Abstract
Completed chloroplast genome of Zoysia matrella (L.) Merr. isolated in Korea is 135,888 bp long (GC ratio is 38.4%) and has four subregions: 81,370 bp of large single copy (36.3%) and 12,594 bp of small single copy (32.7%) regions are separated by 20,962 bp of inverted repeat (44.1%) regions including 130 genes (83 protein-coding genes, eight rRNAs, 38 tRNAs, and one pseudogene). 28 SNPs and 57 INDELs were identified ss intraspecific variations against previously sequenced chloroplast genome. Phylogenetic trees show that Z. matrella and Z. tenuifolia (=Z. pacifica) are clustered in one clade with low level of variations on chloroplast genomes.
Zoysiagrass is an herbaceous and perennial plant and has a wide range of uses such as soil conservation, landscaping, playgrounds, and parks (Bae et al. Citation2008), and the demand and commercialization are rapidly increasing (Choi J-S et al. Citation2017). Zoysia matrella (L.) Merr is one of the native Zoysia spp. in Korea and has a delicate and dense foliage. Molecular breeding has recently been applied to develop new varieties of turfgrass (Bae et al. Citation2008; Choi J-S et al. Citation2017). Chloroplast genome of cultivar Chiba Fair Green was sequenced (Tanaka, Hirakawa, et al. Citation2016) to clarify species identification problem of Z. matrella (Tanaka, Tokunaga, et al. Citation2016) and to unravel their evolutionary relationships. To investigates intraspecific relationship of Z. matrella, we completed the chloroplast genome of Z. matrella isolated in Korea.
Total DNA of Z. matrella isolated from maintained line in National Institute of Agricultural Sciences, Jeonju city, Korea (35°49′47.94ʺN 127°03′55.2ʺE) for conserving natural isolate in Jejudo island in Korea, was extracted from fresh leaves by using a DNeasy Plant Mini Kit (QIAGEN, Hilden, Germany). Voucher was deposited in InfoBoss Cyber Herbarium (IN; http://herbarium.infoboss.co.kr/; Suhyeon Park; [email protected]) under the voucher number IB-01031. Genome was sequenced using NovaSeq6000 at Macrogen Inc., Korea, and de novo assembly and confirmation were performed by Velvet v1.2.10 (Zerbino and Birney Citation2008), SOAPGapCloser v1.12 (Zhao et al. Citation2011), BWA v0.7.17 (Li Citation2013), and SAMtools v1.9 (Li et al. Citation2009) under the environment of Genome Information System (GeIS; http://geis.infoboss.co.kr/; Park et al., in preparation). Geneious R11 v11.0.5 (Biomatters Ltd., Auckland, New Zealand) was used for annotation based on Z. matrella chloroplast (AP014937; Tanaka, Hirakawa, et al. Citation2016).
Chloroplast genome of Z. matrella (GenBank accession is MT983887) is 135,888 bp (GC ratio is 38.4%) and has four subregions: 81,370 bp of large single copy (LSC; 36.3%) and 12,594 bp of small single copy (SSC; 32.7%) regions are separated by 20,962 bp of inverted repeat (IR; 44.1%). It contains 130 genes (83 protein-coding genes, eight rRNAs, 38 tRNAs and one pseudogene); 19 genes (seven protein-coding gene, four rRNAs, and eight tRNAs) are duplicated in IR regions. Interestingly, trnfM-CAU pseudogene was found at 37,280 to 37,333, where hotspot of pseudogenes identified in Triticum aestivum chloroplast genome (Ogihara et al. Citation2002)
28 SNPs and 57 INDELs were identified from the pair-wide alignment of two Z. matrella chloroplast genomes. 8 out of 57 INDELs are 2-bp long and three 3-bp INDELs, two 4-bp INDELs, one 5-bp, and one 10-bp INDEL are also identified. Numbers of SNPs and INDELs are less than those of species between two countries (Jeon et al. Citation2019; Oh and Park Citation2020; Park, Kim, Lee Citation2019); however, similar to those identified inside Korea (Choi YG et al. Citation2019; Kim, Yi, et al. Citation2019) and even larger than several plants (Park and Kim Citation2019; Kim et al. Citation2020; Min et al. Citation2019; Park and Oh Citation2020; Park, Kim, Xi, et al. Citation2019), indicating that number of intraspecific variations of Z. matrella between Korea and Japan is relatively small.
Eleven chloroplast genomes including two Z. matrella chloroplasts were selected from phylogenetically neighbor species based on previous phylogenetic study (Hilu and Alice Citation2001). They were used for constructing bootstrapped maximum-likelihood and neighbor-joining trees using MEGA X (Kumar et al. Citation2018) and Bayesian inference tree with MrBayes v3.2.7a (Ronquist et al. Citation2012) after aligning whole chloroplast genomes using MAFFT v7.450 (Katoh and Standley Citation2013). Phylogenetic trees show that our chloroplast genome is clustered as one clade with previously sequenced chloroplast genome of Z. matrella and Zoysia tenuifolia with high supportive values (). Interestingly, only 4 SNPs and 19 INDELs were identified between our Z. matrella and Z. tenuifolia, which is smaller than intraspecific variations of Z. matrella. This phenomenon was also found in Viburnum (Choi YG et al. Citation2019) and Pseudostellaria (Kim, Heo, et al. Citation2019), displaying discrepancy between morphology and molecular markers. Because Z. tenuifolia is considered as Zoysia pacifica (Goudswaard Citation1980; Loch et al. Citation2017) and there are hybrids between Z. tenuifolia and Z. pacifica (Tanaka, Tokunaga, et al. Citation2016), this low number of variations between the two species require more investigation of the two species in both morphological and genetical aspects.
Figure 1. Phylogenetic tree was drawn based on maximum-likelihood tree based on alignment of eleven chloroplast genomes of Zoysia and neighbor species including one outgroup species. The numbers above branches indicate bootstrap support values of maximum likelihood, neighbor-joining, and Bayesian phylogenetic trees, respectively.
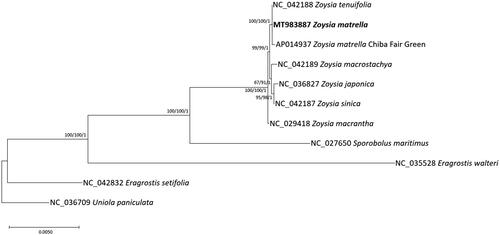
Disclosure statement
No potential conflict of interest was reported by the author(s).
Data availability statement
Mitochondrial genome sequence can be accessed via accession number MT983887 in GenBank of NCBI at https://www.ncbi.nlm.nih.gov. The associated BioProject, SRA, and Bio-Sample numbers are PRJNA685656, SAMN17088999, and SRR13258751, respectively.
References
- Bae TW, Vanjildorj E, Song SY, Nishiguchi S, Yang SS, Song IJ, Chandrasekhar T, Kang TW, Kim JI, Koh YJ, et al. 2008. Environmental risk assessment of genetically engineered herbicide-tolerant Zoysia japonica . J Environ Qual. 37(1):207–218.
- Choi J-S, Yang G-M, Bae E-J, Park Y-B, Lee K-S. 2017. Development of new hybrid zoysiagrass cultivar 'Seah. Weed Turf Sci. 6(4):306–312.
- Choi YG, Yun N, Park J, Xi H, Min J, Kim Y, Oh S-H. 2019. The second complete chloroplast genome sequence of the Viburnum erosum (Adoxaceae) showed a low level of intra-species variations. Mitochondrial DNA B Resour. 5(1):271–272.
- Goudswaard P. 1980. The genus Zoysia (Gramineae) in Malesia. Blumea. 26(1):169–175.
- Hilu KW, Alice LA. 2001. A phylogeny of Chloridoideae (Poaceae) based on matK sequences. Syst Bot. 26(2):386–405.
- Jeon J-H, Park H-S, Park JY, Kang TS, Kwon K, Kim YB, Han J-W, Kim SH, Sung SH, Yang T-J. 2019. Two complete chloroplast genome sequences and intra-species diversity for Rehmannia glutinosa (Orobanchaceae). Mitochondrial DNA Part B. 4(1):176–177.
- Katoh K, Standley DM. 2013. MAFFT multiple sequence alignment software version 7: improvements in performance and usability. Mol Biol Evol. 30(4):772–780.
- Kim Y, Heo K-I, Park J. 2019. The second complete chloroplast genome sequence of Pseudostellaria palibiniana (Takeda) Ohwi (Caryophyllaceae): intraspecies variations based on geographical distribution. Mitochondrial DNA Part B. 4(1):1310–1311.
- Kim Y, Park J, Chung Y. 2020. The comparison of the complete chloroplast genome of Suaeda japonica Makino presenting different external morphology (Amaranthaceae). Mitochondrial DNA Part B. 5(2):1616–1618.
- Kim Y, Yi J-S, Min J, Xi H, Kim DY, Son J, Park J, Jeon J-I. 2019. The complete chloroplast genome of Aconitum coreanum (H. Lév.) Rapaics (Ranunculaceae). Mitochondrial DNA B Resour. 4(2):3404–3406.
- Kumar S, Stecher G, Li M, Knyaz C, Tamura K. 2018. MEGA X: molecular evolutionary genetics analysis across computing platforms. Mol Biol Evol. 35(6):1547–1549.
- Li H, Handsaker B, Wysoker A, Fennell T, Ruan J, Homer N, Marth G, Abecasis G, Durbin R. 2009. The sequence alignment/map format and SAMtools. Bioinformatics. 25(16):2078–2079.
- Li H. 2013. Aligning sequence reads, clone sequences and assembly contigs with BWA-MEM. arXiv preprint arXiv:13033997.
- Loch DS, Ebina M, Choi JS, Han L. 2017. Ecological Implications of Zoysia species, distribution, and adaptation for management and use of zoysiagrasses. Int Turf Soc Res J. 13(1):11–25.
- Min J, Park J, Kim Y, Kwon W. 2019. The complete chloroplast genome of Artemisia fukudo Makino (Asteraceae): providing insight of intraspecies variations. Mitochondrial DNA Part B. 4(1):1510–1512.
- Ogihara Y, Isono K, Kojima T, Endo A, Hanaoka M, Shiina T, Terachi T, Utsugi S, Murata M, Mori N, et al. 2002. Structural features of a wheat plastome as revealed by complete sequencing of chloroplast DNA. Mol Genet Genomics. 266(5):740–746.
- Oh SH, Park J. 2020. The complete chloroplast genome of Euscaphis japonica (Thunb.) Kanitz (Staphyleaceae) isolated in Korea. Mitochondrial DNA Part B. 5(3):3769–3771.
- Park J, Kim Y. 2019. The second complete chloroplast genome of Dysphania pumilio (R. Br.) mosyakin & clemants (Amranthaceae): intraspecies variation of invasive weeds. Mitochondrial DNA Part B. 4(1):1428–1429.
- Park J, Kim Y, Lee K. 2019. The complete chloroplast genome of Korean mock strawberry, Duchesnea chrysantha (Zoll. & Moritzi) Miq.(Rosoideae). Mitochondrial DNA Part B. 4(1):864–865.
- Park J, Kim Y, Xi H, Oh YJ, Hahm KM, Ko J. 2019. The complete chloroplast genome of common camellia tree in Jeju island, Korea, Camellia japonica L. (Theaceae): intraspecies variations on common camellia chloroplast genomes. Mitochondrial DNA Part B. 4(1):1292–1293.
- Park J, Oh S-H. 2020. A second complete chloroplast genome sequence of Fagus multinervis Nakai (Fagaceae): intraspecific variations on chloroplast genome. Mitochondrial DNA Part B. 5(2):1868–1869.
- Ronquist F, Teslenko M, Van Der Mark P, Ayres DL, Darling A, Höhna S, Larget B, Liu L, Suchard MA, Huelsenbeck JP. 2012. MrBayes 3.2: efficient Bayesian phylogenetic inference and model choice across a large model space. Syst Biol. 61(3):539–542.
- Tanaka H, Hirakawa H, Muguerza M, Hashiguchi M, Tabata S, Akashi R, Sato S. 2016. The complete chloroplast genome sequence of Zoysia matrella (L.). Merr Crop Sci. 56(3):1206–1212.
- Tanaka H, Tokunaga R, Muguerza M, Kitazaki Y, Hashiguchi M, Sato S, Tabata S, Akashi R. 2016. Genetic structure and speciation of zoysiagrass ecotypes collected in Japan. Crop Sci. 56(2):818–826.
- Zerbino DR, Birney E. 2008. Velvet: algorithms for de novo short read assembly using de Bruijn graphs. Genome Res. 18(5):821–829.
- Zhao Q-Y, Wang Y, Kong Y-M, Luo D, Li X, Hao P. 2011. Optimizing de novo transcriptome assembly from short-read RNA-Seq data: a comparative study. BMC Bioinf. 12(Suppl 14):S2.