Abstract
The full mitogenome of an ethanol-preserved museum specimen of Ceramaster japonicus was determined using the NGS Illumina MiSeq platform. The specimen was collected from Tosa Bay, Japan, facing the Pacific Ocean (33.0781 N 134.0601 E), at 700 m depth in 2011. The mitogenome shows a typical metazoan genomic structure, with all of the 37 genes included in its 16,370 base-long mitogenome. We conducted phylogenetic analyses using a data set including 18 publicly available asteroids rooted against five ophiuroids as outgroups. The result confirms the position of C. japonicus in the order Valvatida. The complete mitogenome of C. japonicus reported here is the first reported for the family Goniasteridae Forbes, 1841.
Content
The deep-sea asteroid Ceramaster japonicus is one of the most commonly encountered asteroids in the Japanese deep-sea benthic communities of the Pacific Ocean (Horikoshi et al. Citation1990). The species occurs widely in the Pacific and has been reported from the deep continental shelves along the northern Pacific rim, including the Alaskan coast, the Bering Sea, and off Washington (Fisher Citation1911; Setiamarga et al. Citation2019). Here, we report the full mitochondrial genome (mitogenome) sequence of Ceramaster japonicus. This is the first report of the complete mitochondrial genome of the family Goniasteridae (Asteroidea, Valvatacea, Valvatida). Goniasterids are the most diverse family of asteroids, including some 256 living species within 65 genera (Mah and Blake Citation2012). The complete mitochondrial genome of the ophidiasterid, Linckia laevigata, a species also representing the large Valvatacea cluster has recently been reported by Hiruta et al. (Citation2020).
Tissue was collected from the tube feet of an ethanol-preserved specimen from the collection of the National Museum of Nature and Science, Japan (Voucher No. NSMT E-11866). The specimen was collected in 2011 from Tosa Bay, Kochi Prefecture, Japan, facing the Pacific Ocean (33.0781 N 134.0601 E), at the depth of 700 m. Total genomic DNA was extracted from the tissue sample using standard CTAB-Phenol/Chloroform protocol, purified using magnetic beads, and then fragmented and ligated to adaptors using the QiaSeq FX DNA Library Kit (QIAGEN). After fragment size and quality checks (TapeStation, Agilent Technologies), the DNA sample was run on a MiSeq Illumina Next Generation Sequencer. Obtained sequence fragments were edited, manipulated, and then assembled into a circular contig using CLC Genomics Workbench ver. 12 (QIAGEN) under default settings. Assembled mitogenome sequence was annotated using the MITOS web server (Bernt et al. Citation2013).
The newly sequenced mitogenome of Ceramaster japonicus was 16,376 bases-long, and was registered on Genbank (Accession number: LC592775). Its genomic structure is as follows: (1) There are 13 protein-coding, two rRNA, and 22 tRNA genes, (2) There is one control region, located between the tRNA-Thr and LSU-rRNA (575 bp-long); (3) Most protein genes are coded on the H chain, except for ND1, ND2, and ND6. Meanwhile, ND4L is located on the H chain; (4) Only the SSU-rRNA coding gene is located on the H chain; (5) 11 tRNA genes (tRNA-Asp, tRNA-Val, tRNA-Ala, tRNA-Gln, tRNA-Arg, tRNA-Lys, tRNA-His, tRNA-Ser (agc), tRNA-Phe, tRNA-Glu, and tRNA-Thr) are located on the H chain. The total GC content the mitogenome was 39.3%, which is common among asteroid species (Mu et al. Citation2018).
In order to check the molecular phylogenetic position of C. japonicus, we collected eighteen asteroids rooted against five ophiuroid outgroups sequences from GenBank, and included them in the data set for phylogenetic analyses. We conducted a maximum likelihood phylogenetic analysis (Yang Citation1994) using the GUI version of RAxML (Silvestro and Michalak Citation2012; Stamatakis Citation2014) and neighbor joining analysis using MEGA X (Kumar et al. Citation2018), of which methods are detailed in the legend of .
Figure 1. The maximum likelihood (ML) phylogenetic tree showing the position of Ceramaster japonicus with bootstrap supports (%) shown as ML/neighbor joining (NJ). Obtained NJ tree showed a congruent topology (not shown). Phylogenetic analyses were conducted on the data matrix (10,911 positions) including all concatenated nucleotide sequences of the mitogenomes except the third codon positions. Gene sequences were aligned individually using the online version of MAFFT under default settings (Katoh and Standley Citation2013). Aligned sequences were individually edited using the online version of GBlocks using the least stringent settings (Castresana Citation2000). Partitioned ML analyses (four partitions: 1st codon, 2nd codon, rRNA, tRNA) were performed with RAxML-GUI ver. 1-5b1 (Silvestro and Michalak Citation2012; Stamatakis Citation2014), with the GTR + Γ nucleotide substitution model (Yang Citation1994). The rapid bootstrap analyses were conducted with 1000 replications, with four threads running in parallel. NJ analyses were conducted using MEGA X (Kumar et al. Citation2018), under the Maximum Composite Likelihood substitution model, GTR nucleotide substitution rate, and 50% cutoff for each site with 1000 bootstrap replications.
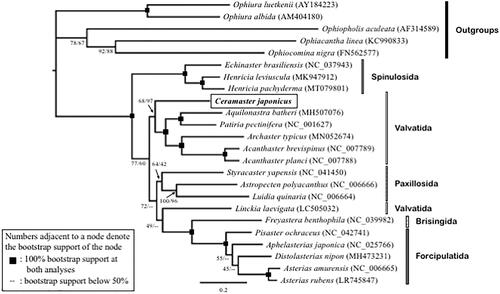
Only the topology of the maximum likelihood tree, with the bootstrap supports of both the maximum likelihood and neighbor joining analyses, are shown on . Our phylogenetic tree supports the monophyly of living Asteroidea, rooted against Ophiuroidea. agrees with monophyletic support for several historically recognized groups within the Asteroidea (Mah and Foltz Citation2011a, Citation2011b; Linchangco et al. Citation2017), such as the Forcipulatida, Brisingida, Paxillosida, and the Spinulosida. However, Valvatida was paraphyletic, with Linckia laevigata present as the sister clade to the Forcipulatacea (Forcipulatida + Brisingida), and thus away from other sampled valvatidans (Ceramaster, Archaster, Acanthaster, Patiria, and Aquilonastra). Many of the basal relationships, such as the Forcipulatacea + Paxillosida also disagree with those observed in prior accounts (e.g. Linchangco et al. Citation2017). Linckia laevigata’s position here differs from its position in the tree presented by Hiruta et al. (Citation2020) which showed it as sister to two members of Paxillosida, Luidia and Astropecten. This is likely attributable to the different number of markers used in previous studies. Linchangco et al. Citation2017 included 2,683 nuclear loci, ca. 1 million bases, and Hiruta et al. Citation2020 included only the 13 protein coding genes of the mitogenomes versus our study which includes all 37 mitogenomic genes, ca. 10,911 positions. These are all in contrast to the earlier study of Mah and Foltz (Citation2011a, b) which included the lowest number of nucleotides analyzed (three gene fragments, 1084 bases) but with the highest number of taxa (180 OTUs).
While the lack of molecular characters might adversely affect phylogenies (Rosenberg and Kumar Citation2001; Wiens et al. Citation2008), taxon sampling is a highly significant consideration when interpreting and comparing the topologies presented herein (Pollock et al. Citation2002; Hillis et al. Citation2003; Heath et al. Citation2008). Ceramaster’s addition to the tree is the first member of the Goniasteridae sampled for a complete mitogenome. The Goniasteridae is the most diverse group within the Asteroidea, and has demonstrated important relationships with other members of the Valvatida, such as the Asterodiscididae, the Oreasteridae, and the Ophidiasteridae (e.g. Blake and Portell Citation2011; Mah Citation2017).
Furthermore, at the timing of writing, neither our tree nor that of Hiruta et al. (Citation2020) included any members of the Velatida, the third major clade within the Asteroidea (Linchangco et al. Citation2017). The lack of comprehensive taxon sampling in prior studies may have resulted in Long Branch Attraction artifacts which might have led to the erroneous grouping of some OTUs (Wiens et al. Citation2008). Ideally, future studies must include a more comprehensive taxonomic survey, in order to provide a clearer picture of asteroid phylogeny. We are confident, however, that the result presented here will be useful for future molecular studies addressing phylogenetic and other systematic questions in the Asteroidea, with particular reference to hyper-diverse groups, such as the Goniasteridae and the Valvatacea.
Acknowledgements
The authors would like to thank Rena Shiba, Nazifa Naziha bt. Razali, Yuki Kamito, Nagisa Nakaji, Satoko Nakashima, and Tamami Ohara (National Institute of Technology (KOSEN), Wakayama College) for their assistance on sampling, molecular experiments, and data-set building for the phylogenetic analyses in this study.
Disclosure statement
The authors declare that there is no conflict of interest. All authors are responsible for the content and writing of the paper.
Data availability statement
The full mitochondrial genome sequence reported in this study is registered in and openly available from the National Center for Biotechnology Information (NCBI) Genbank database (Accession No.: LC592775; https://www.ncbi.nlm.nih.gov/nuccore/LC592775.1).
A specimen was deposited at National Museum of Nature and Science, Japan (Toshihiko Fujita; [email protected]) under the voucher number NSMT E-11866.
Additional information
Funding
References
- Bernt M, Donath A, Jühling F, Externbrink F, Florentz C, Fritzsch G, Pütz J, Middendorf M, Stadler PF. 2013. MITOS: improved de novo metazoan mitochondrial genome annotation. Mol Phylogenet Evol. 69(2):313–319.
- Blake DB, Portell RW. 2011. Kionaster petersonae, n. gen. and sp. (Asteroidea), the first fossil occurrence of the Asterodiscididae, from the Miocene of Florida. Swiss J Palaeontol. 130(1):25–42.
- Castresana J. 2000. Selection of conserved blocks from multiple alignments for their use in phylogenetic analysis. Mol Biol Evol. 17(4):540–552.
- Fisher WK. 1911. Asteroidea of the North Pacific and adjacent waters: phanerozonia and spinulosida. Bull US Natl Mus. 76(76):i–420.
- Heath TA, Hedtke SM, Hillis DM. 2008. Taxon sampling and the accuracy of phylogenetic analyses. J Syst Evol. 46(3):239–257.
- Hillis DM, Pollock DD, McGuire JA, Zwickl DJ. 2003. Is sparse taxon sampling a problem for phylogenetic inference? Syst Biol. 52(1):124–126.
- Hiruta SF, Arai M, Chavanich S, Viyakarn V, Fujita T. 2020. Complete mitochondrial genome of a sea star, Linckia laevigata (Echinodermata, Asteroidea, Valvatida, Ophidiasteridae). Mitochondrial DNA Part B. 5(2):1342–1343.
- Horikoshi M, Fujita T, Ohta S. 1990. Benthic associations in bathyal and hadal depths off the Pacific coast of north eastern Japan: physiognomies and site factors. Prog Oceanogr. 24(1–4):331–339.
- Katoh K, Standley DM. 2013. MAFFT multiple sequence alignment software version 7: improvements in performance and usability. Mol Biol Evol. 30(4):772–780.
- Kumar S, Stecher G, Li M, Knyaz C, Tamura K. 2018. MEGA X: molecular evolutionary genetics analysis across computing platforms. Mol Biol Evol. 35(6):1547–1549.
- Linchangco GV, Jr Foltz DW, Reid R, Williams J, Nodzak C, Kerr AM, Miller AK, Hunter R, Wilson NG, Nielsen WJ, et al. 2017. The phylogeny of extant starfish (Asteroidea: Echinodermata) including Xyloplax, based on comparative transcriptomics. Mol Phylogenet Evol. 115:161–170.
- Mah C, Blake DB. 2012. Global diversity and phylogeny of the Asteroidea (Echinodermata). PLoS One. 7(4):e35644.
- Mah CL. 2017. Overview of the Ferdina-like Goniasteridae (Echinodermata: Asteroidea) including a new subfamily, three new genera and fourteen new species. Zootaxa. 4271(1):1–72.
- Mah CL, Foltz DW. 2011a. Molecular phylogeny of the Valvatacea (Asteroidea, Echinodermata). Zool J Linnean Soc. 161(4):769–788.
- Mah CL, Foltz DW. 2011b. Molecular phylogeny of the Forcipulatacea (Asteroidea: Echinodermata): systematics & biogeography. Zool J Linnean Soc. 162(3):646–660.
- Mu W, Liu J, Zhang H. 2018. The first complete mitochondrial genome of the Mariana Trench Freyastera benthophila (Asteroidea: Brisingida: Brisingidae) allows insights into the deep-sea adaptive evolution of Brisingida. Ecol Evol. 8(22):10673–10686.
- Pollock DD, Zwickl DJ, McGuire JA, Hillis DM. 2002. Increased taxon sampling is advantageous for phylogenetic inference. Syst Biol. 51(4):664–671.
- Rosenberg MS, Kumar S. 2001. Incomplete taxon sampling is not a problem for phylogenetic inference. Proc Natl Acad Sci USA. 98(19):10751–10756.
- Setiamarga DHE, Shiba R, Kamito Y, Yamamoto M, Bt Razali NN, Arai M, Fujita F, Mah CL. 2019. Two partial mitochondrial gene sequences (COI and 12S-rRNA) suggested that Ceramaster japonicus (Sladen, 1889) and Ceramaster patagonicus (Sladen, 1889) (Asteroidea: Goniasteridae) from the Japanese waters are probably of the same species. Zoosymposia. 15(1):129–140.
- Silvestro D, Michalak I. 2012. raxmlGUI: a graphical front-end for RAxML. Org Divers Evol. 12(4):335–337.
- Stamatakis A. 2014. RAxML version 8: a tool for phylogenetic analysis and post-analysis of large phylogenies. Bioinformatics. 30(9):1312–1313.
- Wiens JJ, Kuczynski CA, Smith SA, Mulcahy DG, Sites Jr, JW, Townsend TM, Reeder TW. 2008. Branch lengths, support, and congruence: testing the phylogenomic approach with 20 nuclear loci in snakes. Syst Biol. 57(3):420–431.
- Yang Z. 1994. Maximum likelihood phylogenetic estimation from DNA sequences with variable rates over sites: approximate methods. J Mol Evol. 39(3):306–314.