Abstract
The Gray’s sea fan, Paramuricea grayi (Johnson, 1861), typically inhabits deep littoral and circalittoral habitats of the eastern temperate and tropical Atlantic Ocean. Along the Iberian Peninsula, where P. grayi is a dominant constituent of circalittoral coral gardens, two segregating lineages (yellow and purple morphotypes) were recently identified using single-copy nuclear orthologues. The mitochondrial genomes of 9 P. grayi individuals covering both color morphotypes were assembled from RNA-seq data, using samples collected at three sites in southern (Sagres and Tavira) and western (Cape Espichel) Portugal. The complete circular mitogenome is 18,668 bp in length, has an A + T-rich base composition (62.5%) and contains the 17 genes typically found in Octocorallia: 14 protein-coding genes (atp6, atp8, cob, cox1-3, mt-mutS, nad1-6, and nad4L), the small and large subunit rRNAs (rns and rnl), and one transfer RNA (trnM). The mitogenomes were nearly identical for all specimens, though we identified a noteworthy polymorphism (two SNPs 9 bp apart) in the mt-mutS of one purple individual that is shared with the sister species P. clavata. The mitogenomes of the two species have a pairwise sequence identity of 99.0%, with nad6 and mt-mutS having the highest rates of non-synonymous substitutions.
Paramuricea grayi (Johnson, 1861), colloquially referred to as Gray’s sea fan, is an octocoral species typically inhabiting deep littoral and circalittoral habitats (20-200 m depth; with occasional reports from the upper bathyal zone) of the eastern temperate and tropical Atlantic Ocean, from the Lusitanian-Mauritanian East Atlantic south to the Gulf of Guinea and Angola (Grasshoff Citation1977, Citation1992; Altuna Citation1991). Although historically described as an eastern Atlantic species, recent studies have extended the distribution range of P. grayi to the NW Atlantic (Walting et al. Citation2011; Thoma Citation2013) and Mediterranean Sea (Quattrini et al. Citation2022). In regions like the Algarve in southern Portugal, P. grayi is an important constituent of circalittoral coral gardens, often dominating coral communities and occurring at high densities despite potentially severe impacts from bottom-contact fisheries (Dias et al. Citation2020; Nestorowicz et al. Citation2021).
We recently determined the identity of multiple populations of Paramuricea in southern and western Portugal previously identified as P. clavata to be P. grayi, and identified two color morphotypes of P. grayi as segregating lineages based on multi-locus genotyping and phylogenomic analyses (Coelho et al. Citation2022; ). While P. clavata and P. grayi can be distinguished using the mt-mutS barcode, the two lineages of P. grayi share the same haplotype for this barcode (Coelho et al. Citation2022). This highlights the need to further study genetic differentiation between P. clavata and P. grayi, as well as between the two segregating lineages of P. grayi at other mitochondrial genes. Here, we report the complete mitochondrial genome (mitogenome) of P. grayi assembled from RNA-seq read data generated in Coelho et al. (Citation2022). In total, we assembled complete/partial mitogenomes of nine individuals of P. grayi, including specimens of both the yellow (n = 7) and purple (n = 2) morphotypes. The samples were collected at three sites in southern (Sagres and Tavira) and western (Cape Espichel) Portugal. A specimen of each color morphotype are deposited at the Biogeographical Ecology and Evolution team collection at the Centre of Marine Sciences (email: [email protected]) under voucher IDs 19-0054 and 19-0046. The mitogenomes of P. clavata collected in the Mediterranean (Spain, Italy and Croatia; Coelho et al. Citation2022) and of P. biscaya from the Gulf of Mexico (see DeLeo et al. Citation2018) were also assembled for phylogenetic analysis (see below). For additional information about the origin and collection of samples, as well as about RNA extraction and sequencing see Gómez-Gras et al. (in review), Coelho et al. (Citation2022) and Supplemental Materials Section S1. Quality-filtered, ribosomal RNA-free read data for the samples from Coelho et al. (Citation2022) used here are deposited on NCBI's Sequence Read Archive (BioProject ID: PRJNA847883).
Figure 1. Colonies of Paramuricea grayi belonging to the purple (A) and yellow (B) morphotypes corresponding to the segregating lineages identified in Coelho et al. (Citation2022). Colonies were photographed at two distinct sites off Cape Espichel in western Portugal. The colonies of both color morphs tend to have relatively thin branches compared to the Mediterranean sister species P. clavata (though colonies with thick branches can also be observed in P. grayi, especially in the purple morphotype) and change color when dried or preserved (e.g. in etOH 96%), turning into a black/dark brown coloration. Photo credits: A.C. Ferreira.
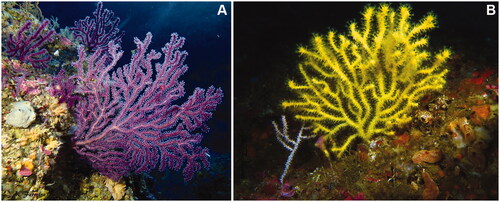
The mitogenomes were assembled with MITGARD (Mitochondrial Genome Assembly from RNA-seq Data) using default parameters (Nachtigall et al. Citation2021). MITGARD is an automated pipeline that allows mitochondrial sequence reads to be retrieved from RNA-seq data using a reference mitogenome as bait to subsequently generate de novo contigs and a consensus mitogenome assembly. We used the mitogenome sequence of P. clavata as a reference (Genbank Accession: NC_034749). The assembled mitogenomes were then visually curated by examining the alignment files of mapped reads, as well as of the assembled contigs and consensus mitogenome, onto the P. clavata reference in Geneious Prime v2022.0.1. This analysis revealed sequence incongruencies for some specimens in regions with consistently low read coverage across samples (e.g. regions spanning the 3'-end of cox1 and rnl; see Supplemental Materials, Section S2). For this reason and due to widespread (virtual) lack of intra-population polymorphism at mitochondrial genes in Octocorallia (e.g. Calderón et al. Citation2006; Hooper et al. Citation2021), the assembled mitogenomes of specimens with the highest read coverage were validated by comparison against the population consensus sequences (P. clavata: VAC, ALT and BALU; P. grayi: BAL; see Section S1 for details on population codes), as well as against a mitogenome assembled with long-read sequencing data for P. grayi (Costa et al. unpublished data). The assembled mitogenomes of both P. grayi and P. clavata were annotated using MITOS2 (Donath et al. Citation2019). Genes that were not identified (only rns), as well as gene boundaries in disagreement with available references (e.g. cox1 and rnl) were manually added in Geneious Prime. Finally, we performed a maximum likelihood (ML) phylogenetic analysis in IQ-TREE 2 (Minh et al. Citation2020) based on the mitogenomes of multiple octocorals (for details see Supplemental Materials, Section S3); and calculated the rate of synonymous (dS) and non-synonymous (dN) substitutions for protein-coding genes between P. grayi and P. clavata (NC_034749) using the online platform PAL2NAL (Suyama et al. Citation2006).
The complete circular mitogenome of P. grayi is 18,668 bp in length, has an A + T-rich base composition (62.5%) and contains the 17 genes typically found in Octocorallia: 14 protein-coding genes, including 13 energy pathway proteins (atp6, atp8, cob, cox1-3, nad1-6, and nad4L) and the mitochondrial homologue of the DNA mismatch repair MutS-like protein (mt-mutS); the small and large subunit rRNAs, rns and rnl, respectively; and one transfer RNA (trnM; methionine) (). Like other members of family Paramuriceidae (formerly Plexauridae; see McFadden et al. Citation2022), P. grayi has the presumed ancestral octocoral gene order A (Brockman and McFadden Citation2012), with 12 genes encoded in the heavy strand (cox1-rns-nad1-cob-nad6-nad3-nad4L-mt-mutS-rnl-nad2-nad5-nad4) and 5 genes in the light strand (trnM-cox3-atp6-atp8-cox2). In total, the intergenic regions (IGR) accounted for 618 bp of the mitogenome, ranging between 5 bp (rns-nad1 and rnl-nad2 IGRs) and 112 bp (cox2-cox1 IGR). The gene boundaries of nad2 and nad5 overlapped by 12 bp (; Supplemental Materials, Section S4). The partial/complete mitogenome sequences of P. grayi were nearly identical for all nine individuals, including across the two segregating color morphotypes. Notable exceptions included low-confidence regions with low/no read coverage (Section S2); one single nucleotide polymorphism (SNP) in nad6 for one of the seven individuals of the yellow lineage (BAL_8; 439 X coverage); and two SNPs 9 bp apart in mt-mutS for one of the two purple individuals (59X and 56X coverage), a polymorphism shared with P. clavata.
Figure 2. Circular map of the complete mitochondrial genome (i.e. mitogenome) of Paramuricea grayi (sample BAL_3; Sequence Read Archive (SRA) and GenBank accessions: SRR19977454 and SRR19977458, and OP205061, respectively). Note that there are two SRA accession numbers that correspond to RNA-seq read data from the same specimen sampled at two distinct time points during a heat-stress experiment. Arrows indicate the direction of transcription, with 12 genes encoded in the heavy strand (cox1-rns-nad1-cob-nad6-nad3-nad4L-mt-mutS-rnl-nad2-nad5-nad4) and 5 genes in the light strand (trnM-cox3-atp6-atp8-cox2). The genome is 18,668 bp in length and has the presumed ancestral octocoral gene order A (Brockman and McFadden Citation2012). The lower panel shows read coverage along the linearized reference mitogenome of P. clavata (Genbank Accession: NC_034749) obtained with MITGARD for the mitogenome assembly of sample BAL_3. Note that only a subset of the genes are labeled in the linearized graph. The average coverage was 3089X (range: 3–41,870X). For additional information about gene boundaries see Supplemental Materials, Section S4.
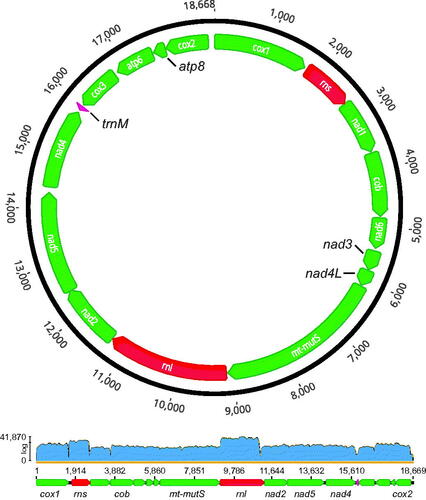
Like previous studies, our ML analysis based on mitogenome sequence data show that P. grayi and P. clavata are recently diverged sister species (Poliseno et al. Citation2017; Coelho et al. Citation2022; Quattrini et al. Citation2022; ). The mitogenomes of the two species have a pairwise sequence identity of 99.0%, with 87.2% of those mutations in protein-coding genes. The dS, a proxy for the mutation rate, ranged between 0.0000 (atp8) and 0.0602 (cox3), with the cox1 and mt-mutS genes, which encompass the target regions commonly employed for animal and octocoral barcoding, respectively, showing similar dS values (0.0385 vs. 0.0425, respectively; Supplemental Materials, Section S5). In contrast, none of the mutations observed in cox1 resulted in amino acid replacements, whereas mt-mutS had 12 non-synonymous mutations (dN = 0.0058). Surprisingly, for the P. grayi-P. clavata comparison nad6 had the highest dN of all protein-coding genes (0.0071; Section S4), and together with cox2 the highest dN/dS ratios (Section S4; for a comprehensive overview of the evolution of mitochondrial protein-coding genes in octocorals see Muthye et al. Citation2022). With the rapid expansion of next- and third-generation sequencing projects, data from RNA-seq represent an underused, but valuable resource to assemble octocoral mitochondrial genomes and more broadly to study the evolution of the eukaryotic powerhouse.
Figure 3. Phylogenetic relationships in 24 octocorals determined by maximum likelihood partition analysis in IQ-TREE 2. The analysis included species from seven families within order Alcyonacea (soft corals and gorgonians). The tree was built using the alignments of 14 mitochondrial protein-coding genes with a separate evolutionary model per gene (for details see Supplemental Materials, Section S3). Branches with full ultrafast bootstrap support (1000 replicates) are indicated by circles, with lower values shown on the branches. Mitogenomes assembled here are shown in bold, with gradient boxes highlighting the specimens of the purple and yellow morphotypes of Paramuricea grayi and the sister species Paramuricea clavata. Note that the mitogenome sequence of the purple individual P39_54 differed from the remaining eight P. grayi mitogenomes assembled (including another individual of the purple morphotype) by two polymorphisms located in the mt-mutS gene (9 bp apart), which are also found in P. clavata. Close inspection of read data in other specimens revealed multiple reads containing these two SNPs in the other purple individual (P39_57) despite of the consensus sequence matching the SNP pattern found in the yellow lineage of P. grayi. Note that the two SNPs identified in the mt-mutS are located downstream of the region of this gene commonly used to barcode octocorals. Compared to the reference available on Genbank, the P. clavata mitogenomes assembled here differed from the reference in four polymorphisms, one SNP in the mt-mutS, as well as a 1 bp deletion in the rnl and two SNPs in nad6 also observed in all of the P. grayi mitogenomes. Accession numbers of sequences retrieved from NCBI GenBank or Sequence Read Archive (SRA) are embedded in the taxon names, including P. biscaya for which the mitogenome was assembled here using RNA-seq data generated previously by DeLeo et al. (Citation2018). GenBank accession numbers for the complete mitogenome sequences of P. clavata (sample VAC_1) and P. grayi (sample BAL_3) are OP205061 and OP205062, respectively. The partial/complete sequences of the remaining mitogenome assemblies, including the purple individual P39_54 used in the phylogeny are deposited in Figshare (DOI: 10.6084/m9.figshare.20526978). For additional information about SRA accessions of raw read data for each sample, see Supplemental Materials Section S1.
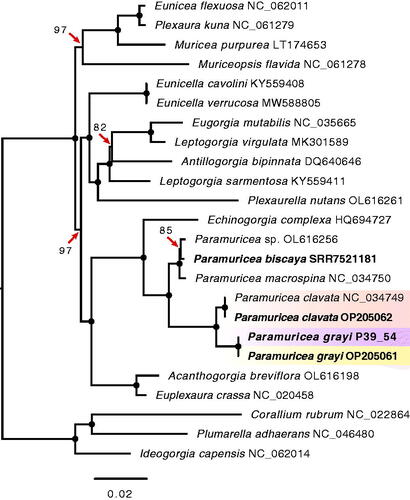
Author contributions
M.C., J.-B.L., J.G, E.S., and G.P. conceived the study. M.C., J.-B.L., J.B., D.P., D.G.-G., N.B., P.L.-S., C.C., S.K., and T. B.-P. contributed to data acquisition, including sample collection of the original study. M.C. and G.P analyzed the data. J.G. and E.S. contributed funding. M.C. drafted the manuscript. All authors critically revised the content of the manuscript, gave their approval for publication of the final version and agreed to be accountable for all aspects of the work.
Supplemental Material
Download MS Word (24.1 MB)Acknowledgements
For a full overview of acknowledgements regarding help provided during lab work and field collections of samples used here see Coelho et al. (Citation2022). The authors thank C. Linares and E. Ferretti for assistance collecting data, and A.C. Ferreira for providing photographs of P. grayi.
Disclosure statement
No potential conflict of interest was reported by the author(s).
Data availability statement
Raw RNA-seq read data are available at NCBI's SRA database (BioProject ID: PRJNA847883). The complete assembled mitogenomes of P. grayi (specimen BAL_3; Biosample [SRA] accessions: SAMN28899305 [SRR19977458] and SAMN28899308 [SRR19977454]) and P. clavata (specimen VAC_1; Biosample accessions: SAMN28899326 [SRR19977435] and SAMN28899323 [SRR19977438]) have been submitted to GenBank under accession numbers OP205061 and OP205062, respectively. Partial/complete mitogenome sequences of all the assemblies performed here (including P. biscaya) are available on Figshare (DOI: 10.6084/m9.figshare.20526978).
Additional information
Funding
References
- Altuna A. 1991. On the presence of Paramuricea grayi (Johnson, 1861) (Cnidaria, Anthozoa), in the Basque Coast. Munibe. 43:85–90.
- Brockman SA, McFadden CS. 2012. The mitochondrial genome of Paraminabea aldersladei (Cnidaria: Anthozoa: Octocorallia) supports intramolecular recombination as the primary mechanism of gene rearrangement in octocoral mitochondrial genomes. Genome Biol Evol. 4(9):994–1006.
- Calderón I, Garrabou J, Aurelle D. 2006. Evaluation of the utility of COI and ITS markers as tools for population genetic studies of temperate gorgonians. J Exp Mar Biol Ecol. 336(2):184–197.
- Coelho MAG, Pearson G, Boavida J, Paulo D, Aurelle D, Arnaud-Haond S, Gómez-Gras D, Bensoussan N, López-Sendino P, Cerrano C, et al. 2022. Not out of the Mediterranean: Atlantic populations of the gorgonian Paramuricea clavata are a separate sister species under further lineage diversification. Authorea. DOI: 10.22541/au.165544523.32209087/v1
- DeLeo DM, Herrera S, Lengyel SD, Quattrini AM, Kulathinal RJ, Cordes EE. 2018. Gene expression profiling reveals deep-sea coral response to the Deepwater Horizon oil spill. Mol Ecol. 27(20):4066–4077.
- Dias V, Oliveira F, Boavida J, Serrão EA, Gonçalves JMS, Coelho MAG. 2020. High coral bycatch in bottom-set gillnet coastal fisheries reveals rich coral habitats in Southern Portugal. Front Mar Sci. 7:1.
- Donath A, Jühling F, Al-Arab M, Bernhart SH, Reinhardt F, Stadler PF, Middendorf M, Bernt M. 2019. Improved annotation of protein-coding genes boundaries in metazoan mitochondrial genomes. Nucleic Acids Res. 47(20):10543–10552.
- Gómez-Gras D, Bensoussan N, Ledoux JB, López-Sendino P, Cerrano C, Ferretti E, Bakran-Petricioli SK, Cruz T, Gómez-Garrido F, Alioto J, et al. in review. Exploring the response of a key Mediterranean habitat-forming gorgonian to heat stress across biological and spatial scales. Sci Rep.
- Grasshoff M. 1977. Die Gorgonarien des östlichen Nordatlantik und des Mittelmeeres III. Die Familie Paramuriceidae (Cnidaria, Anthozoa). Meteor Forsch-Ergebnisse. 27:5–76.
- Grasshoff M. 1992. Die Flachwasser-Gorgonarien von Europa und Westafrika (Cnidaria, Anthozoa). Courier Forsch-Inst Senckenberg. 149:1–135.
- Hooper L, Jenkins TL, Griffiths AM, Moore KA, Stevens JR. 2021. The complete mitochondrial genome of the pink sea fan, Eunicella verrucosa (Pallas, 1766). Mitochondrial DNA B Resour. 6(11):3309–3311.
- McFadden CS, van Ofwegen LP, Quattrini AM. 2022. Revisionary systematics of Octocorallia (Cnidaria: Anthozoa) guided by phylogenomics. B Soc Syst Biolog. 1:8735.
- Minh BQ, Schmidt HA, Chernomor O, Schrempf D, Woodhams MD, Haeseler AV, Lanfear R, Teeling E. 2020. IQ-TREE 2: new models and efficient methods for phylogenetic inference in the genomic era. Mol Biol Evol. 37(5):1530–1534.
- Muthye V, Mackereth CD, Stewart JB, Lavrov DV. 2022. Large dataset of octocoral mitochondrial genomes provides new insights into mt-mutS evolution and function. DNA Repair. 110:103273.
- Nachtigall PG, Grazziotin FG, Junqueira-De-Azevedo ILM. 2021. MITGARD: an automated pipeline for mitochondrial genome assembly in eukaryotic species using RNA-seq data. Brief Bioinform. 22(5):1–15.
- Nestorowicz I-M, Oliveira F, Monteiro P, Bentes L, Henriques NS, Aguilar R, Costa BE, Gonçalves JMS. 2021. Identifying habitats of conservation priority in the São Vicente Submarine Canyon in Southwestern Portugal. Front Mar Sci. 8:672850.
- Poliseno A, Altuna A, Cerrano C, Wörheide G, Vargas S. 2017. Historical biogeography and mitogenomics of two endemic Mediterranean gorgonians (Holaxonia, Plexauridae). Org Divers Evol. 17(2):365–373.
- Quattrini AM, Herrera S, Adams JM, Grinyó J, Louise AA, Shuler A, Wirshing HH, Cordes EE, McFadden CS. 2022. Phylogeography of Paramuricea: the role of depth and water mass in the evolution and distribution of deep-sea corals. Front Mar Sci. 9:849402.
- Suyama M, Torrents D, Bork P. 2006. PAL2NAL: robust conversion of protein sequence alignments into the corresponding codon alignments. Nucleic Acids Res. 34(Web Server issue):W609–W612.
- Thoma J. 2013. [Molecular and morphological diversity of sea fans with emphasis on deep-sea octocorals of the order Alcyonacea Lamouroux, 1812] [PhD dissertation]. Lafayette (LA): University of Louisiana Lafayette.
- Walting L, France SC, Pante E, Simpson A. 2011. Chapter 2: biology of deep-water octocorals. In: Lesser MP, editors. Advances in marine biology. Vol. 60. London: Elsevier Ltd; p. 41–122.