gastric cancer sample 14. It should be worth noting that the nucleotide at the np 12338 of rCRS was thymine, the same nucleotide in all other gastric cancer paired tissue samples (). This indicated that the np 12338 was an SNP in these tissue samples.
Table 2. The result of exome sequencing and sanger sequencing of GC cell lines and paired tissue samples.
Additionally, thymine was found at the np 12705 in five gastric cancer cell lines except for the GES1 and AGS (), whereas the nucleotide at the same position in the rCRS was cytosine. It was found that thymine was detected at the np 12705 in some gastric cancer tissues and normal tissues (sample 1, 2, 6, 8, 9 and 13), the nucleotide at same position in the other paired tissue samples appeared to be cytosine. This indicated that the np 12705 was also an SNP. Similarly, numerous SNPs were identified in the tissue samples using the same analysis, including A12720G in the tissue sample 1, T13263C in the tissue sample 2, A12950G, T13681C and A13269G in the tissue sample 3, A13093G and G13590A in the tissue sample 5, G12372A in the tissue sample 6 and 9, A13269G in the tissue sample 7, A12358G in the tissue sample 9, and T12696C in the tissue sample 14. It should be noted that thymine was the nucleotide at the np 12705 in the cell line BGC823, N87, MGC803, SGC7901, and MKN45, whereas the nucleotide at the np 12372 in the AGS cell line was adenine. Their nucleotide was not as the same as that in the rCRS. However, the 12705 and 12372 could not be determined to be an SNP in these cell lines due to the lack of the control cells.
Single nucleotide variants in gastric cancer tissue and cell lines
A total of seven SNVs were found in three samples out of 13 gastric cancer paired tissue samples, indicating an approximate 23% mutation rate in the present study. Additionally, the mtDNA mutation frequency appeared to be different in these three gastric cancer tissue samples. For example, an SNV was detected in every 453 bp in the tissue sample 16, whereas the tissue sample 14 exhibited an SNV frequency at every 906 bp. Only one SNV was found in the tissue sample 9. It should be noted that six SNVs found in these tissue samples were found to be the SNPs regarding the dbSNP database. However, the nucleotide at the position np 12584 appeared to be a novel SNV in these tissue samples.
It was observed that both thymine and cytosine were present at the np 12882 position in the gastric cancer tissue sample 16 with the similar quantity (). However, the nucleotide at the np 12882 position in the adjacent normal tissue sample 16 was found only to be thymine, whereas cytosine was the only nucleotide at the np 12882 of the rCRS. This indicated that an SNV happed at the np 12882. The T12882C was a heteroplasmic point mutation. Such a mutation could further lead to a synonymous mutation of amino acids.
Meanwhile, the exome sequencing data revealed that the nucleotide at the np 12340 was mutated from adenine to guanine in the gastric cancer tissue samples 210. The nucleotide at the np 12340 position was detected 96 times with adenine 22 times and guanine 74 times, indicating that two different nucleotides at the np 12340 position were found in the gastric cancer tissue samples. The A12340G was also a heteroplasmic point mutation ().
The nucleotide at the np 12406 in two tissue samples (GC208 and GC209) was mutated from guanine to adenine, whereas the GC205 tissue sample had the nucleotide at this position was guanine instead of adenine. In the gastric cancer 208 normal tissue sample, the nucleotide at the np 12406 position was detected 76 times with adenine 12 times and guanine 64 times. The sanger sequencing analysis indicated that two peaks (guanine and adenine) were observed at the np 12406 in the gastric cancer tissue sample 16 (). However, only adenine peak was found at the np 12406 position in the normal tissue sample 16. These indicated that adenine in the gastric cancer tissue sample was mutated from guanine at the np 12406 in some mtDNA, which could further result in the mutation of amino acid formation from isoleucine to valine. In the gastric cancer cell lines, adenine and thymine were found at the np 12406 and 12882 position in the gastric cancer cell line GES1, respectively.
In the gastric cancer sample 16, double peaks were found at the np 12705 position representing thymine and cytosine (). However, cytosine was the only nucleotide found at this position in the normal tissue sample 16. This indicated that the mtDNA might be partially mutated from cytosine to thymine at this position in the gastric cancer tissue 16, since those double peaks at the np 12705 were similar as the SNVs at the np 12406 and 12882. This also suggested that the nucleotide at the np 12705 turned out to be an SNV in the gastric cancer paired tissue sample 16, although it was an SNP in other tissues and previously published reports (Mosquera-Miguel et al. Citation2009; Ren et al. Citation2015). Similarly, the nucleotide at the np 13708 has been reported to be an SNP (van der Walt et al. Citation2003; Mosquera-Miguel et al. Citation2009). In the exome sequencing analysis, guanine and adenine were found at this position for 11 and 13 times in the normal tissue samples. However, the nucleotide at the np 13708 was found to be adenine in the gastric cancer tissue sample. This indicated that the nucleotide at the np 13708 appeared to be an SNV in the exome sequencing analysis.
It should be worth noting that some SNVs were not identified using the exosome sequencing analysis. However, they were detected via the PCR and Sanger sequencing analyses in the present study. For example, the nucleotide at the np 12584 position in the gastric cancer tissue sample 9 consisted of adenine and guanine, whereas adenine appeared to be the nucleotide at this position in the adjacent normal tissue sample. These indicated that some mtDNA in the gastric cancer tissue sample 9 was mutated from adenine to guanine, which further resulted in an alteration on amino acid synthesis from aspartic acid to glycine. Similarly, the gastric cancer tissue sample 16 exhibited guanine and adenine at the np 12501 position, whereas the nucleotide at the same position in the adjacent normal tissue sample was guanine. This caused a synonymous mutation in the gastric cancer tissue sample 16.
A homoplasmic point mutation was found at the np 13590 position in comparison of the gastric cancer tissue sample 13 with its adjacent normal tissue sample. Particularly, the nucleotide at this position in the normal tissue sample was guanine, whereas it was mutated to adenine in the gastric cancer tissue sample 13. Such a mutation could further lead to a synonymous mutation on amino acids. It should be noted that adenine was the only nucleotide at the np 13590 position in the gastric cancer tissue sample 5 and its adjacent normal tissue sample (), whereas the nucleotide at the same position in the other paired tissue samples was guanine. These indicated that the nucleotide at the np 13590 in the cancer tissue sample 13 was an SNV but it was an SNP in the other tissue samples. Additionally, the nucleotide at the np 12561 also turned to be a homoplasmic point mutation. This was because adenine and guanine were found to be the nucleotide at this position in the gastric cancer tissue sample 13 and its adjacent tissue sample, respectively ().
In the gastric cancer cell lines, two heteroplasmic point mutations were also found, including the C12474T in the AGS cell and the G12835A in the BGC823 cell. The mutation of G12835A resulted in the amino acid formation from alanine to threonine (). In addition, the comparison on the sequence of these gastric cancer cell lines with the rCRS exhibited the differences in seven nucleotides. For example, the nucleotide at the np 13759 and 13928 were adenine and cytosine in the GES1 cell line, whereas the difference in the N87 cell line was observed in adenine at the np 12771, cytosine at the np 13830, and guanine at the np 13105. The nucleotide at the np 13914 and 13105 in the BGC823 cell line appeared to be adenine and guanine, respectively.
Discussion
We hypothesized that gastric cancer incidence might result from the mtDNA mutation, and such mtDNA mutations might be caused by mitochondrial SNVs in gastric cancer tissue. After analysing paired gastric cancer tissue samples and gastric cancer cell lines using a combined approach of the PCR and Sanger sequencing analyses, nine MTND5 SNVs and some SNPs were detected. This included seven MTND5 SNVs found in the 13-paired gastric cancer tissue samples and two SNVs in the gastric cancer cell lines. More importantly, the point mutation frequency appeared to be different in paired gastric cancer tissue samples from different patients. For example, the SNV at the position 12406, 12705, 12882, and 12501 was found in the gastric cancer tissue sample 16 compared to its adjacent normal tissue sample using the PCR technique. No SNVs and SNPs were observed in the gastric cancer tissue sample 4. Besides, we also found that the nucleotide at the position 12406, 12705, 12501, 12882, 12561, and 13590 appeared to be SNVs in the gastric cancer tissue and cell line samples of the present study although they have been classified as the SNPs in the dbSNP database. It has been reported that SNPs could also trigger the carcinogenesis (Wang et al. Citation2007; Grzybowska-Szatkowska and Ślaska Citation2014; Williams Stephen et al. Citation2015; Bussard and Siracusa Citation2017). For instance, SNPs G9055A, A10398G, and T16519C have been reported to increase the breast cancer risk (Bai et al. Citation2007). It has also been suggested that SNP 10398G played an inherited predisposition factor in developing the breast cancer (Czarnecka et al. Citation2010). Our results were in an accordance with these studies.
Exome sequencing analysis could determine the nucleotide at the specific position of a mutation. It should be noted that a nucleotide with the high frequency could be considered the mutated nucleotide in the site, whereas the low frequency nucleotide could be ignored when a site contains two nucleotides. The present study could indicate that the same tissue or cell could contain two different types of mtDNA, which was consistent with the previous study (Ye et al. Citation2014). Furthermore, we proposed that the mtDNA point mutations in the present study might result from a process where a normal mtDNA was gradually mutated by a mutated mtDNA in cells/tissues. When the mtDNA mutations reached to a certain level, the mutated cells might become cancerous (McCann et al. Citation2015; Stefano and Kream Citation2016).
Conclusions
In conclusion, NUMTs and mitochondrial copy number exerted an important role in affecting the detection of SNV frequency in gastric cancer tissue and cell line samples using high-throughput sequencing technique. The MTND5 point mutation frequency appeared to be different in different gastric cancer tissue and cell line samples. Heteroplasmic point mutation C12474T and G12835A were found in AGS and BGC823 cell lines, respectively. A total of seven SNVs were observed in three paired gastric cancer tissue samples, including five heteroplasmic point mutations (A12406G, C12705T, T12882C, G12501A, and A12584G) and two homoplasmic point mutations (G12561A and C13590T).
Abbreviations | ||
MT-ND5 | = | NADH dehydrogenase subunit 5 of mitochondrial DNA |
SNP | = | Single nucleotide polymorphism |
SNV | = | Single nucleotide variant |
NUMTs | = | Nuclear mitochondrial pseudogenes |
BLAST | = | Basic local alignment search tool |
rCRS | = | Revised Cambridge reference sequence |
PCR | = | Polymerase chain reaction |
np | = | Nucleotide position |
ROS | = | Reactive oxygen species |
Acknowledgments
The authors were grateful to the Beijing Genomics Institute for providing the data of exome capture sequencing.
Disclosure statement
The authors declare that they have no competing interests.
Additional information
Funding
References
- Anderson S, Bankier AT, Barrell BG, de Bruijn MH, Coulson AR, Drouin J, Eperon IC, Nierlich DP, Roe BA, Sanger F, et al. 1981. Sequence and organization of the human mitochondrial genome. Nature. 290:457–465.
- Andrews RM, Kubacka I, Chinnery PF, Lightowlers RN, Turnbull DM, Howell N. 1999. Reanalysis and revision of the Cambridge reference sequence for human mitochondrial DNA. Nat Genet. 23:147.
- Bai RK, Leal SM, Covarrubias D, Liu A, Wong LJ. 2007. Risk mitochondrial genetic background modifies breast cancer. Cancer Res. 67:4687–4694.
- Bussard KM, Siracusa LD. 2017. Understanding mitochondrial polymorphisms in cancer. Cancer Res. 77:6051–6059.
- Chatterjee A, Mambo E, Sidransky D. 2006. Mitochondrial DNA mutations in human cancer. Oncogene. 25:4663–4674.
- Chen WQ, Zheng RS, Zhang SW, Li N, Zhao P, Li GL, Wu LY, He J. 2013. Report of incidence and mortality in china cancer registries,2009. Chin J Cancer Res. 25:10–21.
- Connolly BS, Feigenbaum AS, Robinson BH, Dipchand AI, Simon DK, Tarnopolsky MA. 2010. MELAS syndrome, cardiomyopathy, rhabdomyolysis, and autism associated with the A3260G mitochondrial DNA mutation. Biochem Biophys Res Commun. 402:443–447.
- Coxhead J, Kurzawa-Akanbi M, Hussain R, Pyle A, Chinnery P, Hudson G. 2016. Somatic mtDNA variation is an important component of Parkinson’s disease. Neurobiol Aging. 38:217.e1–e217.e6.
- Croteau DL, Bohr VA. 1997. Repair of oxidative damage to nuclear and mitochondrial DNA in mammalian cells. J Biol Chem. 272:25409–25412.
- Czarnecka AM, Krawczyk T, Zdrozny M, Lubiński J, Arnold RS, Kukwa W, Scińska A, Golik P, Bartnik E, Petros JA. 2010. Mitochondrial NADH-dehydrogenase subunit 3 (ND3) polymorphism (A10398G) and sporadic breast cancer in Poland. Breast Cancer Res Treatment. 121:511–518.
- Deans C, Yeo MS, Soe MY, Shabbir A, Ti TK, So JB. 2011. Cancer of the gastric cardia is rising in incidence in an Asian population and is associated with adverse outcome. World J Surg. 35:617–624.
- Dhillon VS, Fenech M. 2014. Mutations that affect mitochondrial functions and their association with neurodegenerative diseases. Mutat Res Rev Mutat Res. 759:1–13.
- Eckenweiler M, Catarino CB, Gallenmueller C, Klopstock T, Lagrèze WA, Korinthenberg R, Kirschner J. 2015. Mitochondrial DNA mutation 14487T > C manifesting as Leber's hereditary optic neuropathy. J Neurol. 262:2776–2779.
- Galluzzi L, Kepp O, Trojel-Hansen C, Kroemer G. 2012. Mitochondrial control of cellular life, stress, and death. Circ Res. 111:1198–1207.
- Grzybowska-Szatkowska L, Ślaska B. 2014. Mitochondrial NADH dehydrogenase polymorphisms are associated with breast cancer in Poland. J Appl Genet. 55:173–181.
- Gu SY, Chen CZ, Jiang XJ, Zhang ZZ. 2016. ROS-mediated endoplasmic reticulum stress and mitochondrial dysfunction underlie apoptosis induced by resveratrol and arsenic trioxide in A549 cells. Chem-Biol Interact. 245:100–109.
- Gupta M, Madkaikar M, Rao VB, Mishra A, Govindaraj P, Thangaraj K, Ghosh K. 2013. Mitochondrial DNA variations in myelodysplastic syndrome. Ann Hematol. 92:871–876.
- Holzerová E, Prokisch H. 2015. Mitochondria: much ado about nothing? How dangerous is reactive oxygen species production? Int J Biochem Cell Biol. 63:16–20.
- Kim H, Komiyama T, Inomoto C, Kamiguchi H, Kajiwara H, Kobayashi H, Nakamura N, Terachi T. 2016. Mutations in the mitochondrial ND1 gene are associated with postoperative prognosis of localized renal cell carcinoma. IJMS. 17:2049.
- Kim SH, Kim KY, Park SG, Yu SN, Kim YW, Nam HW, An HH, Kim YW, Ahn SC. 2017. Mitochondrial ROS activates ERK/autophagy pathway as a protected mechanism against deoxypodophyllotoxin-induced apoptosis. Oncotarget. 8:111581–111596.
- Kytövuori L, Lipponen J, Rusanen H, Komulainen T, Martikainen MH, Majamaa K. 2016. A novel mutation m.8561C > G in MT-ATP6/8 causing a mitochondrial syndrome with ataxia, peripheral neuropathy, diabetes mellitus, and hypergonadotropic hypogonadism. J Neurol. 263:2188–2195.
- Lightowlers RN, Taylor RW, Turnbul DM. 2015. Mutations causing mitochondrial disease: what is new and what challenges remain? Science. 349:1494–1499.
- Márquez-Jurado S, Díaz-Colunga J, das Neves RP, Martinez-Lorente A, Almazán F, Guantes R, Iborra FJ. 2018. Mitochondrial levels determine variability in cell death by modulating apoptotic gene expression. Nat Commun. 9:389.
- McCann BJ, Tuppen HAL, Küsters B, Lammens M, Smeitink JAM, Taylor RW, Rodenburg RJ, Wortmann SB. 2015. A novel mitochondrial DNA m.7507A > G mutation is only pathogenic at high levels of heteroplasmy. Neuromuscul Disord. 25:262–267.
- McLean MH, El-Omar EM. 2014. Genetics of gastric cancer. Nat Rev Gastroenterol Hepatol. 11:664–674.
- Montiel-Sosa JF, Herrero MD, Munoz MdL, Aguirre-Campa LE, Pérez-Ramírez G, García-Ramírez R, Ruiz-Pesini E, Montoya J. 2013. Phylogenetic analysis of mitochondrial DNA in a patient with Kearns–Sayre syndrome containing a novel 7629-bp deletion. Mitochondr DNA. 24:420–431.
- Mosquera-Miguel A, Álvarez-Iglesias V, Cerezo M, Lareu MV, Carracedo Á, Salas A. 2009. Testing the performance of mtSNP minisequencing in forensic samples. Forensic Sci Int Genet. 3:261–264.
- Parr RL, Maki J, Reguly B, Dakubo GD, Aguirre A, Wittock R, Robinson K, Jakupciak JP, Thayer RE. 2006. The pseudo-mitochondrial genome influences mistakes in heteroplasmy interpretation. BMC Genomics. 7:185.
- Ren Z, Luo HB, Song F, Wei W, Yang YY, Zhai XD, Chen XG, Hou YP. 2015. Developing a multiplex mtSNP assay for forensic application in Han Chinese based on mtDNA phylogeny and hotspot. Electrophoresis. 36:633–639.
- Sena LA, Chandel NS. 2012. Physiological roles of mitochondrial reactive oxygen species. Mol Cell. 48:158–167.
- Stefano GB, Kream RM. 2016. Mitochondrial DNA heteroplasmy in human health and disease. Biomed Rep. 4:259–262.
- Thun MJ, DeLancey JO, Center MM, Jemal A, Ward EM. 2010. The global burden of cancer: priorities for prevention. Carcinogenesis. 31:100–110.
- Todorov IN, Todorov GI. 2009. Multifactorial nature of high frequency of mitochondrial DNA mutations in somatic mammalian cells. Biochemistry (Mosc). 74:962–970.
- van der Walt JM, Nicodemus KK, Martin ER, Scott WK, Nance MA, Watts RL, Hubble JP, Haines JL, Koller WC, Lyons K, et al. 2003. Mitochondrial polymorphisms significantly reduce the risk of Parkinson disease. Am J Hum Genet. 72:804–811.
- Wallace DC. 2012. Mitochondria and cancer. Nat Rev Cancer. 12:685–698.
- Wang L, Bamlet WR, de Andrade M, Boardman LA, Cunningham JM, Thibodeau SN, Petersen GM. 2007. Mitochondrial genetic polymorphisms and pancreatic cancer risk. Cancer Epidemiol Biomarkers Prev. 16:1455–1459.
- Williams Stephen B, Ye YQ, Huang MS, Chang DW, Kamat AM, Pu X, Dinney CP, Wu X. 2015. Mitochondrial DNA content as risk factor for bladder cancer and its association with mitochondrial DNA polymorphisms. Cancer Prev Res (Phila). 8:607–613.
- Xu B, Li X, Du M, Zhou C, Fang H, Lyu J, Yang Y. 2017. Novel mutation of ND4 gene identified by targeted next-generation sequencing in patient with Leigh syndrome. J Hum Genet. 62:291–297.
- Ye KX, Lu J, Ma F, Keinan A, Gu ZL. 2014. Extensive pathogenicity of mitochondrial heteroplasmy in healthy human individuals. PNAS. 111:10654–10659.
- Zamzami N, Kroemer G. 2001. The mitochondrion in apoptosis: how Pandora's box opens. Nat Rev Mol Cell Biol. 2:67–71.