Abstract
Zannichellia palustris Linnaeus 1753 is a cosmopolitan submerged species capable of rapidly responding to environmental changes, with potential applications in the ecological treatment of heavy metal pollution in water bodies. This study aimed to characterize the complete chloroplast genome of Z. palustris, which has not been reported previously. The chloroplast genome of Z. palustris displays a quadripartite structure with a length of 155,262 base pairs (bp), comprising a large single copy (LSC) region of 85,397 bp, a small single copy (SSC) region of 18,057 bp, and a pair of inverted repeat (IR) regions of 25,904 bp. The GC content of the genome is 35.8%, with corresponding values of 33.4% for the LSC, 28.2% for the SSC, and 42.5% for the IR regions. The genome contained 130 genes, including 85 protein-coding genes, 37 tRNA genes, and eight rRNA genes. Phylogenetic analysis within the order Alismatales revealed that Z. palustris clusters with the clade of Potamogeton perfoliatus, P. crispus and Stuckenia pectinata.
Introduction
Zannichellia palustris Linnaeus 1753 is a submerged perennial herb that grows in various shallow-water habitats with fresh or brackish water worldwide (Guo et al. Citation2010). It can respond quickly to changes in its habitat (Crow Citation1979) and accumulate copper, nickel, zinc, cadmium and silver (Bielmyer-Fraser et al. Citation2022), indicating a high potential for Z. palustris in bio-monitoring and phytoremediation of heavy metals in water. The taxonomic classification of the genus Zannichellia has been contentious. It was once classified under the family Najadaceae (Zhou et al. Citation1992) but was later treated as an independent family, Zannichelliaceae (Guo et al. Citation2010). In APG IV (2016), it was placed in the family Potamogetonaceae based on molecular data. However, the chloroplast genome of Zannichellia species has not been described until now. In this study, we report the complete chloroplast genome sequence of Z. palustris, to provide a useful resource for the genus Zannichellia and confirm its phylogenetic position.
Materials and methods
Samples of Z. palustris were collected from a pond 800 m north of Caowotan Town, Jingtai County, Baiyin, Gansu, China (37.3172°N, 104.1228°E) (). A specimen was deposited at the herbarium of Wuhan University (contact person: Xinwei Xu, [email protected]) under the voucher number GS2022091001. Z. palustris is not a rare species for protection. No permission is required to collect the plant samples. Experimental research and field studies on plants comply with relevant institutional, national, and international guidelines and legislation. Total genomic DNA was extracted with the DNA SECURE PLANT KIT (Tiangen Biotech, Beijing, China).
Figure 1. Species reference image of Zannichellia palustris. (A) Plant of Z. palustris. (B) Fruits of Z. palustris. Photos attributed to Jinwang Wang from Zhejiang Academy of Agricultural Sciences and used by his permission.
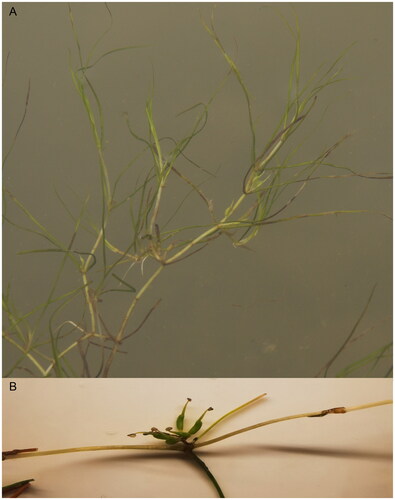
Library preparation and genome sequencing were performed on the Illumina HiSeq2500 platform (Novogene Biotech, Beijing, China). The raw data were filtered using Trimmomatic v0.38 (Bolger et al. Citation2014) and then a total of 2.66 GB clean reads were obtained. GetOrganelle was used to assemble the complete chloroplast genome (Jin et al. Citation2020). The accuracy and completeness of the chloroplast genome were assessed using Geneious Prime (https://www.geneious.com). With the reference chloroplast genome of Stuckenia pectinata (NC_057253.1) (Tian et al. Citation2019), the assembled genome was annotated by CPGAVAS2 (http://www.herbalgenomics.org/cpgavas2) (Shi et al. Citation2019). The package CPGView (http://www.1kmpg.cn/cpgview) (Liu et al. Citation2023) was used to visualize detailed chloroplast genome structures, examine genome annotation and identify genes with complex structure, including cis-splicing genes and trans-splicing genes. The obtained complete chloroplast genome of Z. palustris was subsequently submitted to GenBank (accession number: OP748246). Simple sequence repeat (SSR) loci in the chloroplast genome were identified using MISA (https://webblast.ipk-gatersleben.de/misa/) (Beier et al. Citation2017). The minimum number of repeats for mononucleotide, dinucleotide, trinucleotide, tetranucleotide, and pentanucleotide was set to 10, 5, 4, 4, and 4, respectively.
To confirm the phylogenetic position of Z. palustris within the order Alismatales, we generated a maximum-likelihood (ML) phylogenetic tree based on 23 chloroplast genomes obtained from the GenBank (Table S1). Multiple sequence alignment was performed using MAFFT v7.149 (Katoh et al. Citation2002). The best-fit model of nucleotide substitution was estimated by Modeltest v3.7 (Posada and Crandall Citation1998). The ML analysis was performed using MEGA X (Kumar et al. Citation2018) and 1000 repetitions were performed to summarize the ML bootstrap support.
Results
The coverage of the genome from paired-end reads was 100%, with an average depth of 2217.4× (Figure S1), indicating that the chloroplast genome of Z. palustris was assembled correctly. The complete chloroplast genome of Z. palustris is 155,262 bp in length and has a quadripartite structure (), including a large single copy (LSC) region of 85,397 bp, a small single copy (SSC) region of 18,057 bp, and a pair of inverted repeat (IR) regions of 25,904 bp. The GC content of the genome is 35.8%, with corresponding values of 33.4%, 28.2%, and 42.5% for the LSC, SSC, and IR regions, respectively. The chloroplast genome contains 130 genes, including 85 protein-coding genes, 37 tRNA genes, and eight rRNA genes. A total of 22 genes contain introns, with ycf3 and clpP containing two introns each. Thirteen cis-splicing genes and the trans-splicing gene rps12 were identified, and their structures are shown in Figure S2. Thirty-one SSRs were identified, including 28 mononucleotides (A/T, 48.16%/48.16%), two dinucleotides (AT/TA, 3.22%/3.22%), and one trinucleotide (ATA, 3.22%).
Figure 2. Genetic map of the chloroplast genome of Zannichellia palustris. The map consists of several circles, each with the following information from the center outward: the circles closest to the center are indicated by red and green arcs for forward and reverse repeats, respectively. The second and third circles are indicated by short bars for tandem repeats and microsatellite sequences, respectively. The fourth circle indicates the positions of the LSC, SSC, IRA, and IRB regions, respectively. The fifth circle indicates the GC content. The outer circle indicates the function of the gene. Different colors are used to show different functional categories, as shown in the lower left of the picture.
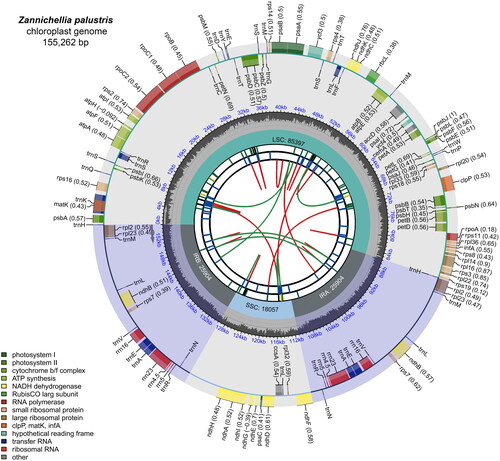
Figure 3. The ML phylogenetic tree based on the complete genome sequences of Zannichellia palustris and 22 other species within the order Alismatales. The position of Z. palustris is highlighted in bold and numbers next to each node are bootstrap support. The used sequences and their references were listed in Table S1.
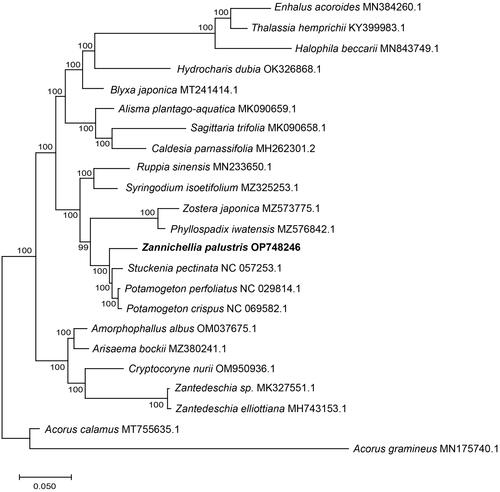
The best-fit model of nucleotide substitution was GTR + F+R3. The phylogenetic tree showed that Z. palustris formed a separate branch and clustered with the clade of Potamogeton perfoliatus, P. crispus and Stuckenia pectinata with full support.
Discussion and conclusion
The chloroplast genome of Z. palustris possesses the typical quadripartite structure, and its size and gene number are similar to those of two closely related species, P. perfoliatus (156,226 bp and 130 genes, Luo et al. Citation2016) and S. pectinata (156,669 bp and 131 genes, Tian et al. Citation2019). This is the first reported chloroplast genome in the genus Zannichellia. There are six accepted species within Zannichellia and three subspecies of Z. palustris worldwide (POWO Citation2023). Due to the variation in the shape of the stigma and the characteristics of the fruit, it is difficult to identify species of Zannichellia based on morphological characteristics alone (Guo et al. Citation2010). Therefore, our results provide an essential genetic resource for the taxonomic and related studies of Zannichellia.
Authors’ contributions
Lei Huang carried out the experiments, performed the data analysis and drafted the manuscript. Xinwei Xu designed the research, carried out the field collection and revised the manuscript critically for important intellectual content. Both authors approved the final manuscript.
Supplemental Material
Download MS Word (19.2 KB)Supplemental Material
Download MS Word (265.8 KB)Disclosure statement
No potential conflict of interest was reported by the authors.
Data availability statement
The genome sequence data that support the findings of this study are openly available in GenBank of NCBI at https://www.ncbi.nlm.nih.gov/ under the accession no. OP748246. The associated BioProject, SRA, and BioSample numbers are PRJNA916656, SRR22924206, and SAMN32489662, respectively.
Additional information
Funding
References
- Beier S, Thiel T, Munch T, Scholz U, Mascher M. 2017. MISA-web: a web server for microsatellite prediction. Bioinformatics. 33(16):2583–2585.
- Bielmyer-Fraser G, Llazar K, Ward A, Trent T, Goldberg N. 2022. Metal analysis of submerged aquatic vegetation in the lower St. Johns River, Florida. Environ Monit Assess. 194(7):492.
- Bolger AM, Lohse M, Usadel B. 2014. Trimmomatic: a flexible trimmer for Illumina sequence data. Bioinformatics. 30(15):2114–2120.
- Crow JH. 1979. Distribution and ecological characteristics of Zannichellia palustris L. along the Alaska Pacific Coast. Bull Torrey Bot Club. 106(4):346–349.
- Guo Y, Haynes RB, Hellquist CB. 2010. Zannichelliaceae. In: Wu Z, Raven PH, Hong D. (Eds.) Flora of China Vol. 23. Beijing: Science Press; St. Louis: Missouri Botanical Garden Press; , p. p116.
- Jin J, Yu W, Yang J, Song Y, DePamphilis CW, Yi T, Li D. 2020. GetOrganelle: a fast and versatile toolkit for accurate de novo assembly of organelle genomes. Genome Biol. 21(1):241.
- Katoh K, Misawa K, Kuma K, Miyata T. 2002. MAFFT: a novel method for rapid multiple sequence alignment based on fast Fourier transform. Nucleic Acids Res. 30(14):3059–3066.
- Kumar S, Stecher G, Li M, Knyaz C, Tamura K. 2018. MEGA X: molecular evolutionary genetics analysis across computing platforms. Mol Biol Evol. 35(6):1547–1549.
- Liu S, Ni Y, Li J, Zhang X, Yang H, Chen H, Liu C. 2023. CPGView: a package for visualizing detailed chloroplast genome structures. Mol Ecol Resour. 23(3):694–704.
- Luo Y, Ma P, Li H, Yang J, Wang H, Li D. 2016. Plastid phylogenomic analyses resolve Tofieldiaceae as the root of the early diverging monocot order Alismatales. Genome Biol Evol. 8(3):932–945.
- Posada D, Crandall KA. 1998. MODELTEST: testing the model of DNA substitution. Bioinformatics. 14(9):817–818.
- POWO 2023., Plants of the World Online. Facilitated by the Royal Botanic Gardens, Kew. Published on the Internet; http://www.plantsoftheworldonline.org/. Retrieved 01 April 2023.
- Shi L, Chen H, Jiang M, Wang L, Wu X, Huang L, Liu C. 2019. CPGAVAS2, an integrated plastome sequence annotator and analyzer. Nucleic Acids Res. 47(W1):W65–W73.
- Chase MW, Christenhusz MJM, Fay MF, Byng JW, Judd WS, Soltis DE, Mabberley DJ, Sennikov AN, Soltis PS, Stevens PF, The Angiosperm Phylogeny Group 2016. An update of the angiosperm phylogeny group classification for the orders and families of flowering plants: APG IV. Bot J Linn Soc. 181:1–20.
- Tian J, Zhang X, Liu Z, Shen S. 2019. Characterization of the complete chloroplast genome sequence of submerged macrophyte Stuckenia pectinata (Potamogeonaceae) and its phylogenetic position. Mitochondrial DNA Part B Resour. 5(1):327–328.
- Zhou L, You J, Zhong X. 1992. Zannichellia. In: Sun X, ed., Fl. Reipubl. Popularis Sin. 8:p. 102–107. Beijing: Science Press.