Abstract
Oyster reefs have important ecological functions and environmental service values, such as filtering water, providing fish habitats, maintaining biodiversity, and carbon sequestration. Oyster reef recovery has become one of the most important means for fishery resource conservation and ecosystems restoration of coastal regions. C. sikamea and C. ariakensis are two important reef oysters along the coast of China. However, it is very difficult to identify the larvae of these two oysters. To solve this problem, we developed two pairs of novel species-specific PCR primers for identifying these two oysters. The forward C. sikamea-specific primer is Cs-F: 5′-CGAAGAGGGGCATGATAAATGAGG-3′, and the reverse primer is Cs-R: 5′-ATATGAACTTCTCCAACCTCCCC-3′, both of them within the scope of mitochondrial ND5 gene. The forward C. ariakensis-specific primer is Ca-F: 5′-GGGCAAATAAAAGGCAAAACCC-3′, located between tRNAser and tRNApro, and the reverse primer is Ca-R: 5′-CATAAACTTCTGCAAGACTCCC-3′, lies between tRNApro and small subunit ribosomal RNA (ssrRNA). These two primers can identify the larvae of C. ariakensis and C. sikamea from other oysters rapidly and effectively, with extremely high accurate rate (=100%). This molecular identification method does not require prior sorting of larvae. These two specific primers are good contribution to molecular identification of C. sikamea, C. ariakensis and other oysters, and could be a useful tool for oyster larvae identification and ecological restoration.
Oysters (Ostreidae, Pterioida) are widely distributed in temperate estuaries and coastal areas (Beck et al. 2011). Oysters are economically important species and aquaculture shell-fishes with the largest products in the world. More than 100 species are found in the world and about 20 species in China (Wang and Wang Citation2008). Oyster reefs also have important ecological functions and environmental service values, such as filtering water (Nelson et al. Citation2004; Quan et al. Citation2007), providing fish habitats (Coen et al. Citation1999; Quan et al. Citation2009, Citation2012, Citation2013), maintaining biodiversity (Quan et al. Citation2006, Citation2011, Citation2013; Rodney and Paynter, Citation2006), and carbon sequestration (Shen et al. Citation2011). Oysters are ‘keystone species’ of the oyster reef ecosystem. Among all the oysters distributed globally, six species, i.e. C. sikamea (Quan et al. Citation2012) , C. ariakensis (Quan et al. Citation2009, Citation2011), C. gigas (Lejart and Hily. Citation2011), C. virginica (Boudreaux et al. Citation2006), Ostrea lurida (Brumbaugh and Coen. Citation2009), O. edulis (Smyth and Roberts. Citation2010), are reported as reef-building species. In China, five oysters, i.e. C. sikamea, C. ariakensis, C. gigas, C. hongkongensis, and C. angulata are common species and two of them, i.e. C.sikamea and C.ariakensis, are reef-forming species.
Identifying the reef-forming species accurately is an important pre-requisite for analyzing the spatial niche and reef-building ability of oysters, inspecting oyster competition for adhesion substrates space use, and colonizing ability. Due to the diverse habitat and shell plasticity, external morphological characteristics of oysters often changes greatly with the different living environment. Distinguishing them relying only on shell exterior appearance is difficult.
The life cycle of oysters consist of a larval phase (prior to settlement) and adult phase (after settlement). Once settled on the required hard substrate, adults cannot relocate (Coen and Humphries Citation2017). Based on successful recruitment of oyster larvae and later larvae in successive years, oyster reefs can continue to expand (Coen and Luckenbach Citation2000; Luckenbach et al. Citation2005). Reliably detecting larvae during critical life stages is urgent to forecast population recruitment.
Larvae cannot be reliably identified using conventional morphologic observations similar to the larvae of most marine organisms (Christo et al. Citation2010). This method is problematic during earlier stages of larval development and relies on proficient expertise on bivalve larvae identification. Although traditional microscopic screening based on morphology is possible, it is time-consuming, laborious, and need skilled expertise (Boudry et al. Citation2003).
Molecular probes and markers are widely used for the identification of oyster species in the last decades (Morgan and Rogers 2001; Anil et al. Citation2002). For example, using molecular markers, Anderson and Adlard (Citation1994) and Kenchington et al. (Citation2002) classified the oyster populations described in different geographical regions into one species, Hedgecock et al. (Citation1999) verified a species’ presence in a geographic area, and various Crassostrea oysters species were distinguished (Banks et al. Citation1993, O’Foighil et al. Citation1995, Klinbunga et al. Citation2000, Citation2001, Citation2003, Boudry et al. Citation2003). In order to solve difficulty in the identification of larvae oysters, especially the larvae of two main reef-forming oysters, i.e. C. sikamea and C. ariakensis, in China, we developed two pairs of species-specific primers. These two pairs of primers will be widely used for the identification of the larvae of C. sikamea and C. ariakensis, and can also be helpful to improve the detection level of period of larval release and settlement in complex environmental and water samples.
Adult individuals of five oysters species including C. sikamea, C. ariakensis, C. gigas, C. angulata, and C. hongkongensis, were collected from the intertidal and coastal zones of China, i.e Dalian(DL), Weihai(WH), Nantong(NT), Shanghai(SH), Ningbo(NB), Ningde(ND), and Yangjiang(YJ). The adductor tissues of these individuals were stored at −20 °C until used. C. sikamea and C. ariakensis larvae were collected from Liyashan, Luchao, and Xiangshan, using a 200-mesh sieve. They were preserved in 95% ethanol fixative and stored at 4 °C until extracted. All specimens were deposited in East China Sea Fisheries Research Institute.
Genomic DNA of adult adductor muscles (about 20mg) were extracted using the TIAN & Marine Animal DNA kit (Tiangen). The product contents of this DNA extraction kitinclude Buffer GA, Buffer GB, Buffer GD, Buffer PW, Buffer PW, Buffer PW, Buffer PW, ProteinaseK, and adsorption Column CB3 (Spin Column CB3), CollectionTube 2ml. Larvae samples were concentrated by vacuum filtration through a 0.45 micron nitrocellulose filter or isolated under an Optical microscope were transferred directly to 1.5 ml PCR tubes immersed in ethanol, and DNA-extracted using the Marine animal tissue genomic DNA extraction kit (Qiagen) following the suppliers instructions. DNA was retrieved in 100 μl of elution buffer and stored at 4 °C.
Amplification and sequencing of the 16SrDNA markers was carried out using the universal primers 16SAR/BR. Approximately 400–600bp amplicons correspond to mitochondrial genome base sites between 1865 and 2365 of C. sikamea (accession number FJ841966.1) and from 1870 to 2359 of C. ariakensis (accession number FJ841964.1). Standard PCR reactions were performed using a 25 μL volume of PCR mixture, containing DNA Template 1 μL, 1 μL of each primer, 2 × Master Mix 12.5μL (0.1U/μL Taq DNA polymerase; 4mm MgCl2; 0.4 mM dNTPs), and ddH2O 9.5 μL. PCR amplifications were performed under the following conditions: initial pre-denaturation at 94 °C for 5 min followed by 35 thermal cycles of denaturation at 94 °C for 30 s, annealing at 52 °C for 45 s, and extension at 72 °C for 1 min. A final extension was performed at 72 °C for 7 min.
Two pairs of primers were designed by comparing complete mitochondrial sequences of C. sikamea and C. ariakensis with other members of the family Crassostrea including those of C. gigas, C. hongkongensis, and C. angulata. The forward C. sikamea-specific primer is Cs-F: 5′-CGAAGAGGGGCATGATAAATGAGG-3′, and the reverse primer is Cs-R: 5′-ATATGAACTTCTCCAACCTCCCC-3′, both of them within the scope of mitochondrial ND5 gene. The forward C. ariakensis-specific primer is Ca-F: 5′-GGGCAAATAAAAGGCAAAACCC-3′, located between tRNAser and tRNApro, and the reverse primer is Ca-R: 5′-CATAAACTTCTGCAAGACTCCC-3′, lies between tRNApro and small subunit ribosomal RNA (ssrRNA).
The PCR reaction components and amplification conditions of these two specific primers are the same. PCR reactions were performed using a 20 μl volume of PCR mixture containing 1 μl of each primer, 2 × Master Mix 10 μl (0.1 units/μl Taq DAN polymerase; 4m MMgCl2; 0.4 mM dNTPs), ddH20 7.5 μl, and Template 0.5 μl. PCR amplifications were performed under the following conditions: initial pre-denaturation at 95 °C for 3 min followed by 35 thermal cycles of denaturation at 95 °C for 15 s, annealing at 55 °C for 15 s, and extension at 72 °C for 15 s. A final extension was performed at 72 °C for 3 min. In order to control the contamination, high-temperature sterilization is required for each pipette tips with all PCR solutions, and negative control reactions were performed with sterilized water and other oyster species. Verification of the species-specific PCR results of C. sikamea and C.ariakensis were undertaken with a DNA marker DL500 using 2μL of each PCR product electrophoresed separately in 3% agarose gel containing ethidium bromide.
The specificity of the predicted C. sikamea-specific primers and C. ariakensis-specific primers was empirically tested by PCR amplification of genomic DNA of the five Crassostrea species using sterile water as negative control. For C. sikamea specific primers, the expected 114 bp amplicon can only be amplified from C. sikamea individuals, while the other four oysters and sterile water had no amplicons and only C. ariakensis can obtain the expected 126 bp target fragment ().
Figure 1 The agarose gel electrophoresis image of five oysters PCR products. Notes: (a) from left to right: lane 1, DL500 marker; lanes 2–5, C. sikamea; lanes 6–9, C.ariakensis; lanes 10–13, C. angulata; lanes 14–17, C. gigas; lanes 18–21, C. hongkongensis; lanes 22–24, sterile water (negative control); (b) from left to right: lane 1, DL500 marker; lanes 2–5, C.ariakensis; lanes 6–9, C. sikamea; lanes 10–13, C. gigas; lanes 14–17, C. angulata; lanes 18–21, C. hongkongensis; lanes 22–24, sterile water (negative control).
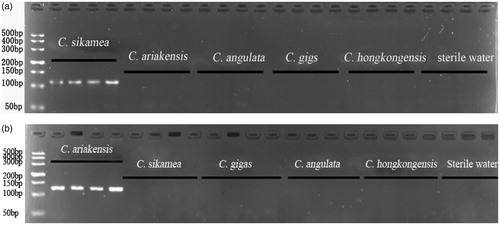
The PCR product was sequenced by Sanger’s dideoxy chain termination method and then compared with Vector NTI software. The results indicate that the forward C. sikamea-specific primer Cs-F and the reverse primer Cs-R are both within the scope of mitochondrial ND5 gene, but the forward C. ariakensis-specific primer Ca-F is located between tRNAser and tRNApro and the reverse primer Ca-R lies between tRNApro and small subunit ribosomal RNA(ssrRNA).
Larvae sample testing: It was possible to amplify the isolated larvae as templates by using C. sikamea-specific primers and C. ariakensis-specific primers. Detection accuracy was 100% when larvae or spat were used as templates (). Identifying oyster larvae rapidly and accurately is of great importance for the seashore ecological management because it is not only useful to judge natural distribution of the larval across the areas of the sea, settlement, and recruiting commercial fisheries, but also to monitor the presence of other new oysters in the native ecosystem (Bax et al. 2005). Above all, identifying C. sikamea and C. ariakensis larvae in China is particularly important because it can provide scientific guidance for further analysis about the presence/absence and spatial niche of these two oysters distributed in water body, evaluating reef-forming ability of oysters, and monitoring the ecological status of seashore.
Disclosure statement
No potential conflict of interest was reported by the authors.
Additional information
Funding
References
- Anderson TJ, Adlard RD. 1994. Nucleotide-sequence of a rDNA internal transcribed spacer supports synonym of Saccostrea commercialis and S. glomerat. J Mollus Stud. 60:196–197.
- Anil AC, Venkat K, Sawant SS, Dileepkumar M. 2002. Ansari marine bio-invasion: concern for ecology and shipping. Curr Sci India. 83:214–218.
- Banks MA, Hedgecock D, Waters C. 1993. Discrimination between closely related pacific oyster species (Crassostrea) via mitochondrial DNA sequences coding for large subunit rRNA. Mol Mar Biol Biotechno. 12:129–136.
- Bax NJ. 2005. Specific detection of Pacific oyster (Crassostreagigas) larvae in plankton samples using nested polymerase chain reaction. J Marine Biotechnology. 7(1):11–20.
- Beck MW, Brumbaugh RD, Airoldi L, et al. 2011. Oyster reefs at risk and recommendations forconservation, restoration and management. BioScience. 61:107–116.
- Boudreaux ML, Stiner JL, Walters LJ. 2006. Biodiversity of sessile and motile macrofauna on intertidal oyster reefs in Mosquito lagoon, Florida. J Shellfish Res. 25:107–1089.
- Boudry P, Heurtebise S, Lapegue S. 2003. Mitochondrial and nuclear DNA sequence variation of presumed Crassostrea gigas and Crassostrea angulata specimens: a new oyster species in Hong Kong? Aquaculture. 228:15–25.
- Brumbaugh RD, Coen LD. 2009. Contemporary approaches for small-scale oyster reef restoration to address substrate versus recruitment limitation: a review and comments relevant for the Olympia oyster, Ostrea lurida (Carpenter, 1864). J Shellfish Res. 28:147–161.
- Christo SW, Absher TM, Boehs G. 2010. Morphology of the larval shell of three oyster species of the genus Crassostrea SACCO,1897 (Bivalvia: Ostreidae). Braz. J. Biol. 70:645.
- Coen LD, Luckenbach MW. 2000. Developing success criteria and goals for evaluating oyster reef restoration: ecological function or resource exploitation? Ecol Eng. 15:323–650.
- Coen LD, Luckenbach MW, Breitburg DL. 1999. The role of oyster reefs as essential fish habitat: a review of current knowledge and some new perspectives. In: Benaka L R (ed.). Fish habitat: essential fish habitat and rehabilitation. American Fisheries Society, Symposium 22, Bethesda, M D. p.438–454.
- Coen LD, Humphries A. 2017. Routledge Handbook of Ecological and Environmental Restoration. Taylor and Francis Group, Routledge, Cambridge, U.K. Oyster-generated marine habitats: their services, enhancement, restoration, and monitoring.
- Coen LD, Luckenbach MW, Breitburg DL, 1999. The role of oyster reefs as essential fishhabitat: a review of current knowledge and some new perspectives. In: Benaka LR, editor. Fish habitat: essential fish habitat and rehabilitation. Symposium. 22, Bethesda, MD;American Fisheries Society; p. 438–454.
- Hedgecock D, Li G, Banks MA, Kain Z. 1999. Occurrence of the Kumamoto oyster Crassostrea sikamea in the Ariake Sea, Japan. Mar Biol. 133:65–68.
- Kenchington E, Bird CJ, Osborne J, Reith M. 2002. Novel repeat elements in the nuclear ribosomal RNA operon of the flat oyster Ostrea edulis C. Linneaus, 1758 and O-angasi Sowerby, 1871. J Shellfish Res. 21:697–705.
- Klinbunga S, Ampayup P, Tassanakajon A, Jarayabhand P, Yoosukh W. 2001. Genetic diversity and molecular markers of cupped oysters (Genera Crassostrea, Caccostrea, and Striostrea) in Thailand revealed by randomly amplified polymorphic DNA analysis. Mar Biotechnol. 3:133–144.
- Klinbunga S, Khamnamtong N, Tassanakajon A, Puanglarp N, Jarayabhand P, Yoosukh W. 2003. Molecular genetic identification tools for three commercially cultured oysters (Crassostrea belcheri, Crassostrea iredalei, and Saccostrea cucullata) in Thailand. Mar Biotechnol. 5:27–36.
- Klinbunga S, Ampayup P, Tassanakajon A, Jarayabhand P, Yoosukh W. 2000. Development of species-specific markers of the tropical oyster (Crassostrea belcheri) in Thailand. Mar Biotechno. 12:476–484.
- Lejart M, Hily C. 2011. Differential response of benthic macrofauna to the formation of novel oyster reefs (Crassostrea gigas, Thunberg) on soft and rocky substrate in the intertidal of the Bay of Brest. France. J Sea Res. 65:84–93.
- Luckenbach MW, Coen LD, Ross PG, Stephen JA. Jr., 2005. Oyster reef habitat restoration: relationships between oyster abundance and community development based on two studies in Virginia and South Carolina. J. Coastal Res Special Issue. 40:64–78.
- Morgan TS, Rogers AD 2001. Specificity and sensitivity of microsatellite markers for the identification of larvae. Mar Biol. 139:967–973.
- Nelson KA, Leonard LA, Posey MH, Alphin TD, Mallin MA. 2004. Using transplanted oyster (Crassostrea virginica) beds to improve water quality in small tidal creeks: a pilot study. J Exp Mar Bio Ecol. 298:347–368.
- Ó Foighil D, Gaffney PM, Hilbish TJ. 1995. Differences in mitochondrial16S ribosomal gene sequences allow discrimination among American [Crassostrea virginica (Gmelin)] and Asian [C. gigas (Thunberg) C. ariakensis Wakiya] oyster species. J Exp Mar Biol Ecol. 192:211–220.
- Quan WM, Shen XQ, Luo MB. 2006. Ecological functions and restoration measures of oyster reef in estuaries. Ecology. 25:1234–1239.
- Quan WM, An CG, Ma CY, et al. 2012. Diversity and community structure of macrozoobenthos in Hong oyster reef of Su-xiao Temple. Marine and Lake Marsh. 43:992–999.
- Quan W-m, Humphries AT, Shi L-y, Chen Y-q. 2011. Determination of trophic transfer at a created intertidal oyster (Crassostrea ariakensis) reef in the Yangtze River estuary using stable isotope analyses. Estuar Coast. 35:109–120.
- Quan WM, Zhang JP, Ping XY, et al. 2007. Purification function and ecological service value of Crassostrea sp. in Yangtze Revier estuary. Chin J Appl Ecol. 18:871–876.
- Quan W, Zheng L, Li B, An C. 2013. Habitat values for artificial oyster (Crassostrea ariakensis) reefs compared with natural shallow-water habitats in Changjiang River estuary. Chin J Oceanol Limnol. 31:957–969.
- Quan W-m, Zhu J-x, Ni Y, Shi L-y, Chen Y-q. 2009. Faunal utilization of constructed intertidal oyster (Crassostrea rivularis) reef in the Yangtze River estuary, China. Ecol Eng. 35:1466–1475.
- Rodney WS, Paynter KT. 2006. Comparisons of macrofaunal assemblages on restored and non-restored oyster reefs in mesohaline regions of Chesapeake Bay in Maryland. J Exp Mar Biol. 335:39–51.
- Shen XQ, Quan WM, Yuan Q. 2011. Restoration and assessment of carbon sink potential for Aintertidal oyster reef in the Yangtze River Estuary, China. J Agro-Environ Sci. 30:2119–2123.
- Smyth D, Roberts D. 2010. The European oyster (Ostrea edulis) and its epibiotic succession. Hydrobiologia. 655:25–36.
- Wang RC, Wang ZP. 2008. Science of marine shellfish culture. China Ocean University Press, Qingdao.