Abstract
The complete mitogenome sequence of the brown-headed gull, Chroicocephalus brunnicephalus was determined in this study. The 16,771 bp genome consists of 13 protein-coding genes (PCGs), two ribosomal RNA (rRNA) genes, and 22 transfer RNA (tRNA) genes, and a control region (CR). The decoded mitogenome was AT-rich (54.77%) with nine overlapping and 17 intergenic spacer regions. Most of the PCGs were started by a typical ATG initiation codon except for cox1 and nad3. Further, the usual termination codons (AGG, TAG, TAA, and AGA) were used by 11 PCGs except for cox3 and nad4. The concatenated PCGs based Bayesian phylogeny clearly discriminates all the Laridae species and reflects the sister relationship of C. brunnicephalus with C. ridibundus. The present mitogenome-based phylogeny was congruent with the earlier hypothesis and confirmed the evolutionary position of the brown-headed gull as masked species. The generated mitogenome of C. brunnicephalus is almost identical to the previously generated mitogenome from China except for two base pairs in CR. To visualize the population structure of this migratory species, we propose more sampling from different geographical locations and the generation of additional molecular data to clarify the reality.
1. Introduction
The family Laridae (order: Charadriiformes) comprises 105 known species under 20 genera globally (BirdLife International Citation2020). Among them, 38 species under 17 genera were recorded from Indian coastal or inland (Praveen et al. Citation2020). They are medium to large-sized shorebirds, commonly known as gulls. Typically they are white or gray in color with webbed feet and have typical harsh wailing calls. Among all extant gulls, the brown-headed gull Chroicocephalus brunnicephalus usually migrate from China to their wintering localities in Thailand and Cambodia; while Bangladesh, India, Myanmar, and Vietnam being their major stopover locations during such migrations. They can travel an average distance of about 2400 km from their breeding grounds and take one to two weeks to cover this distance (Ratanakorn et al. Citation2012). Due to habitat loss, over-fishing, and other anthropogenic pressures, the population trend of many gulls is declining throughout the world including India (Aarif et al. Citation2014). Furthermore, C. brunnicephalus is also reported to be a host of highly pathogenic avian influenza H5N1 virus and could be a potential carrier for the outbreak within Southeast Asian countries and act as an environmental bio-indicator (Ratanakorn et al. Citation2012; Hasan et al. Citation2014).
Owing to the convergent plumage evolution, the taxonomic approach often flunks to resolve the phylogenetic relationship of Charadriiformes birds (Crochet et al. Citation2000). Later on, the genetic analyses evidenced that, the order Charadriiformes can be classified into three major clades (Paton and Baker Citation2006; Fain and Houde Citation2007; Livezey Citation2010). However, the evolutionary relationship of this group is still perplexing while examining in-depth phylogenetic analysis. Both mitochondrial and nuclear genetic information were well studied to know the systematics status, evolutionary relationships, and population structures of Charadriiformes birds (Liebers et al. Citation2001; Crochet et al. Citation2003; Ericson et al. Citation2003; Paton et al. Citation2003; Thomas et al. Citation2004; van Tuinen et al. Citation2004; Pons et al. Citation2005; Baker et al. Citation2007). The comparative genomics is also employed for estimating the genetic variability, substitution pattern, phylogenetic relationship, and evolution of avifauna (van Tuinen et al. Citation2001; Paton et al. Citation2002; Backström et al. Citation2008; Hackett et al. Citation2008; Künstner et al. Citation2010). As of now, 47 complete mitochondrial genomes of Charadriiformes birds are available in the GenBank database. Among them, 13 mitogenomes of 11 Laridae species were generated from different geographical regions (Slack et al. Citation2007; Ryu and Hwang Citation2012; Yang et al. Citation2012, Citation2016, Citation2017; Yoon et al. Citation2015; Dong et al. Citation2016; Kim and Park Citation2016; Anmarkrud and Lifjeld Citation2017; Skujina et al. Citation2019). The analysis of the complete mitogenomes was also evidenced to characterize various genomic traits within protein-coding genes (PCGs), ribosomal RNA (rRNA), transfer RNA (tRNA), and control region (CR) as well as to detect gene order evolution in birds (Bensch and Härlid Citation2000; Crochet and Desmarais Citation2000; Ruokonen and Kvist Citation2002; Pacheco et al. Citation2011). Hence, this study is aimed to generate the complete mitochondrial genome of the brown-headed gull from India and estimate the phylogeny with other Laridae species to determine the evolutionary relationships.
2. Materials and methods
A naturally dead adult specimen of C. brunnicephalus was collected on 27 February 2019 from the seashore of Coringa Wildlife Sanctuary (16.58 N 82.30 E) in Andhra Pradesh, India (). As the sample was naturally dead, no prior permission was acquired for this biological sampling. The live photograph of the same species was captured from the same locality. The country-level map has been downloaded from the spatial data platform and overlaying by DIVA-GIS 7.5 software (http://www.diva-gis.org). The muscle tissue was collected from the carcass specimen in a sterile condition and stored in 70% ethanol at −80 °C in the National Zoological Collections of Bird Section, Zoological Survey of India, Kolkata under voucher No. 41301/AVES.
Figure 1. (A) Map showing the collection locality of C. brunnicephalus from the southern coast of India. (B) The spherical representation of mitochondrial genome of C. brunnicephalus. Direction of gene transcription is indicated by arrows. PCGs are shown as blue arrows, rRNA genes as coral arrows, tRNA genes as orchid arrows, and non-coding control region as mint rectangle. The GC-skew is plotted using green and violet color sliding window as the deviation from the average in the complete mitogenome. The species photograph was taken by the third author (G.M.) (C) The BA phylogeny based on the concatenated nucleotide sequences of 13 PCGs showing the evolutionary relationship of the studied taxa with other Laridae species. The posterior probability support values were superimposed with each node. The figure was edited with the representative species photograph acquired from the internet using Adobe Photoshop CS version 8.0.
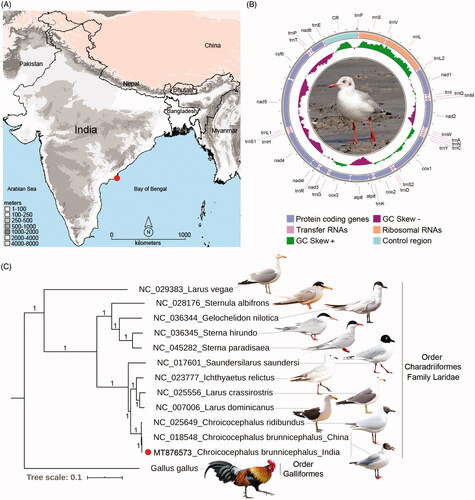
The collected tissue sample was thoroughly chopped by the surgical blade and re-suspended in 200 μl of buffer (50 mM Tris–HCl, 25 mM of EDTA, and 150 mM NaCl), with the addition of 20 μl of proteinase K (20 mg/ml) followed by incubation at 56 °C for overnight. The sample was further religiously vortex with 10 ml buffer (0.32 M Sucrose, 1 mM EDTA, 10 mM Tris–HCl) and centrifuged at 700 × g for 5 min at 4 °C to remove nuclei and cell debris. The supernatant was collected in 1.5 ml Eppendorf tubes and centrifuged at 12,000 × g for 10 min at 4 °C to precipitate the mitochondrial pellet. The mitochondrial DNA was extracted by Qiagen DNeasy Blood & Tissue Kit (QIAGEN Inc., Germantown, MD)..
The complete mitochondrial genome was obtained commercially and the high-quality paired-end data was assembled through NOVOPlasty version 2.6.7 with default parameters (Dierckxsens et al. Citation2017). The genome library was sequenced using the Illumina platform (2 × 150 bp PE chemistry), to generate ∼6 GB data (Illumina, Inc, San Diego, CA). The raw reads were screened using cutadapt tool (http://code.google.com/p/cutadapt/) and low-quality bases were trimmed with a cutoff of Phred quality score (Q score =20). The cox1 sequence of the same species (accession no. NC_018548/JX155863) was used as a reference seed sequence for the present assembly. To confirm the assembly, a similarity search was carried out in the GenBank database using BLASTn version 2.2.28 search (https://blast.ncbi.nlm.nih.gov) algorithm.
The spherical representation of the generated mitogenome of C. brunnicephalus was plotted by CGView Server (http://stoth ard.afns.ualbe rta.ca/cgview_server/) with default parameters (Grant and Stothard Citation2008). The strand direction and arrangements of each PCG, tRNA, rRNA, and CR were checked through MITOS version 806 online web server (http://mitos.bioinf.uni-leipzig.de) (Bernt et al. Citation2013). The overlapping regions and intergenic spacers between the neighbor genes were counted manually through Microsoft Excel. The sequences of PCGs were translated into the putative amino acid sequences on the basis of the vertebrate mitochondrial genetic code. The initiation and termination codons were identified in ClustalX using other publicly available reference sequences of Laridae (Thompson et al. Citation2003). The mitogenome sequence was submitted to the GenBank database through the Bankit submission tool. The mitogenome size and nucleotide composition were calculated using MEGA version 6.0 (Tamura et al. Citation2013).
On the basis of homology search in the Refseq database (https://www.ncbi.nlm.nih.gov/refse q/), 11 Laridae species mitogenomes were downloaded from GenBank and incorporated in the dataset for their phylogenetic relationships. The PCGs were aligned individually by codons using MAFFT algorithm in TranslatorX with L‐INS‐i strategy with GBlocks parameters (Abascal et al. Citation2010). The dataset of all PCGs was concatenated using SequenceMatrix version 1.7.84537 (Vaidya et al. Citation2011). The best suitable model (GTR + G + I) for phylogenetic analysis was calculated by PartitionFinder 2 (Lanfear et al. Citation2016) at CIPRES Science Gateway version 3.3 (Miller et al. Citation2015). The Bayesian analysis (BA) was performed through Mr. Bayes version 3.1.2 and the metropolis‐coupled Markov Chain Monte Carlo (MCMC) was run for 100,000,000 generations with sampling at every 100th generation and 25% of samples were discarded as burn‐in (Ronquist and Huelsenbeck Citation2003). The BA phylogeny was further illustrated in iTOL version 4 (https://itol.embl.de/login.cgi) and edited with Adobe Photoshop CS version 8.0. The database sequence of Gallus gallus (order Galliformes) was as out-group taxa in the phylogenetic analysis.
3. Results and discussion
The complete mitochondrial genome of C. brunnicephalus was determined by using next-generation sequencing approach. The total length of the decoded mitogenome was 16,771 bp and deposited with the accession number (MT876573/NC_050864) in the GenBank database. The complete mitogenome contains 13 PCGs, 22 tRNAs, two rRNAs, and a CR as depicted in other avian mitogenomes (, Table S1). A total of nine genes (nad6 and eight tRNAs) were located on the light strand, while the other genes were encoded on the heavy strand. The overall base-composition of this mitogenome was 30.72% A, 14.13% G, 31.10% C, and 24.04% T. The AT and GC content of the complete mitogenome was 54.77 and 45.23%, respectively. A total of nine overlapping regions with a total length of 34 bp were identified. These sequences varied in length (1–10 bp) with the longest overlapping region present between ATP synthase F0 subunit 8 (atp8) and ATP synthase F0 subunit 6 (atp6). Further, the intergenic spacers spread over 17 regions ranging from 1 to 19 bp with a total length of 68 bp. The longest spacer (19 bp) occurred between NADH dehydrogenase subunit 5 (nad5) and Cytochrome b (Cytb) (Table S1). Most of the PCGs were started by the typical ATG initiation codon with an exception in Cytochrome oxidase subunit I (cox1) with GTG and NADH dehydrogenase subunit 3 (nad3) with ATT. The 11 PCGs used usual termination codons (AGG, TAG, TAA, and AGA), except for Cytochrome oxidase subunit III (cox3) and NADH dehydrogenase subunit 4 (nad4) with incomplete termination codon (T) (Table S1).
The mitogenome based BA phylogeny clearly discriminates all the studied Laridae species with high posterior probability supports (). Both Indian and Chinese C. brunnicephalus sequences were cohesively clustered in the BA tree and reflected the sister relationship with the Black-headed gull, Chroicocephalus ridibundus. The C. brunnicephalus was thought to be a valid species under genus Larus from long back and later on shifted under Chroicocephalus genus based on the mitochondrial markers based phylogeny (Pons et al. Citation2005). This taxonomic revision was further accorded by the subsequent studies (Sternkopf Citation2011; Liebers-Helbig Citation2013). The present complete mitochondrial genome-based phylogeny elucidates congruent results with the earlier hypothesis (Pons et al. Citation2005) and confirmed their evolutionary position as masked species.
The genetic applications are largely employed in the conservation of avifauna around the world (Haig et al. Citation2011). It is also detected that the genetic composition of birds is often altered linked with their fragmented habitats (Brown et al. Citation2004). Further, it is also noticed that the migratory species acquired their energy via mitochondrial adaptations and oxidative phosphorylation during their locomotion (Toews et al. Citation2014). It is also evident that locomotion plays an important role in the evolution of mtDNA and independently evolves different lineages (Pulido Citation2007; Shen et al. Citation2009). The molecular study further suggested the gene flow controlling the expression of migratory behavior of birds (Mueller et al. Citation2011; Pons et al. Citation2014). This analysis elucidates that the nucleotide composition of the Indian C. brunnicephalus mitogenome, sampled from the Southern coast is almost identical to the Chinese mitogenome (accession no. NC_018548/JX155863) except for two base pairs in CR. The genetic information of these two isolates is important and could be adopted for population genetics studies of this Laridae species in near future. However, we suggest more sampling from different geographical locations and subsequent generation of more molecular data to clarify the mitochondrial introgression and function of this migratory species.
Acknowledgments
We are thankful to the Director of Zoological Survey of India (ZSI), Ministry of Environment, Forest and Climate Change (MoEF&CC), Govt. of India for providing necessary molecular facilities and support for the study. The first author (SK) acknowledges the fellowship grant received from the Council of Scientific and Industrial Research (CSIR) Senior Research Associateship (Scientists’ Pool Scheme) Pool No. 9072-A. We thank Avas Pakrashi and Pronomay Karmakar for analytical help.
Disclosure statement
No potential conflict of interest was reported by the authors.
Data availability statement
The complete mitochondrial genome data that support the findings of this study are openly available in NCBI GenBank database at (https://www.ncbi.nlm.nih.gov) with the accession number (MT876573/NC_050864) which permits unrestricted use, distribution, and reproduction in any medium, provided the original work is properly cited.
Additional information
Funding
References
- Aarif KM, Prasadan PK, Basheer PM, Hameed SVA. 2014. Population trend of wintering gulls in Kadalundi-Vallikkunnu Community Reserve, Southern India. J Environ Biol. 36:597–600.
- Abascal F, Zardoya R, Telford MJ. 2010. TranslatorX: multiple alignment of nucleotide sequences guided by amino acid translations. Nucleic Acids Res. 38(Web Server issue):W7–13.
- Anmarkrud JA, Lifjeld JT. 2017. Complete mitochondrial genomes of eleven extinct or possibly extinct bird species. Mol Ecol Resour. 17(2):334–341.
- Backström N, Fagerberg S, Ellegren H. 2008. Genomics of natural bird populations: a gene-based set of reference markers evenly spread across the avian genome. Mol Ecol. 17(4):964–980.
- Baker AJ, Pereira SL, Paton TA. 2007. Phylogenetic relationships and divergence times of Charadriiformes genera: multigene evidence for the Cretaceous origin of at least 14 clades of shorebirds. Biol Lett. 3(2):205–209.
- Bensch S, Härlid A. 2000. Mitochondrial genomic rearrangements in songbirds. Mol Biol Evol. 17(1):107–113.
- Bernt M, Donath A, Jühling F, Externbrink F, Florentz C, Fritzsch G, Pütz J, Middendorf M, Stadler PF. 2013. MITOS: improved de novo metazoan mitochondrial genome annotation. Mol Phylogenet Evol. 69(2):313–319.
- BirdLife International. 2020. IUCN red list for birds. [accessed 2020 Aug 15]. http://www.birdlife.org.
- Brown LM, Ramey IIRR, Tamburini B, Gavin TA. 2004. Population structure and mitochondrial DNA variation in sedentary neotropical birds isolated by forest fragmentation. Conserv Genet. 5(6):743–755.
- Crochet P-A, Bonhomme F, Lebreton J-D. 2000. Molecular phylogeny and plumage evolution in gulls (Larini). J Evol Biol. 13:47–57.
- Crochet PA, Chen JZ, Pons JM, Lebreton JD, Hebert PDN, Bonhomme F. 2003. Genetic differentiation at nuclear and mitochondrial loci among large white-headed gulls: sex-biased interspecific gene flow? Evolution. 57(12):2865–2878.
- Crochet P-A, Desmarais E. 2000. Slow rate of evolution in the mitochondrial control region of gulls (Aves: Laridae). Mol Biol Evol. 17(12):1797–1806.
- Dierckxsens N, Mardulyn P, Smits G. 2017. NOVOPlasty: de novo assembly of organelle genomes from whole genome data. Nucleic Acids Res. 45(4):e18.
- Dong Y, Zhou L, Li B, Zhao G. 2016. The complete mitochondrial genome of the black-headed gull Chroicocephalus ridibundus (Charadriiformes: Laridae). Mitochondrial DNA Part A. 27(3):1991–1992.
- Ericson PG, Envall I, Irestedt M, Norman JA. 2003. Inter-familial relationships of the shorebirds (Aves: Charadriiformes) based on nuclear DNA sequence data. BMC Evol Biol. 3(1):16–154.
- Fain MG, Houde P. 2007. Multilocus perspectives on the monophyly and phylogeny of the order Charadriiformes (Aves). BMC Evol Biol. 7(1):35.
- Grant JR, Stothard P. 2008. The CGView Server: a comparative genomics tool for circular genomes. Nucleic Acids Res. 36(Web Server issue):W181–184.
- Hackett SJ, Kimball RT, Reddy S, Bowie RCK, Braun EL, Braun MJ, Chojnowski JL, Cox WA, Han K-L, Harshman J, et al. 2008. A phylogenomic study of birds reveals their evolutionary history. Science. 320(5884):1763–1768.
- Haig SM, Bronaugh WM, Crowhurst RS, D'Elia J, Eagles-Smith CA, Epps CW, Knaus B, Miller MP, Moses ML, Oyler-McCance S, et al. 2011. Genetic applications in avian conservation. Auk. 128(2):205–229.
- Hasan B, Melhus A, Sandegren L, Alam M, Olsen B. 2014. The gull (Chroicocephalus brunnicephalus) as an environmental bioindicator and reservoir for antibiotic resistance on the coastlines of the Bay of Bengal. Microb Drug Resist. 20(5):466–471.
- Kim JY, Park YC. 2016. The complete mitogenome of the black-tailed gull Larus crassirostris (Charadriiformes: Laridae). Mitochondrial DNA Part A. 27(3):1885–1886.
- Künstner A, Wolf JBW, Backström N, Whitney O, Balakrishnan CN, Day L, Edwards SV, Janes DE, Schlinger BE, Wilson EK, et al. 2010. Comparative genomics based on massive parallel transcriptome sequencing reveals patterns of substitution and selection across 10 bird species. Mol Ecol. 19:266–276.
- Lanfear R, Frandsen PB, Wright AM, Senfeld T, Calcott B. 2016. PartitionFinder 2: new methods for selecting partitioned models of evolution for molecular and morphological phylogenetic analyses. Mol Biol E. 34:772–773.
- Liebers D, Helbig AJ, De Knijff P. 2001. Genetic differentiation and phylogeography of gulls in the Larus cachinnans-fuscus group (Aves: Charadriiformes). Mol Ecol. 10(10):2447–2462.
- Liebers-Helbig D. 2013. The “molecular revolution” and its implications for research ornithological taxonomic. Ornithol Beob. 110:257–269.
- Livezey BC. 2010. Phylogenetics of modern shorebirds (Charadriiformes) based on phenotypic evidence: analysis and discussion. Zool J Linn Soc. 160(3):567–618.
- Miller MA, Schwartz T, Pickett BE, He S, Klem EB, Scheuermann RH, Passarotti M, Kaufman S, O'Leary MA. 2015. A RESTful API for access to phylogenetic tools via the CIPRES science gateway. Evol Bioinform Online. 11:43–48.
- Mueller JC, Pulido F, Kempenaers B. 2011. Identification of a gene associated with avian migratory behaviour. Proc Biol Sci. 278(1719):2848–2856.
- Pacheco MA, Battistuzzi FU, Lentino M, Aguilar RF, Kumar S, Escalante AA. 2011. Evolution of modern birds revealed by mitogenomics: timing the radiation and origin of major orders. Mol Biol Evol. 28(6):1927–1942.
- Paton TA, Baker AJ. 2006. Sequences from 14 mitochondrial genes provide a well-supported phylogeny of the Charadriiform birds congruent with the nuclear RAG-1 tree. Mol Phylogenet Evol. 39(3):657–667.
- Paton TA, Baker AJ, Groth JG, Barrowclough GF. 2003. RAG-1 sequences resolve phylogenetic relationships within Charadriiform birds. Mol Phylogenet Evol. 29(2):268–278.
- Paton T, Haddrath O, Baker AJ. 2002. Complete mitochondrial DNA genome sequences show that modern birds are not descended from transitional shorebirds. Proc Biol Sci. 269(1493):839–846.
- Pons J-M, Hassanin A, Crochet P-A. 2005. Phylogenetic relationships within the Laridae (Charadriiformes: Aves) inferred from mitochondrial markers. Mol Phylogenet Evol. 37(3):686–699.
- Pons J-M, Sonsthagen S, Dove C, Crochet P-A. 2014. Extensive mitochondrial introgression in North American great black-backed gulls (Larus marinus) from the American herring gull (Larus smithsonianus) with little nuclear DNA impact. Heredity (Edinb). 112(3):226–239.
- Praveen J, Jayapal R, Pittie A. 2020. Checklist of the birds of India (v4.0). [accessed 2020 Aug 19]. http://www.indianbirds.in/india/.
- Pulido F. 2007. The genetics and evolution of avian migration. BioScience. 57(2):165–174.
- Ratanakorn P, Wiratsudakul A, Wiriyarat W, Eiamampai K, Farmer AH, Webster RG, Chaichoune K, Suwanpakdee S, Pothieng D, Puthavathana P. 2012. Satellite tracking on the flyways of brown-headed gulls and their potential role in the spread of highly pathogenic avian influenza H5N1 virus. PLoS One. 7(11):e49939.
- Ronquist F, Huelsenbeck JP. 2003. MrBayes 3: Bayesian phylogenetic inference under mixed models. Bioinformatics. 19(12):1572–1574.
- Ruokonen M, Kvist L. 2002. Structure and evolution of the avian mitochondrial control region. Mol Phylogenet Evol. 23(3):422–432.
- Ryu SH, Hwang UW. 2012. Complete mitochondrial genome of Saunders’s gull Chroicocephalus saundersi (Charadriiformes, Laridae). Mitochondrial DNA. 23(2):134–136.
- Shen Y-Y, Shi P, Sun Y-B, Zhang Y-P. 2009. Relaxation of selective constraints on avian mitochondrial DNA following the degeneration of flight ability. Genome Res. 19(10):1760–1765.
- Skujina I, Healey AJE, de Becquevort S, Shaw PW, McMahon R, Morgan C, Evans C, Taylor R, Hegarty M, McKeown NJ. 2019. The complete mitochondrial genome of record-breaking migrant Arctic tern (Sterna paradisaea). Mitochondrial DNA Part B. 4(2):2738–2739.
- Slack KE, Delsuc F, Mclenachan PA, Arnason U, Penny D. 2007. Resolving the root of the avian mitogenomic tree by breaking up long branches. Mol Phylogenet Evol. 42(1):1–13.
- Sternkopf V. 2011. Molekulargenetische Untersuchung in der Gruppe der Möwen (Laridae) zur Erforschung der Verwandtschaftsbeziehungen und phylogeographischer Differenzierung. Inauguraldissertation zur Erlangung des akademischen Grades doctor rerum naturalium (Dr. rer. nat.) an der Mathematisch-Naturwissenschaftlichen Fakultät der Ernst-Moritz-Arndt-Univ. Greifswald, Germany: Ernst-Moritz-Arndt-Univ.
- Tamura K, Stecher G, Peterson D, Filipski A, Kumar S. 2013. MEGA6: molecular evolutionary genetics analysis version 6.0. Mol Biol Evol. 30(12):2725–2729.
- Thomas GH, Wills MA, Szekely T. 2004. Phylogeny of shorebirds, gulls, and alcids (Aves: Charadrii) from the cytochrome-b gene: Parsimony, Bayesian inference, minimum evolution, and quartet puzzling. Mol Phylogenet Evol. 30(3):516–526.
- Thompson JD, Gibson TJ, Higgins DG. 2003. Multiple sequence alignment using ClustalWand ClustalX. Curr Protoc Bioinformatics. 0(1):2.3.1–2.3.22.
- Toews DPL, Mandic M, Richards JG, Irwin DE. 2014. Migration, mitochondria, and the yellow-rumped warbler. Evolution. 68(1):241–255.
- Vaidya G, Lohman DJ, Meier R. 2011. SequenceMatrix: concatenation software for the fast assembly of multi-gene datasets with character set and codon information. Cladistics. 27(2):171–180.
- van Tuinen M, Butvill DB, Kirsch JAW, Hedges SB. 2001. Convergence and divergence in the evolution of aquatic birds. Proc Biol Sci. 268(1474):1345–1350.
- van Tuinen M, Waterhouse D, Dyke GJ. 2004. Avian molecular systematics on the rebound: a fresh look at modern shorebird phylogenetic relationships. J Avian Bio. 35(3):191–194.
- Yang C, Wang Q-X, Huang Y, Xiao H. 2012. Analysis of the complete mitochondrial genome sequence of Larus brunnicephalus (Aves, Laridae). Hereditas (Beijing). 34(11):1434–1446.
- Yang C, Wang Q-X, Huang Y, Xiao H. 2016. Complete mitochondrial genome of Relict Gull, Larus relictus (Charadriiformes: Laridae). Mitochondrial DNA Part A. 27(1):411–412.
- Yang C, Wang Q-X, Li X-J, Yuan H, Xiao H, Huang Y. 2017. The mitogenomes of Gelochelidon nilotica and Sterna hirundo (Charadriiformes, Sternidae) and their phylogenetic implications. Mitochondrial DNA Part B. 2(2):601–603.
- Yoon KB, Cho CU, Park YC. 2015. The mitochondrial genome of the Saunders’s gull Chroicocephalus saundersi (Charadriiformes: Laridae) and a higher phylogeny of shorebirds (Charadriiformes). Gene. 572(2):227–236.