Abstract
Paralvinella hessleri is a terrebellomorphy polychaetae worm lives on the hydrothermal vents in the Okinawa trough and Mariana Back-Arc basin. We report the complete mitochondrial genome of P. hessleri, which is 16,978 bp in length, containing 13 protein-coding genes, two ribosomal proteins, and 22 tRNAs. The gene order is identical to that of reported terebellids. The overall base composition is AT-biased. Our results of P. hessleri mt genome may provide useful genetic information for phylogenetic and population connectivity studies of the deep-sea alvinellid worms.
The Alvinellids are deep-sea terrebellomorphy polychaete worms that are endemic to hydrothermal vent habitats (Desbruyeres and Laubier Citation1991; Juniper and Martineu Citation1995). They commonly inhabit the hot spring fields of the hydrothermal vent, and therefore, are able to tolerate high concentration of toxic heavy metals, sulfide, carbon dioxide, and harmful compounds (Desbruyeres et al. Citation1998; Sicot et al. Citation2000; Mary et al. Citation2010). The Paralvinella hessleri is an East Pacific alvinellid worm, which could be commonly found in Okinawa trough and Mariana back-arc basin (Desbruyeres and Laubier Citation1989). Similar to the famous ‘Pompeii worm’ Alvinella pompeijna and the ‘Sulfur worm’ Paralvinilla sulfincola, P. hessleri also colonizes mid to high temperature hydrothermal vent. It is considered to be among the most thermotolerant metazoan (Shigeno et al. Citation2014). However, the molecular information of the P. hessleri (as well as most other alvinellid worms) is still extremely rare. Therefore, little is known about its phylogenetic relationship with other alvinellids or population connectivity. Here, we report the mitogenome of P. hessleri and explored its phylogenetic position
The specimens of P. hessleri was collected from a hydrothermal vent in the JADE hydrothermal field located at the Okinawa trough, using the remotely operated vehicle (ROV) Faxian in September of 2016. The animals were snap frozen by liquid nitrogen and preserved on board −80 °C freezer, then transferred to sample archive of Centre of Deep-Sea Research, Institute of Oceanology, CAS. Total genomic DNA of the worm was extracted using the E.Z.N.A. Mollusc DNA Kit (OMEGA Bio-Tek, Norcross, GA, USA) and then sequenced on an Illumina Hiseq (2X150bp Pair-end reads, San Diego, USA). Approximately, 50 Gb sequence data were generated. The mitogenome of P. hessleri was de novo assembled by using SPAdes v3.12.0 (Bankevich et al. Citation2012). Gene annotation was performed with MITOS web server (Bernt et al. Citation2013). The sequence has been deposited in GenBank under accession number MK192098. A maximum likelihood (ML) tree was constructed using the IQ-TREE web service (Nguyen et al. Citation2015; Trifinopoulos et al. Citation2016).
The circular mitogenome of P. hessleri is 16,978 bp in size, with an overall base composition of 30.0% for A, 25.3% for C, 15.1% for G, and 29.6% for T. The overall GC content of the mitogenome is 40.4%. The genome exhibits codon biases, with an AT content of about 59.9% for protein-coding genes. The mitochondrion genome contains 13 protein-coding genes, 2 ribosomal RNA genes, and 22 tRNA genes. The gene order is identical to the reported Terebellids. ATG is used as start codon for all genes. All genes use either TAA or TAG as a stop codon.
The phylogenetic tree based on mitochondrial coding genes’ sequences of P. hessleri and all 13 Scolecida species available on NCBI showed that all terebellids were clustered together. Also, the two alvinellids further grouped together (). Based on these data, primers for individual genes can be designed to study the population connectivity, which will help understand their population connectivity and the conservation of these deep sea animals that are facing increasing human activities (Mengerink et al. Citation2014).
Figure 1. The maximum likelihood (ML) tree of 14 species of Scolecidae based on the concatenated nucleotide sequences of 12 mitochondrial protein-coding (Cytochrome C was omitted because it’s not available in the partial mitogenome of P. sulfincola) and two ribosomal RNA genes. The number at each node is the bootstrap support value. The number after species name is the GenBank accession number.
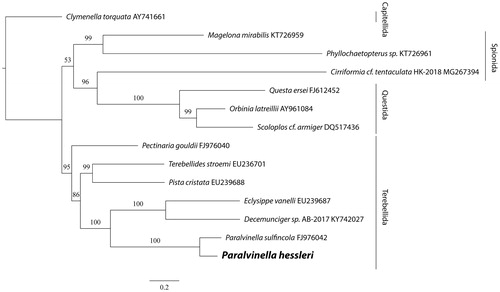
Ethical approval
This article does not contain any studies with human subjects by any of the authors.
Acknowledgements
We appreciate all the assistance provided by the crews on RV ‘Kexue’ and the operation team of the ROV ‘Faxian’.
Disclosure statement
No potential conflict of interest was reported by the authors.
Additional information
Funding
References
- Bankevich A, Nurk S, Antipov D, Gurevich AA, Dvorkin M, Kulikov AS, Lesin VM, Nikolenko SI, Pham S, Prjibelski AD, et al. 2012. SPAdes: a new genome assembly algorithm and its applications to single-cell sequencing. J Comput Biol. 19:455–477.
- Bernt M, Donath A, Juhling F, Externbrink F, Florentz C, Fritzsch G, Putz J, Middendorf M, Stadler PF. 2013. MITOS: improved de novo metazoan mitochondrial genome annotation. Mol Phylogenet Evol. 69:313–319.
- Desbruyeres D, Chevaldonne P, Alayse AM, Jollivet D, Lallier FH, Jouin-Toulmond C, Zal F, Sarradin PM, Cosson R, Caprais JC, et al. 1998. Biology and ecology of the "Pompeii worm" (Alvinella pompejana Desbruyeres and Laubier), a normal dweller of an extreme deep-sea environment: A synthesis of current knowledge and recent developments. Deep-Sea Res Pt Ii. 45:383.
- Desbruyeres D, Laubier L. 1989. Paralvinella-Hessleri, new species of Alvinellidae (Polychaeta) from the Mariana Back-Arc basin hydrothermal vents. P Biol Soc Wash. 102:761–767.
- Desbruyeres D, Laubier L. 1991. Systematics, phylogeny, ecology and distribution of the Alvinellidae (Polychaeta) from deep-sea hydrothermal vents. Ophelia. 5:31–45.
- Juniper SK, Martineu P. 1995. Alvinellids and sulfides at hydrothermal vents of the Eastern Pacific: a review. Am Zool. 35:174–185.
- Mary J, Rogniaux H, Rees JF, Zal F. 2010. Response of Alvinella pompejana to variable oxygen stress: a proteomic approach. Proteomics. 10:2250–2258.
- Mengerink KJ, Van Dover CL, Ardron J, Baker M, Escobar-Briones E, Gjerde K, Koslow JA, Ramirez-Llodra E, Lara-Lopez A, Squires D, et al. 2014. A call for deep-ocean stewardship. Science. 344:696–698.
- Nguyen LT, Schmidt HA, von Haeseler A, Minh BQ. 2015. IQ-TREE: a fast and effective stochastic algorithm for estimating maximum-likelihood phylogenies. Mol Biol Evol. 32:268–274.
- Shigeno S, Ogura A, Mori T, Toyohara H, Yoshida T, Tsuchida S, Fujikura K. 2014. Sensing deep extreme environments: the receptor cell types, brain centers, and multi-layer neural packaging of hydrothermal vent endemic worms. Front Zool. 11:82
- Sicot FX, Mesnage M, Masselot M, Exposito JY, Garrone R, Deutsch J, Gaill F. 2000. Molecular adaptation to an extreme environment: origin of the thermal stability of the pompeii worm collagen. J Mol Biol. 302:811–820.
- Trifinopoulos J, Nguyen LT, von Haeseler A, Minh BQ. 2016. W-IQ-TREE: a fast online phylogenetic tool for maximum likelihood analysis. Nucleic Acids Res. 44:W232–W235.