Abstract
Here, the complete chloroplast genome sequence of Zingiber teres is described using MGI paired-end sequencing. The genome is 163,428 bp in length and contains a small single-copy region (SSC) of 15,782 bp, a large single-copy region (LSC) of 88,142 bp, and two inverted repeat (IR) regions of 29,752 bp. The overall GC content is 36.1%, and the GC content of the IR regions is 41.1%, which is higher than that of both the LSC region (33.8%) and SSC region (29.5%). The genome of Z. teres contains 133 complete genes, including 88 protein-coding genes (79 protein-coding gene species), 38 tRNA genes (28 tRNA species), and 8 rRNA genes (four rRNA species). Maximum likelihood phylogenetic analysis yielded a well-resolved tree of the genus Zingiber, and Z. teres and Zingiber mioga were sister species in this tree. The development of DNA barcodes could aid the identification of Zingiber species.
Introduction
Zingiber teres S. Q. Tong & Y. M. Xia (Tong and Xia Citation1987) is a member of the genus Zingiber in the family Zingiberaceae. Z. teres occur at high elevations in hill evergreen forests in southern China and northern and northeastern Thailand (Tong and Xia Citation1987; Triboun et al. Citation2007). The height of the slender stem of Z. teres ranges from 60 to 100 cm; Z. teres can be distinguished from other Zingiber species by the length of the ligule (2–4 mm) and petiole (ca. 2 mm) (Wu and Larsen Citation2000). The rhizome of Z. teres is cylindrical and has an aromatic odor similar to that of other ginger species. The elliptic inflorescences arise from rhizomes with peduncles embedded in the ground. Z. teres has several features that would potentially make it an excellent ornamental plant. Zingiber plants are difficult to identify because of their short and seasonal flowering cycles, the high degree of phenotypic plasticity in many of their traits, and their high morphological similarity (Theerakulpisut et al. Citation2012; Basak Citation2014). DNA barcoding is an effective technique for the identification of species (Hebert et al. Citation2003; Chen et al. Citation2010). Divergent hotspots that could be used for the identification of species in the subfamily Zingiberoideae have been previously identified (Li et al. Citation2021a, Citation2021b). The chloroplast genome of Z. teres has not yet been described. Here, we sequenced and assembled the whole chloroplast genome of Z. teres. This chloroplast genome will enhance our understanding of the population genetics of Z. teres as well as the evolutionary history of the genus Zingiber.
Materials
The Z. teres () material sequenced in this study was collected from Laiyanghe Park in Puer, Yunnan Province, China (22.58°N, 101.06°E). This species is common in southwest Yunnan and can be collected without permits. Fresh and healthy leaves were dried with silica gel and preserved for DNA extraction. A specimen was deposited at the Herbarium of Chongqing University of Arts and Sciences (www.cqwu.net, Huamin Liu, [email protected]) under the voucher number Li_Z032.
Methods
Total genomic DNA was extracted from silica gel-dried leaves using the modified sucrose gradient centrifugation method (Li et al. Citation2012). A NanoDrop 2000 micro spectrometer (Wilmington, DE, USA) was used to evaluate the concentration of isolated genomic DNA; the genomic DNA was then stored in a −80 °C refrigerator at the Key Laboratory of the College of Landscape Architecture and Life Science. The MGIEasy PCR-Free DNA Library Prep kit (MGI-TECH) was used to generate the paired-end DNA library with an insert size of 350 bp; the MGI DNBSEQ-T7 platform (MGI-TECH, Shenzhen, China) was then used to sequence the library. A total of 5.5 G raw data were produced. Clean data were obtained by removing the adaptor and low-quality sequences. The software NOVOPlasty3.5 (Dierckxsens et al. Citation2017) was used to de novo assemble the whole chloroplast genome; the k-mer length was 39 bp, and a fragment of the rbcL gene from Zingiber officinale was used as the seed sequence. Geneious v11.0.4 was used to annotate and manually correct the chloroplast genome using the Z. officinale (MW602894) genome as the reference genome sequence. The circular chloroplast genome maps of Z. teres were built using the OGDRAW v1.3.1 program with default settings.
A phylogeny of the genus Zingiber was built using the chloroplast genome sequence of Z. teres, along with those of seven other species, which were obtained from GenBank: Zingiber recurvatum, Zingiber mioga, Z. officinale, Zingiber montanum, Zingiber corallinum, Zingiber spectabile, and Zingiber zerumbet. Kaempferia gaalanga was used as the outgroup in the phylogenetic analysis. MAFFT was used to align the complete plastome sequences with a gap opening penalty of 2–2.5 and a gap extension penalty of 0. Phylogenetic trees based on these aligned sequences were built using the maximum likelihood (ML) method under the GTRGAMMA model; branch support was evaluated using 1000 bootstrap replicates. The software Geneious R11 was used to conduct the above analyses.
Results
The chloroplast genome of Z. teres was 163,428 bp () and contained a small single-copy (SSC) region of 15,782 bp, a large single-copy (LSC) region of 88,142 bp, and two inverted repeat (IR) regions of 29,752 bp. The overall GC content was 36.1%; the GC content of the IR regions, LSC region, and SSC region was 41.1%, 33.8%, and 29.5%, respectively. The sequenced chloroplast genome contained 133 predicted functional genes, including 88 protein-coding genes (79 protein-coding gene species), 38 tRNA genes (28 tRNA species), and 8 rRNA genes (four rRNA species). (). In total, 10 cis-splicing genes including rps16, atpF, rpoC1, ycf3, clpP, petB, petD, rpl16, rpl2, ndhB and ndhA (Figure S2A), and one trans-splicing genes rps12 (Figure S2B) were detected by CPGview (Liu et al. Citation2023) (http://www.1kmpg. cn/cpgview/).
Figure 2. Map of the whole Z. teres chloroplast genome. Genes marked outside the circle are transcribed counterclockwise, and genes marked inside the circle are transcribed clockwise. The gray arrow indicates gene orientation. The tRNA genes are indicated by the one-letter code of amino acids with anticodons. LSC, large single-copy region; IR, inverted repeat; SSC, small single-copy region. Genes belonging to different functional groups are shaded differently. The innermost first ring in black indicates the chloroplast genome size of Z. teres. The innermost darker gray indicates the GC content, and the lighter gray indicates the at content.
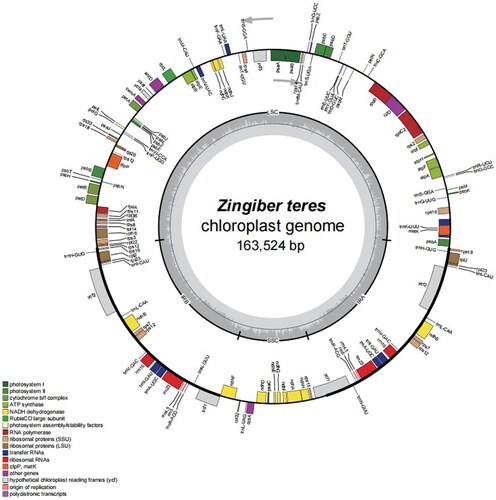
We generated a well-resolved phylogeny of Zingiber using a chloroplast genome dataset (). The bootstrap support values of all the branches were greater than 99%. Thus, the support values are not provided in the text below. Zingiber was divided into two main clades. Clade I comprising Z. recurvatum was sister to Z. mioga + Z. teres; clade II including Z. officinale was sister to (Z. montanum + Z. corallinum) + (Z. spectabile + Z. zerumbet).
Figure 3. Maximum likelihood (ML) analysis of Z. teres and other related species based on the complete chloroplast genome sequence. The following sequences were used: Z. teres (OM868074), Z. recurvatum (MT473712; Li et al. Citation2021a), Z. mioga (NC_057615; Jiang et al. Citation2023), Z. officinale (MW602894; Jiang et al. Citation2023), Z. montanum (MW801386; Tian et al. Citation2023), Z. corallinum (MW801385; Tian et al. Citation2023), Z. spectabile (NC_020363; Tian et al. Citation2023), Z. zerumbet (MK262726; Li et al. Citation2021a), and Kaempferia gaalanga (MK209001; Li et al. Citation2019a).
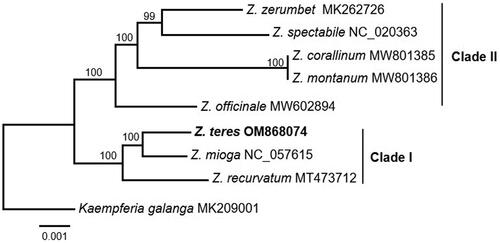
Discussion and conclusion
The chloroplast genome of Z. teres was reported for the first time in this study. The size (163,428 bp), GC content (36.1%), gene composition, quadripartite structure, protein-coding genes, tRNAs, and rRNAs of the Z. teres chloroplast genome were similar to those of other Zingiberoideae chloroplast genomes (Cui et al. Citation2019; Li et al. Citation2019a, Citation2019b). Many plants in families such as Malvaceae, Araceae, and Rhamnaceae have chloroplast genomes with the same gene content and gene order (Waseem et al. Citation2020; Henriquez et al. Citation2021; Mehmood et al. Citation2021a, Citation2021b). Genome variations such as plastome rearrangement, gene loss, intron loss, and gene duplication are common in plants (Lin et al. Citation2015; Daniell et al. Citation2016, Lee et al. Citation2020; Li et al. Citation2021a). The loss of both trnS-GGA and trnT-GGU has been reported in the chloroplast genome of Globba schomburgkii, which is in the family Zingiberoideae. The lhbA gene has been lost in both Hedychium coccineum and Hedychium neocarneum (Li et al. Citation2021a). However, the chloroplast genomes of Z. teres were highly conserved. Hence, specific molecular mechanisms might be responsible for the conservation of the Z. teres plastomes, including the rarity of plastid fusion, uniparental inheritance, and the presence of an active repair mechanism, as has been reported in previous studies (Wicke et al. Citation2011; Mehmood et al. Citation2021b).
Our phylogeny of Zingiber was well resolved, and all the branches were highly supported. The relationships among Zingiber species were similar to those inferred in previous studies (Theerakulpisut et al. Citation2012; Li et al. Citation2021a). Z. recurvatum was grouped with Z. teres + Z. mioga and sister to clade II. This result is consistent with a previously published molecular phylogeny in which Z. recurvatum was sister to the other Zingiber species (Li et al. Citation2021a; equivalent to clade II in this study). Our results showed that Z. zerumbet was sister to Z. spectabile with strong support. Similar relationships have also been observed in previous studies (Theerakulpisut et al. Citation2012; Li et al. Citation2021a). In general, the support values of each branch within our phylogeny of Zingiber were higher than those of a previously published phylogeny based on nuclear genome data (Theerakulpisut et al. Citation2012; Li et al. Citation2021a). These results indicate that the chloroplast genome has a sufficient number of informative sites to resolve the phylogenetic relationships among Zingiber species. The development of DNA barcodes could aid the identification of Zingiber species.
Author contributions
Hong-Lei Li and Haitao Xing conceived the study. Xiao Wang and Shuming Tian performed the experiments. Hong-Lei Li, Xiao Wang, Shuming Tian, Hao Wang, Lin Yang, Xavier-ravi Baskaran, Qiang Li and Xiaoling Zou contributed reagents/materials/analysis tools and analyzed the data. Hong-Lei Li, Xiao Wang and Haitao Xing wrote the paper. Hong-Lei Li, Haitao Xing, Qiang Li and Xavier-ravi Baskaran edited the paper.
Supplemental Material
Download MS Word (132.7 KB)Disclosure statement
No potential conflict of interest was reported by the authors.
Data availability statement
The genome sequence data that support the findings of this study are openly available in GenBank of NCBI at (https://www.ncbi.nlm.nih.gov/nuccore/OM868074) under the accession no. OM868074. The associated BioProject, SRA, and Bio-Sample numbers are PRJNA827347, SRR18899755, and SAMN27609002 respectively.
Additional information
Funding
References
- Basak S. 2014. [Molecular phylogeny of Zingiberoideae]. [PHD Thesis]. Indian institute of technology Guwahati, Assam, India.
- Chen SL, Yao H, Han JP, Liu C, Song JY, Shi LC, Zhu YJ, Ma XY, Gao T, Pang XH, et al. 2010. Validation of the ITS2 region as a novel DNA barcode for identifying medicinal plant species. PLOS One. 5(1):e8613. doi: 10.1371/journal.pone.0008613.
- Cui Y, Nie L, Sun W, Xu Z, Wang Y, Yu J, Song J, Yao H. 2019. Comparative and phylogenetic analyses of ginger (Zingiber officinale) in the family Zingiberaceae based on the complete chloroplast genome. Plants. 8(8):283. doi: 10.3390/plants8080283.
- Daniell H, Lin C-S, Yu M, Chang W-J. 2016. Chloroplast genomes: diversity, evolution, and applications in genetic engineering. Genome Biol. 17(1):29. doi: 10.1186/s13059-016-1004-2.
- Dierckxsens N, Mardulyn P, Smits G. 2017. NOVOPlasty: de novo assembly of organelle genomes from whole genome data. Nucleic Acids Res. 45(4):e18. doi: 10.1093/nar/gkw955.
- Hebert PDN, Cywinska A, Ball SL, deWaard JR. 2003. Biological identifications through DNA barcodes. Proc Biol Sci. 270(1512):313–321. doi: 10.1098/rspb.2002.2218.
- Henriquez, Claudia L, Mehmood, Furrukh, Hayat, Amir, Sammad, Abdul, Waseem, Shahid, Waheed, Mohammad Tahir, Matthews, Peter J, Croat, Thomas B, Poczai, Peter, Ahmed, Ibrar, Abdullah . 2021. Chloroplast genome evolution in the Dracunculus clade (Aroideae, Araceae). Genomics, 1 Pt 1. 113: 183–192. doi: 10.1016/j.ygeno.2020.12.016.
- Jiang D, Cai X, Gong M, Xia M, Xing H, Dong S, Tian S, Li J, Lin J, Liu Y, et al. 2023. Complete chloroplast genomes provide insights into evolution and phylogeny of Zingiber (Zingiberaceae). BMC Genomics. 24(1):30. doi: 10.1186/s12864-023-09115-9.
- Lee C, Ruhlman TA, Jansen RK. 2020. Unprecedented intraindividual structural heteroplasmy in Eleocharis (Cyperaceae, Poales) plastomes. Genome Biol Evol. 12(5):641–655. doi: 10.1093/gbe/evaa076.
- Li X, Hu Z, Lin X, Li Q, Gao H, Luo G, Chen S. 2012. High-throughput pyrosequencing of the complete chloroplast genome of Magnolia officinalis and its application in species identification. Acta Pharm Sin. 47:124–130.
- Li DM, Li J, Wang DR, Xu YC, Zhu GF. 2021a. Molecular evolution of chloroplast genomes in subfamily Zingiberoideae (Zingiberaceae). BMC Plant Biol. 21(1): 558. doi: 10.1186/s12870-021-03315-9.
- Li HL, Wu L, Dong Z, Jiang Y, Jiang S, Xing H, Li Q, Liu G, Tian S, Wu Z. 2021b. Haplotype-resolved genome of diploid ginger (Zingiber officinale) and its unique gingerol biosynthetic pathway. Hortic Res. 8:189.
- Li D-M, Zhao C-Y, Liu X-F. 2019a. Complete chloroplast genome sequences of Kaempferia galanga and Kaempferia elegans: molecular structures and comparative analysis. Molecules. 24(3):474. doi: 10.3390/molecules24030474.
- Li D-M, Zhao C-Y, Zhu G-F, Xu Y-C. 2019b. Complete chloroplast genome sequence of Hedychium coronarium. Mitochondrial DNA Part B Resour. 4(2):2806–2807. doi: 10.1080/23802359.2019.1659114.
- Lin C-S, Chen JJW, Huang Y-T, Chan M-T, Daniell H, Chang W-J, Hsu C-T, Liao D-C, Wu F-H, Lin S-Y, et al. 2015. The location and translocation of ndh genes of chloroplast origin in the Orchidaceae family. Sci Rep. 5(1):10. doi: 10.1038/srep09040.
- Liu S, Ni Y, Li J, Zhang X, Yang H, Chen H, Liu C. 2023. CPGView: a package for visualizing detailed chloroplast genome structures. Mol Ecol Resour. 23(3):694–704. doi: 10.1111/1755-0998.13729.
- Mehmood, Furrukh, Rahim, Abdur, Heidari, Parviz, Ahmed, Ibrar, Poczai, Péter, Abdullah . 2021b. Comparative plastome analysis of Blumea, with implications for genome evolution and phylogeny of Asteroideae.Ecol Evol, 12. 11: 7810–7826. doi: 10.1002/ece3.7614.
- Mehmood, Furrukh, Shahzadi, Iram, Ali, Zain, Islam, Madiha, Naeem, Muhammad, Mirza, Bushra, Lockhart, Peter J., Ahmed, Ibrar, Waheed, Mohammad Tahir, Abdullah . 2021a. Correlations among oligonucleotide repeats, nucleotide substitutions, and insertion-deletion mutations in chloroplast genomes of plant family Malvaceae.J. Syst. Evol. 59(2): 388–402. doi: 10.1111/jse.12585.
- Theerakulpisut P, Triboun P, Mahakham W, Maensiri D, Khampila J, Chantaranothai P. 2012. Phylogeny of the genus Zingiber (Zingiberaceae) based on nuclear ITS sequence data. Kew Bull. 67(3):389–395. doi: 10.1007/s12225-012-9368-2.
- Tian S, Jiang D, Wan Y, Wang X, Liao Q, Li Q, Li H-L, Liao L. 2023. The complete chloroplast genome of Zingiberstriolatum Diels (Zingiberaceae). Mitochondrial DNA B Resour. 8(1):48–51. doi: 10.1080/23802359.2022.2160218.
- Tong S-Q, Xia Y-M. 1987. New taxa of Zingiberaceae from southern Yunnan. Acta Phytotax Sin. 25:460–471.
- Triboun P, Chantaranothai P, Larsen K. 2007. Taxonomic changes regarding three species of Zingiber (Zingiberaceae) from thailand. Acta Phytotax Sin. 45(3):403–404.
- Waseem, Shahid, Mirza, Bushra, Ahmed, Ibrar, Waheed, Mohammad Tahir, Abdullah . 2020. Comparative analyses of chloroplast genomes of Theobroma cacao and Theobroma grandiflorum. Biologia, 5. 75: 15. doi: 10.2478/s11756-019-00388-8.
- Wicke S, Schneeweiss GM, Depamphilis CW, Müller KF, Quandt D. 2011. The evolution of the plastid chromosome in land plants: gene content, gene order, gene function. Plant Mol Biol. 76(3–5):273–297. doi: 10.1007/s11103-011-9762-4.
- Wu TL, Larsen K. 2000. Zingiberaceae. In: Wu ZY, Raven PH, Hong DY, eds. Flora of China, Vol. 24. Science Press, Beijing & Missouri Botanical Garden Press, St. Louis