Abstract
Sphaeropleales is an order of fast-growing microalgae with high oil content and high efficiency in sewage treatment, in which photosynthesis plays a critical role. We isolated a strain of Sphaeropleales, Chlorolobion braunii ITBB-AG6 from an azolla community in a sewage pond, and sequenced its chloroplast genome. The complete genome has a length of 154 kb with a GC content of 31.7%. A total of 89 genes were annotated, including 56 protein-coding genes, 30 tRNA genes, and three rRNA genes. Out of the protein coding genes, 64.3% are involved in photosynthesis, 28.6% are involved in protein synthesis, and 7.1% are involved in ATP synthesis. Transfer RNA genes for 20 amino acids were identified, in which tRNA genes for methionine, leucine, and arginine are tripled, whereas tRNA genes for glutamic acid, glycine, serine, and threonine are doubled. Terminal inverted repeats of 27.9 kb containing 10 genes related to photosynthesis and chloroplast division are present in the genome, suggesting that photosynthesis was strengthened in the evolutionary history. Phylogenetic analysis indicates that C. braunii ITBB-AG6 falls in the family Selenastraceae and is most closely related to Monoraphidium neglectum.
Introduction
Sphaeropleales is one of the most important orders in the class Chlorophyceae. It contains some common freshwater species (Fucikova et al. Citation2014), such as Ankistrodesmus falcatus (Corda) Ralfs, 1848 (Wang et al. Citation2020), Chlorolobion braunii (Naegeli) Komarek (Baracho et al. Citation2019), and Monoraphidium braunii (Nägeli ex Kützing) Komárková-Legnerová Citation1969 (Gattullo et al. Citation2012). These species have been used in applications such as bioassays, bioremediation, and biofuel production (Gorman and Levine Citation1965; Wang et al. Citation2020; El-Sheekh et al. Citation2023). However, Sphaeropleales is relatively poorly understood in terms of diversity and evolution, compared to its sister order Volvocales, which contains the versatile model species Chlamydomonas reinhardtii, and is often used in the investigation of the evolution of multicellularity (Fucikova et al. Citation2016). Chloroplast genome data are widely used to infer phylogenetic relationships of plants and algae, and complex patterns of sequence evolution were revealed in Sphaeropleales (Fucikova et al. Citation2016). This study reports the chloroplast genome of a Sphaeropleales species, C. braunii ITBB-AG6.
Materials
C. braunii strain ITBB-AG6 was isolated from an azolla community in a sewage treatment pond operated by Jiaming Zhang’s laboratory at the experimental station of the Institute of Tropical Bioscience and Biotechnology, CATAS in Danzhou City, Hainan Province, China (19.5211N, 109.5119E). Classification of the strain was performed by referring to the images of the type strains in the SAG algal stocks (https://www.uni-goettingen.de/en/184982.html) and the AlgaeBase (Guiry and Guiry Citation2018) (). For DNA isolation, the strain was cultured in TAP medium (Gorman and Levine Citation1965) and centrifuged to harvest the cells. A sample of the culture is stored at the ClonBank of the Institute of Tropical Bioscience and Biotechnology at −80 °C in 15% glycerol with the voucher number ITBB-AG6 (Curator, Deguan Tan, [email protected]).
Figure 1. The azolla-algae community and the algal cells. (A) Azolla-algae community; (B) wild algal cells from the community; algal cells grown in TAP medium and stained with Nile red, and observed under bright (C) and fluorescent light (D). Red arrow indicates the algal film in the gap between azolla plants; blue arrows indicate the oil droplets in the algal cells. (A, B) Taken by Jiaming Zhang; (C, D) taken by Yaojia Mu.
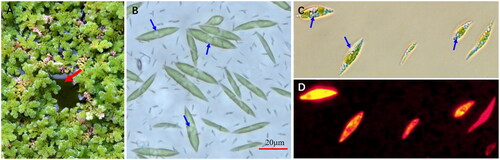
Methods
For fluorescence microscopy, algal cells were stained with Nile red (Chen et al. Citation2009), and observed under a fluorescent microscope (Olympus IX73, Shinjuku City, Japan) with an excitation wavelength of 530 nm.
Genomic DNA was extracted using a Universal Genomic DNA Extraction Kit (Sangon, Shanghai, China) according to the manufacturer’s instruction. The genome was sequenced using Illumina Hiseq 2500 and Nanopore platforms, and was assembled with Canu v1.5 (Koren et al. Citation2017) and wtdbg2 (Ruan and Li Citation2020). The scaffold containing the chloroplast genome was identified by a local blast search using a chloroplast sequence of Chlorella vulgaris (MT920676.1) as a reference (Han et al. Citation2021). The overlapped sequence in the 5′ and 3′ ends of the scaffold was removed with MacVector 13.6. The assembly quality was assessed by mapping the Illumina reads to the assembly, followed by calculating the sequence depth and coverage using a recently published protocol (Ni et al. Citation2023). The protein coding genes were annotated with GeSeq webServer (https://chlorobox.mpimp-golm.mpg.de/geseq.html, as well as by sequence alignment with MacVector 13.6). Transfer RNA genes were annotated with tRNAscan-SE (Chan and Lowe Citation2019). The circular genome was visualized with MacVector 13.6.
For phylogenetic analysis, chloroplast genomes of 20 algal species from Sphaeropleales were retrieved from GenBank. Coding DNA sequences of 20 genes (atpB, atpE, atpF, psbZ, psbE, psbA, rbcL, rps4, rps19, rps12, rpl2, rpl36, rpl5, ycf3, ycf4, psaB, psaC, petD, petG, and petA) that were shared by all taxa were extracted, translated, and combined in the same order. The amino acid sequences were aligned with Clustal Omega (Sievers and Higgins Citation2021). Phylogenetic trees were inferred by using the maximum-likelihood (ML) methods with 1000 bootstrap replicates in MEGAX (Kumar et al. Citation2018). The tree was rooted with a Chlorella vulgaris genome (Han et al. Citation2021). The evolutionary history was inferred using the JTT matrix-based model (Jones et al. Citation1992). The tree with the highest log likelihood (–43849.76) is shown. The percentage of trees in which the associated taxa clustered together is shown next to the branches.
Results
The chloroplast genome of C. braunii ITBB-AG6 has a length of 154,006 bp with a GC content of 31.7%, which is similar to the chloroplast genome of M. neglectum (NW_014013626, 32.4%). The average sequence depth is 5383× with a few nucleotides having a low coverage depth of tens () due to uneven allocation of reads to repetitive fragments (see below).
Figure 2. Organization of the chloroplast genome of C. braunii ITBB-AG6. (A) Coverage depth plot of the genome, the depth was normalized using the logarithm based on 2. (B) Circular map of the genome with genes’ location, size, and orientation indicated by blue (CDS), green (rRNA), and red (tRNA) arrows.
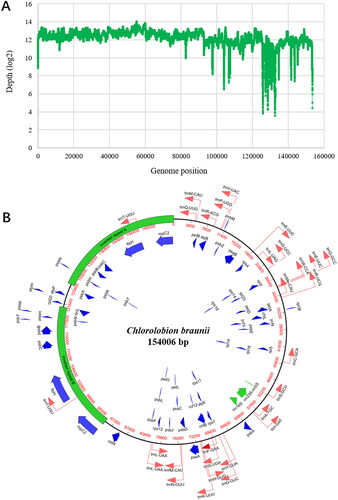
A total of 89 genes were annotated, including 56 protein-coding genes, 30 tRNA genes, and three rRNA genes (). Out of the protein coding genes, 64.3% (36/56) are involved in photosynthesis (), 28.6% (16/56) are involved in protein synthesis, and 7.1% (4/56) are involved in ATP synthesis. The three ribosomal RNA genes (rrn5S, rrn16S, rrn23S) are not interrupted by introns as observed in its mitogenomes (unpublished data). Transfer RNA genes for 20 amino acids were identified, in which the tRNA genes for methionine, leucine, and arginine are tripled, whereas the tRNA genes for glutamic acid, glycine, serine, and threonine are doubled (). Terminal inverted repeats with a length of 27.9 kb and identities of 99.6% were identified (). Ten genes (ropC2, ftsH, psbC, psbB, psbT, psbH, psbK, psbN, rbcL, and trnT) most related to photosynthesis and chloroplast division are located in the repeats, suggesting that photosynthesis may have been strengthened in the evolution of this strain.
Table 1. Gene list of the chloroplast genome of C. braunii ITBB-AG6.
Phylogenomic analysis of related species in Sphaeropleales revealed that C. braunii ITBB-AG6 is positioned in the family Selenastraceae with 100% bootstrap support, and it is most closely related to M. neglectum ().
Figure 3. Consensus maximum-likelihood tree of Sphaeropleales (chlorophyta). The tree is rooted with a Chlorella vulgaris genome (Han et al. Citation2021). Bootstrap supports of 1000 replicates are shown above the branches. The strain in this study is shown in red.
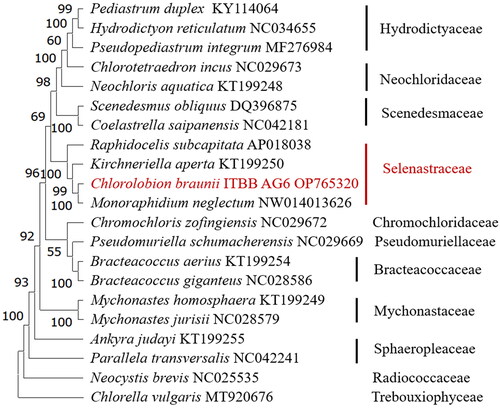
Discussion and conclusions
C. braunii ITBB-AG6 was isolated from a sewage pond covered by azolla for phytoremediation. This pond was once dominated by Trebouxiophyceae algae (e.g. Chlorella vulgaris; Hu et al. Citation2020) before azolla was applied. When the pond was covered by azolla for a few days, ITBB-AG6 became the dominant algal strain, and finally formed a algal film in surface gaps left by azolla (), which prevented sunlight from penetration to submerged algae. The competitive advantage of C. braunii may have come from its large oil body in the cell (), which allowed it to float on water surface and grow in the gaps between azolla plants. The recent duplication of photosystem proteins in its chloroplast genome may have also played a role in enhancing its competitive advantage (), which has not been observed in other green algal species.
Phylogenomic analysis using the chloroplast genomes of Sphaeropleales revealed that strain ITBB-AG6 is most closely related to M. neglectum (), and falls in the family Selenastraceae. Monoraphidium and Chlorolobion are two closely related genera, and are not easy to distinguish by morphology. C. braunii was once named as Monoraphidium braunii (Nägeli ex Kützing) Komárková-Legnerová (Komárková-Legnerová Citation1969), but renamed as Chlorolobion braunii (Nägeli) Komárek (Komárek Citation1979). Our phylogenomic analysis supports this nomenclature.
In summary, the chloroplast genome of C. braunii ITBB-AG6 was sequenced and annotated, and a novel insight into the competitive advantage of this strain over the other microalgae in the azolla community was revealed. This knowledge may be useful in the bioenergy and sewage treatment industry.
Author contributions
Yaojia Mu and Shuai Ma performed genome assembly and annotation; Deguan Tan performed phylogenetic analysis, Xuepiao Sun carried out culture maintenance and microscopic observation; Jiaming Zhang designed the study, analyzed the data, and wrote the draft. All authors revised the manuscript and approved the final version of the manuscript.
Ethical approval
The study involved only a green alga, and was exempted from ethical approval.
Disclosure statement
No potential conflict of interest was reported by the author(s).
Data availability statement
The complete chloroplast genome sequence is openly available in the GenBank of NCBI at https://www.ncbi.nlm.nih.gov under the accession no. OP765320. The Illumina reads generated by the authors to assemble this genome are available under GenBank BioProject no. PRJNA931121, BioSample no. SAMN33050851, and SRA no. SRR23329251.
Additional information
Funding
References
- Baracho DH, Silva JC, Lombardi AT. 2019. The effects of copper on photosynthesis and biomolecules yield in Chlorolobion braunii. J Phycol. 55(6):1335–1347. doi: 10.1111/jpy.12914.
- Chan PP, Lowe TM. 2019. tRNAscan-SE: searching for tRNA genes in genomic sequences. Methods Mol Biol. 1962:1–14. doi: 10.1007/978-1-4939-9173-0_1.
- Chen W, Zhang C, Song L, Sommerfeld M, Hu Q. 2009. A high throughput Nile red method for quantitative measurement of neutral lipids in microalgae. J Microbiol Methods. 77(1):41–47. doi: 10.1016/j.mimet.2009.01.001.
- El-Sheekh MM, Galal HR, Mousa ASH, Farghl AAM. 2023. Coupling wastewater treatment, biomass, lipids, and biodiesel production of some green microalgae. Environ Sci Pollut Res Int. 30(12):35492–35504. doi: 10.1007/s11356-023-25628-y.
- Fucikova K, Lewis PO, Lewis LA. 2014. Putting incertae sedis taxa in their place: a proposal for ten new families and three new genera in Sphaeropleales (Chlorophyceae, Chlorophyta). J Phycol. 50(1):14–25. doi: 10.1111/jpy.12118.
- Fucikova K, Lewis PO, Lewis LA. 2016. Chloroplast phylogenomic data from the green algal order Sphaeropleales (Chlorophyceae, Chlorophyta) reveal complex patterns of sequence evolution. Mol Phylogenet Evol. 98:176–183. doi: 10.1016/j.ympev.2016.01.022.
- Gattullo CE, Bahrs H, Steinberg CE, Loffredo E. 2012. Removal of bisphenol A by the freshwater green alga Monoraphidium braunii and the role of natural organic matter. Sci Total Environ. 416:501–506. doi: 10.1016/j.scitotenv.2011.11.033.
- Gorman DS, Levine RP. 1965. Cytochrome f and plastocyanin: their sequence in the photosynthetic electron transport chain of Chlamydomonas reinhardi. Proc Natl Acad Sci U S A. 54(6):1665–1669. doi: 10.1073/pnas.54.6.1665.
- Guiry MD, Guiry GM. 2018. AlgaeBase.World-wide electronic publication, National University of Ireland, Galway. https://www.algaebase.org; [cited 2023 Mar 2].
- Han B, Mu Y, Tan D, Ma S, Fu L, Sun X, Zhang J. 2021. The chloroplast genome of a unicellular green alga strain isolated from the rubber processing wastewater. Mitochondrial DNA B Resour. 6(1):15–16. doi: 10.1080/23802359.2020.1844090.
- Hu X, Tan D, Fu L, Sun X, Zhang J. 2020. Characterization of the mitochondrion genome of a Chlorella vulgaris strain isolated from rubber processing wastewater. Mitochondrial DNA B Resour. 5(3):2732–2733. doi: 10.1080/23802359.2020.1789004.
- Jones DT, Taylor WR, Thornton JM. 1992. The rapid generation of mutation data matrices from protein sequences. Comput Appl Biosci. 8(3):275–282. doi: 10.1093/bioinformatics/8.3.275.
- Komárek J. 1979. Änderungen in der Taxonomie der Chlorokokkalalgen. Arch Für Hydrobiol Suppl. 56(24):239–263.
- Komárková-Legnerová J. 1969. The systematics and ontogenesis of the genera Ankistrodesmus Corda and Monoraphidium gen. nov. In: Fott B, editor. Studies in phycology. Prague: Academia Publishing House of the Czechoslovak Academy of Sciences; p. 75–144.
- Koren S, Walenz BP, Berlin K, Miller JR, Bergman NH, Phillippy AM. 2017. Canu: scalable and accurate long-read assembly via adaptive k-mer weighting and repeat separation. Genome Res. 27(5):722–736. doi: 10.1101/gr.215087.116.
- Kumar S, Stecher G, Li M, Knyaz C, Tamura K. 2018. MEGA X: molecular evolutionary genetics analysis across computing platforms. Mol Biol Evol. 35(6):1547–1549. doi: 10.1093/molbev/msy096.
- Ni Y, Li J, Zhang C, Liu C. 2023. Generating sequencing depth and coverage map for organelle genomes. protocols.io. doi: 10.17504/protocols.io.4r3l27jkxg1y/v1.
- Ruan J, Li H. 2020. Fast and accurate long-read assembly with wtdbg2. Nat Methods. 17(2):155–158. doi: 10.1038/s41592-019-0669-3.
- Sievers F, Higgins DG. 2021. The clustal omega multiple alignment package. Methods Mol Biol. 2231:3–16. doi: 10.1007/978-1-0716-1036-7_1.
- Wang Q, Shen Q, Wang J, Zhang Y, Zhang Z, Lei Z, Shimizu K, Lee DJ. 2020. Fast cultivation and harvesting of oil-producing microalgae Ankistrodesmus falcatus var. acicularis fed with anaerobic digestion liquor via biogranulation in addition to nutrients removal. Sci Total Environ. 741:140183. doi: 10.1016/j.scitotenv.2020.140183.