Abstract
The complete mitochondrial genome of Rhinogobius maculagenys Wu et al., (Perciformes,Gobiidae) was sequenced and annotated in this study. The circular mitogenome is 16,500 bp long and consists of 13 protein-coding genes, two ribosomal RNA genes, 22 transfer RNA genes and two main non-coding regions (a putative control region and an L-strand replication origin). The overall base composition is 27.5% A, 25.5% T, 16.9% G, and 30.1% C. The gene order and composition are similar to those of other Gobionellinae species. Phylogenetic analysis revealed that R. maculagenys is closely related to Rhinogobius shennongensis in both the maximum likelihood tree and the Bayesian inference tree. The complete mitogenome of R. maculagenys will serve as a valuable resource for future studies on evolution, taxonomy, and genetic conservation of Rhinogobius.
1. Introduction
Of the freshwater gobies, the genus Rhinogobius (Gill, 1859) is a dominant group of benthic fishes in most drainages of East Asia (Chen and Shao Citation1996; Chen et al. Citation1999; Chen and Miller Citation2014). In southern and southeastern mainland China, most species of Rhinogobius are non-diadromous and landlocked (Takahashi and Yanagisawa Citation1999; Huang and Chen Citation2007). Rhinogobius maculagenys Wu et al. (Citation2018) was first discovered in the upper reaches of the Xiangjiang River in Lanshan Country, a county located southeast of mainland China (Wu et al. Citation2018). R. maculagenys can be distinguished from all congeners by a combination of the following features: dorsal fins VI, I/7-9; anal fin I/6-8; pectoral fins 16; longitudinal scales series 32–34; transverse scales series 9–13; predorsal scale series 0; vertebral count 27; pore ω1 missing; head and body yellowish brown; cheek and opercle yellowish brown with over 30 small orange spots (Wu et al. Citation2018). Currently, there is no information on the mitogenome of this species in the public nucleotide database. This study aims to present the complete mitochondrial genome, which will contribute new data for the reconstruction of the Rhinogobius phylogeny.
2. Materials and methods
2.1. Materials
The specimen in this study was collected from Wanshan District, Tongren City, Guizhou province, China (27.7167°N; 108.8350°E). The morphological measurements were conducted following the methods described by Miller (Miller Citation1988) and Suzuki (Suzuki et al. Citation2017). Reference images were captured using a Canon camera (). The collection of fish specimens adhered to the Aquatic Wildlife Protection Regulations of the People’s Republic of China. Specimens were initially fixed in 75% ethanol and later transferred to 95% ethanol for long-term storage. A voucher specimen has been deposited at Mianyang Academy of Agricultural Sciences under the voucher number GY1 (contact Jia Hu: [email protected]).
Figure 1. The specimen of Rhinogobius maculagenys from the Wanshan District, Tongren City, Guizhou province, China(27.7167°N;108.8350°E). the main identifiable morphological features are the first dorsal fin Ⅵ, the second dorsal finI/7; anal-finsI/7; pectoral fin 16; longitudinal scales series 32; transverse scales series 9; predorsal scale series 0; vertebral count 27; pore ω1 missing (Wu Q 2018). (Photo by Jie Mei).
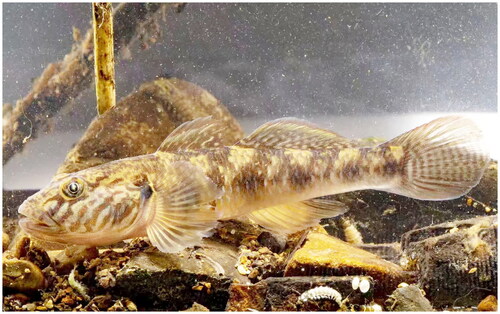
2.2. Methods
Total genomic DNA was extracted from the muscle tissue of R. maculagenys using a Tissue DNA Kit (Qiagen, Germany) following the manufacturer’s protocol. Library pooling and sequencing (PE150 Illumina Hiseq platform 2500) were performed by GeneSky Biotechnologies Inc. Shanghai, China. The depth of coverage is shown in Supplementary Figure S1. The raw data was filtered using fastp v0.36 software (Chen et al. Citation2018) for quality control (QC). The clean reads were then assembled using metaSPAdes v3.13.0 (Nurk et al.Citation2017) with multiple kmers used to find assemblies with the highest N50 values. Contigs of interest were selected by conducting customized command-line blastn analyses, using sequences from Rhinogobius leavelli (GenBank accession: MH729000) as the query (Zhang and Shen Citation2019). The inner gaps within each scaffold were filled using GapFiller v1.11 (Boetzer and Pirovano Citation2012). The assembled sequences were annotated using MitoMaker 1.14 (Bernt et al. Citation2013) with default parameters. Maps of the organellar genomes were generated using CGView (Grant and Stothard Citation2008).
We utilized Blast to filter 12 complete mitochondrial sequences from the same genus in the NCBI database, with a minimum of 88% identity. Those sequences were then used to construct phylogenetic trees, with Pseudogobius taijiangensis (KM624630) serving as the outgroup. The alignment of sequences was performed using ClustalW in MEGA 11 (Tamura et al. Citation2021). Intraspecies genetic distance was calculated using MEGA 11 (Tamura et al. Citation2021). The best fit substitution model (GTR + G) was selected using jModelTest 2.1.10 (Darriba et al.Citation2012) based on the Akaike information criterion (AIC). Maximum-likelihood phylogeny was generated with 1000 replications using MEGA 11(Tamura et al. Citation2021). Bayesian inference phylogenies were inferred using MrBayes3.2.7 (Ronquist et al. Citation2012) with a mixed-model approach (two parallel runs, 1,000,000 generations), in which the initial 25% of the sampled data were discarded as burn-in. Tree information was visualized using FigTree ver.1.4.4 (http://tree.bio.ed.ac.uk/software/figtree/).
3. Results
3.1. Mitogenomic characterization
The complete mitogenome of R. maculagenys is 16,500 bp in length (GenBank accession number OK545540). It consists of 13 protein-coding genes, 22 transfer RNA genes, two ribosomal RNA genes, a putative control region and an L-strand replication origin (OL) (). Structurally, it is very similar to other Gobionellinae mitogenomes. Most of these genes are encoded by the H-strand, except for the ND6 gene and eight tRNA genes. The overall base composition is 27.5% A, 25.5% T, 30.1% Cand 16.9%G. All protein-coding genes use the initiation codon ATG except for the COX1 gene, which begins with GTG. Additionally, most protein-coding genes use TAA or TAG as stop codons, while COX3 uses an incomplete stop codon TA. ND4, COX2 and CYTB use an incomplete stop codon T. The 13 protein-coding genes encode 3787 amino acids, with leucine being the most frequently used amino acid (17.6%), while cysteine is the least used (0.7%). The two rRNA genes (12S and 16S) are separated by tRNA-Val, located between tRNA-Phe and tRNA-Leu. The 22 tRNA genes are interspersed between rRNAs and protein-coding genes, with sizes ranging from 66 bp (tRNA-Cys) to 76 bp (tRNA-Lys). The 32 bp OL is located between the tRNA-Asn and tRNA-Cys genes. The putative control region is 481 bases long and located between tRNA-Pro and tRNA-Phe.
Figure 2. Mitochondrial map of Rhinogobius maculagenys. Circular maps were drawn with CGView (Grant and Stothard Citation2008). The arrows indicate the orientation of gene transcription. Protein-coding genes are shown as blue arrows, rRNA genes as green arrows, tRNA genes as purple arrows and putative control region(D-loop) as dark blue. The GC content was plotted using a black sliding window, as the deviation from the average GC content of the entire sequence. GC-skew was plotted as the deviation from the average GC-skew of the entire sequence, with an average value of −0.28. The window width was set to 500 bp and the step size was set to 1 bp. The inner cycle indicates the location of the genes in the mt genome.
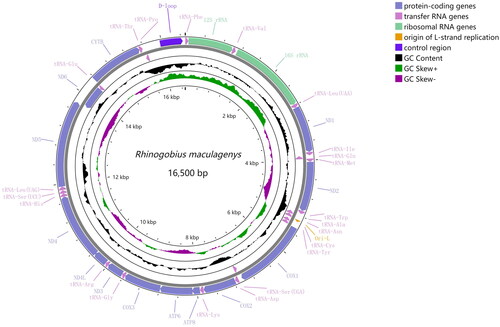
3.2. Phylogenetic analysis
The phylogenies reconstructed by Bayesian and ML methods were topologically identical (). Our analyses revealed that both trees showed that R. maculagenys shared the closest mitochondrial genome relationship with R. shennongensis, and together they formed a clade with Rhinogobius niger. The genetic distance between R. maculagenys and the other 11 Rhinogobius species ranged from 0.0567 to 0.1319.
Figure 3. A Phylogenetic tree of thirteen species was constructed based on complete mitogenome using maximum-likelihood method and Bayesian inference. Pseudogobius taijiangensis was used as outgroups. Numbers near nodes indicate maximum-likelihood bootstrap percentages (BP) and Bayesian posterior probabilities (BPP), given as BP/BPP. The estimates of branch lengths from ML methods. The following sequences were used: Rhinogobius maculagenys OK545540, Rhinogobius yaima LC648308 (Maeda et al. Citation2021), Rhinogobius yonezawai LC648309 (Maeda et al. Citation2021), Rhinogobius brunneus LC648311 (Maeda et al. Citation2021), Rhinogobius flumineus LC648306 (Maeda et al. Citation2021), Rhinogobius formosanus MN549279, Rhinogobius davidi OM617724 (Song et al.Citation2023), Rhinogobius leavelli MH729000 (Zhang and Shen Citation2019), Rhinogobius cliffordpopei KT357638 (Wang et al. Citation2019), Rhinogobius niger OM791349, Rhinogobius shennongensis OM961050, Rhinogobius similis KU871066, Pseudogobius taijiangensis KM624630.
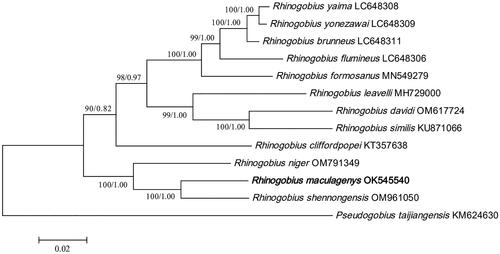
4. Discussion and conclusion
The circular mitogenome of R. maculagenys is 16,500 bp in length. The gene order and composition are similar to those of other Gobionellinae species (Wang et al. Citation2019; Tan et al. Citation2020; Yang et al. Citation2020; Song et al. Citation2022). When compared with sequences deposited in the GenBank, the control region in the Rhinogobius genus was found to be 843 bp in R.duospilus (MH127918) and 529 bp in R.szechuanensis (OM617727). The composition and arrangement of the control region varied considerably within this genus. Phylogenetic analyses of R. maculagenys reveal a close relationship with R.shennongensis, which is consistent with the findings of Song (Song et al.Citation2023). However, it is important to note that changes in taxonomic sampling can potentially impact the relationships of species in the phylogenetic tree. Therefore, further studies involving extensive taxon sampling are necessary to accurately verify the phylogenetic relationships among the Rhinogobius genus. Our findings significantly contribute to understanding the genetic diversity and evolution of Rhinogobius and provide valuable insights for future studies in this field.
Authors’ contribution
JH and YL made substantial contributions to the design of this work, drafting of the paper and final approval of the version to be published. JL, HW and ZX analyzed the data; CC, XC and ZW conducted DNA experiment; All authors agreed to be accountable for all aspects of this work.
Ethical approval
Sample collection protocols were approved by the Ethics Committee for Animal Experiments of Mianyang Academy of Agricultural Sciences (approval number: MAAS 2021001). These policies were enacted according to the Chinese Association for the Laboratory Animal Sciences and the Institutional Animal Care and Use Committee (IACUC) protocols.
Supplemental Material
Download PDF (168.8 KB)Acknowledgements
We are grateful to Professor Lu Wang of Tongren vocational college for his guidance in sample collection.
Disclosure statement
No potential conflict of interest was reported by the author(s).
Data availability statement
The data that support the findings of this study are openly available in Genebank at http://www.ncbi.nlm.nih.gov/, under the accession number OK545540. The associated BioProject, SRA, and Bio-Sample numbers are PRJNA944416, SRR23852506 and SAMN33748151, respectively.
Additional information
Funding
References
- Bernt M, Donath A, Jühling F, Externbrink F, Florentz C, Fritzsch G, Pütz J, Middendorf M, Stadler PF. 2013. MITOS: improved de novo metazoan mitochondrial genome annotation. Mol Phylogenet Evol. 69(2):313–319. doi: 10.1016/j.ympev.2012.08.023.
- Boetzer M, Pirovano W. 2012. Toward almost closed genomes with GapFiller. Genome Biol. 13(6):R56. doi: 10.1186/gb-2012-13-6-r56.
- Chen IS, Shao KT. 1996. A taxonomic review of the gobiid fish genus Rhinogobius Gill, 1859, from Taiwan, with descriptions of three new species. Zool Stud. 35(3):200–214.
- Chen IS, Kottelat M, Miller PJ. 1999. Freshwater gobies the genus Rhinogobius from the Mekong basin in Thailand and Laos, with descriptions of three new species. Zool Stud. 38:19–32.
- Chen IS, Miller PJ. 2014. A new freshwater goby of Rhinogobius(Teleostei: Gobiidae) from Hainan Island, southern China. J Mar Sci Technol. 21(Supplement):124–129.
- Chen S, Zhou Y, Chen Y, Gu J. 2018. fastp: An ultra-fast all-in-one FASTQ preprocessor. Bioinformatics. 34(17):i884–i890. doi: 10.1093/bioinformatics/bty560.
- Darriba D, Taboada GL, Doallo R, Posada D. 2012. jModelTest 2: more models, new heuristics and parallel computing. Nat Methods. 9(8):772–772. doi: 10.1038/nmeth.2109.
- Grant JR, Stothard P. 2008. The CGView Server: a comparative genomics tool for circular genomes. Nucleic Acids Res. 36(Web Server issue):W181–W184. doi: 10.1093/nar/gkn179.
- Huang S, Chen I. 2007. Three new species of Rhinogobius Gill, 1859 (Teleostei: Gobiidae) from the Hanjiang basin, Southern China. Raffles Bulletin of Zoology. 14:101–110.
- Maeda K, Shinzato C, Koyanagi R, Kunishima T, Kobayashi H, Satoh N, Palla HP. 2021. Two new species of Rhinogobius (Gobiiformes: Oxudercidae) from Palawan, Philippines, with their phylogenetic placement. Zootaxa. 5068(1):81–98. doi: 10.11646/zootaxa.5068.1.3.
- Miller PJ. 1988. New species of Corcyrogobius, Thorogobius and Wheelerigobius from West Africa (Teleostei: Gobiidae). J Nat Hist. 22(5):1245–1262. doi: 10.1080/00222938800770761.
- Nurk S, Meleshko D, Korobeynikov A, Pevzner PA. 2017. metaSPAdes: A new versatile metagenomic assembler. Genome Res. 27(5):824–834. doi: 10.1101/gr.213959.116.
- Ronquist F, Teslenko M, van der Mark P, Ayres DL, Darling A, Hohna S, Larget B, Liu L, Suchard MA, Huelsenbeck JP. 2012. MrBayes 3.2: Efficient Bayesian phylogenetic inference and model choice across a large model space. Syst Biol. 61(3):539–542. doi: 10.1093/sysbio/sys029.
- Song L, Chen XJ, Mao HX, Wang Q. 2022. Characterization and phylogenetic analysis of the complete mitochondrial genome of Rhinogobius wuyanlingensis (Gobiiformes: Gobiidae: Gobionellinae). Mitochondrial DNA B Resour. 7(7):1323–1325. doi: 10.1080/23802359.2022.2097488.
- Song L, Chen XJ, Gu YW, Wang Q. 2023. Complete mitochondrial genome sequence and annotation of Rhinogobius lentiginis(Gobiiformes: Gobiidae: Gobionellinae). Mitochondrial DNA B Resour. 8(3):418–421. doi: 10.1080/23802359.2023.2189497.
- Song L, Chen XJ, Song Y, Wang Q. 2023. First complete mitochondrial genome of the endemic goby, Rhinogobius davidi(Gobiiformes: Gobiidae: Gobionellinae), in China. Mitochondrial DNA B Resour. 8(3):410–413. doi: 10.1080/23802359.2023.2189493.
- Suzuki T, Shibukawa K, Aizawa M. 2017. Rhinogobius mizunoi, a new species of freshwater goby (Teleostei: Gobiidae) from Japan. Bull Kanagawa Prefect Mus (Nat Sci). 46:79–95.
- Takahashi D, Yanagisawa Y. 1999. Breeding ecology of an amphidromous goby of the genus Rhinogobius. Ichthyol Res. 46(2):185–191. doi: 10.1007/BF02675437.
- Tamura K, Stecher G, Kumar S. 2021. MEGA11: molecular evolutionary genetics analysis version 11. Mol Biol Evol. 38(7):3022–3027. doi: 10.1093/molbev/msab120.
- Tan HY, Yang YY, Zhang M, Chen XL. 2020. The complete mitochondrial genome of Rhinogobius duospilus(Gobiidae: Gobionellinae). Mitochondrial DNA B Resour. 5(3):3406–3407. doi: 10.1080/23802359.2020.1823279.
- Wang D, Dai CX, Li Q, Li YH, Liu ZZ. 2019. Complete mitochondrial genome and phylogenic analysis of Rhinogobius cliffordpopei (Perciformes, Gobiidae). Mitochondrial DNA B Resour. 4(2):2473–2474. doi: 10.1080/23802359.2019.1637287.
- Wu QQ, Deng XJ, Wang YJ, Liu Y. 2018. Rhinogobius maculagenys, a new species of freshwater goby (Teleostei: Gobiidae) from Hunan, China. Zootaxa. 4476(1):118–129. doi: 10.11646/zootaxa.4476.1.11.
- Yang CJ, Chen Y, Chen Z, He GH, Zhong ZL, Xue WB. 2020. The next-generation sequencing reveals the complete mitochondrial genome of Rhinogobius formosanus (Perciformes: Gobiidae). Mitochondrial DNA B Resour. 5(3):2673–2674. doi: 10.1080/23802359.2020.1787261.
- Zhang FB, Shen YJ. 2019. Characterization of the complete mitochondrial genome of Rhinogobius leavelli(Perciformes: Gobiidae: Gobionellinae) and its phylogenetic analysis for Gobionellinae. Biologia. 74(5):493–499. doi: 10.2478/s11756-018-00189-5.