Abstract
Callicarpa dichotoma (Lour.) K.Koch is a shrub species with distribution from East Asia to Southeast Asia. We assembled and annotated for the first time the complete chloroplast (cp) genome of C. dichotoma. The cp genome of C. dichotoma is 154,110 bp long with the GC content of 38.09% and consists of four subregions: a large single-copy (LSC) region of 84,915 bp, a small single-copy (SSC) region of 17,783 bp and a pair of inverted repeats (IRs) of 25,706 bp each. The cp genome of C. dichotoma encodes a total of 114 unique genes, comprising 80 protein-coding genes, 30 tRNA genes, and four rRNA genes. Phylogenetic trees based on the coding sequences strongly support the position of C. dichotoma within the genus Callicarpa, confirming the previously reported monophyly of the genus.
Introduction
The genus Callicarpa L. (Lamiaceae; inc. sed. Verbenaceae) is commonly known as ‘beautyberry’ due to their outstanding fruits, with ca. 140 species recognized worldwide (Mabberley Citation2017). The genus is also known for its chemical composition, such as callicarpone (Kawazu et al. Citation1967). The plants and their organic, plant-derived components have traditionally been used for centuries as a piscicide, pesticide, and insecticide, as well as a herbal medicine for the prevention and treatment of a wide number of health disorders in traditional pharmacology of China and other parts of Asia (Kawazu Citation1965; Jones and Kinghorn Citation2008; Tu et al. Citation2013; Hung et al. Citation2023).
Callicarpa dichotoma (Lour.) K.Koch 1872, or purple beautyberry, is a small, common shrub that has its natural distribution range in the temperate to subtropical zone of East Asia to Southeast Asia, from Japan to Vietnam (Yamazaki Citation1993; Pham Citation2003). C. dichotoma is used as an ornamental plant in America because of its colorful fruit (Bachmann Citation2002; Bramley Citation2009). However, C. dichotoma has recently been confirmed to be potentially invasive as it is spreading in southeastern USA (Weakley Citation2015; Atha et al. Citation2019). Although C. dichotoma is a common species and can crossbreed without barriers with other Callicarpa species, such as C. bodinieri H.Lév. (Xu et al. Citation2013), its chloroplast (cp) genome has not been elucidated. In this study, we assembled and annotated the complete cp genome of C. dichotoma to better understand the position of C. dichotoma within the genus Callicarpa and for future research of the biogeography and evolution of the genus.
Materials and methods
Sample collection and preservation
Callicarpa dichotoma (Lour.) K.Koch samples were collected from Hiroshima Prefecture, Japan (34.31N, 132.29E) (). The voucher specimen was deposited at the Herbarium of Hiroshima University (hiro: http://sweetgum.nybg.org/science/ih/herbarium-details/?irn=124749, Prof. Tomio Yamaguchi, [email protected]) under specimen number HIRO-MY 156564.
Figure 1. Callicarpa dichotoma (Lour.) K.Koch. (A) Flowering branches. (B) Close-up of flowering and fruiting branch, showing young fruits, flowers, and flower buds from left to right. Morphological features: C. dichotoma is a short deciduous shrub in the beautyberry genus Callicarpa (Lamiaceae). The branches have purplish color with stellate hairs; the leaves are simple, opposite, pubescent, ovate-oblong, acuminate at apex and cuneate at base, and serrate near the leaf tip; the cyme inflorescences are supra-axillary; the corolla is pale purple, glabrous; the stamens are exserted; and the fruits are purple.
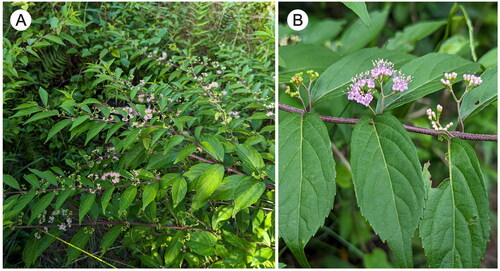
DNA extraction and sequencing, and cleaning raw reads
Total DNA was extracted from a frozen sample with NucleoSpin Plant II (Macherey-Nagel, Duren, Germany) following the manufacturer’s instruction. Library preparation and sequencing were performed by Bioengineering Lab. Co., Ltd. (Sagamihara, Japan) using the MGI DNBSEQ-G400RS FAST platform (MGI Tech Co. Ltd., Shenzhen, China). Approximately, 77.96 M raw reads were analyzed, comprising an average fragment length of 200 bp.
Assembly, annotation, and visualization
Low-quality reads (<Q30), abnormal short reads (<20 bp), and adapter sequences were trimmed using fastp ver. 0.23.2 (Chen et al. Citation2018). After quality control, GetOrganelle ver. 1.7.6.1 (Jin et al. Citation2020) was used for de novo assembly with its standard parameters and the reference data were included, and the assembled sequences were polished using Polypolish ver. 0.5.0 (Wick and Holt Citation2022). The polished sequences were annotated using GeSeq ver. 2.03 (Tillich et al. Citation2017) for protein coding and rRNA genes, and tRNAscan-SE 2.0 (Chan and Lowe Citation2019) for tRNA genes, and manually corrected using SnapGene Viewer ver. 7.0.2 (GSL Biotech, https://www.snapgene.com). To verify the accuracy of the assembly, we mapped clean reads to the assembled cp genome to assess the depth of coverage (Figure S1). A circular map was generated from the final annotated cp sequence OGDRAW ver. 1.3.1 (Greiner et al. Citation2019). The structures of intron-containing genes were also visualized using the CPGView online web (http://www.1kmpg.cn/cpgview, Liu et al. Citation2023; Figures S2 and S3). The cp genome sequence was submitted to the DNA Data Bank of Japan (DDBJ, https://ddbj.nig.ac.jp/arsa/) under the accession number LC775283.
Figure 2. Circular map of Callicarpa dichotoma (Lour.) K.Koch chloroplast genome. In the chloroplast genome, the small single-copy (SSC) and large single-copy (LSC) regions are separated by inverted repeats (IRs: IRA and IRB). Genes inside the map are transcribed clockwise, and genes outside are transcribed counterclockwise. Genes with related functions are shown in the same color. Asterisks denote genes containing introns.
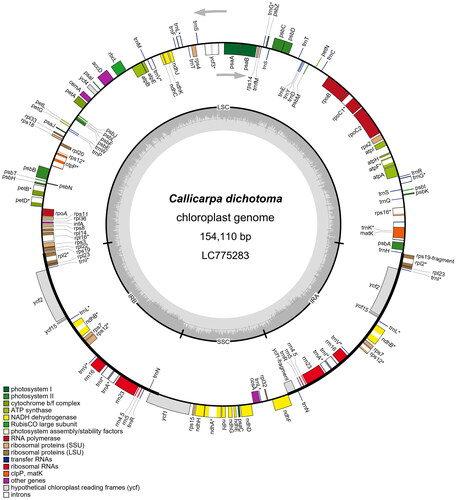
Figure 3. Maximum-likelihood cladogram based on 73 chloroplast protein-coding sequences for Callicarpa species and closely related taxa. Numbers at the branches represent the bootstrap support values of 10,000 replicates, bootstrap values under 50 were not shown. The root is arbitrarily placed on the branch leading to Mentha haplocalyx. The analysis involved 21 nucleotide sequences. There were a total of 59,702 positions in the final dataset. Evolutionary analyses were conducted in RAxML-NG. The following sequences were used: Callicarpa bodinieri MW149077 (Wang et al. Citation2019), Callicarpa nudiflora MK783316 (Wang et al. Citation2019), Callicarpa cathayana MZ424314, Callicarpa rubella MZ424313, Callicarpa rubella MZ520129 (Cai et al. Citation2021), Callicarpa integerrima var. chinensis MW788028 (Gu et al. Citation2021), Callicarpa siongsaiensis MW343456 (Xie et al. Citation2021), Callicarpa brevipes MT473739 (Zhao et al. Citation2021), Callicarpa peichieniana MT473741 (Zhao et al. Citation2021), Callicarpa macrophylla MW829279 (Liu et al. Citation2022), Callicarpa macrophylla MT473740 (Zhao et al. Citation2021), Callicarpa longifolia var. floccosa MW149076 (Wang et al. Citation2021), Callicarpa formosana MT830861 (Du et al. Citation2020), Callicarpa kochiana MZ424315, Callicarpa formosana MW252167, Callicarpa americana MN883825 (Zhao et al. Citation2020), Callicarpa arborea MT473738 (Zhao et al. Citation2021), Dicrastylis parvifolia MT473755 (Zhao et al. Citation2021), Vitex rotundifolia MT937186 (Jo et al. Citation2021), and Mentha haplocalyx MN102358 (He et al. Citation2020).
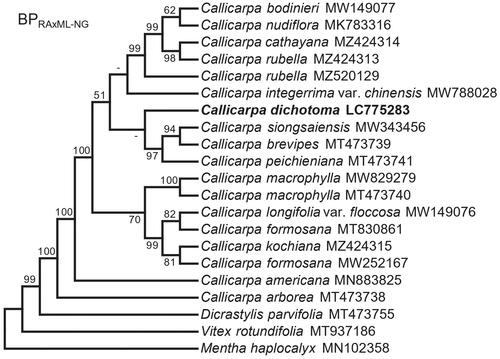
Phylogenetic reconstruction
Phylogenetic analyses were conducted using protein-coding sequences (73 genes) of the cp genome. The data matrix consisted of all Callicarpa species with available cp genomes and closely related species in Lamiaceae (Table S1), based on the topologies demonstrated by previous studies (Li et al. Citation2016; Zhao et al. Citation2021; Liu et al. Citation2023). Sequences were aligned by MAFFT ver. 7.511 in the online web system (Katoh et al. Citation2019). After manual checking and arrangement for the alignment, start and stop codons were removed using the sequence editor of MEGA ver. 7.0.26 (Kumar et al. Citation2016). Gaps were treated as missing data. ModelTest-NG ver. 0.2.0 (Darriba et al. Citation2020) was used to determine the substitution model for each gene based on the corrected Akaike information criterion (AICc; Sugiura Citation1978). The selected substitution models are listed in Table S2. RAxML-NG ver. 1.1.0 (Kozlov et al. Citation2019) was used for maximum-likelihood inference with a rapid bootstrap analysis of 10,000 replicates.
Results and discussion
Characterization of the chloroplast genome
The cp genome of C. dichotoma is a 154,110-bp circular DNA molecule with a typical quadripartite structure composed of a large single-copy (LSC) region of 84,915 bp, a small single-copy (SSC) region of 17,783 bp, and a pair of inverted repeats (IRs) of 25,706 bp each (). The genome’s GC content is 38.09%. It has 114 unique genes, including 80 protein-coding genes, 30 tRNA genes, and four rRNA genes. In the IR regions, 17 genes are repeated, including six protein-coding genes, seven tRNAs, and four rRNAs. The gene rps12 is a trans-splicing gene with the first exon located in the LSC and two remaining exons in each of the IR regions. C. dichotoma possessed two fragments of ycf1 and rps19 genes (pseudogenes) located in the IRA, similar with C. formosana MT830861. C. dichotoma had a similar genome structure and gene number to C. arborea MT473738, C. brevipes MT473739, and C. rubella MZ520129, but differed in one protein-coding gene or one tRNA with species such as C. integerrima var. chinensis MW788028 in lacking rpl32 gene. C. macrophylla MW829279 includes an additional ycf68 gene, and C. siongsaiensis MW343456 lacks trnV-UAC (tRNA-Val). The sequence length of the cp genome obtained in this study is the third shortest among Callicarpa species previously reported, after C. peichieniana (154,102 bp, MT473741) and C. nudiflora (154,080 bp, MK783316).
Phylogenetic analysis of C. dichotoma within the genus Callicarpa
The cp genome data matrix for the phylogenetic analysis was 59,702 bp long, with 3340 variable sites (5.6%) and 589 parsimony-informative sites (17.6% of the variable sites). The phylogenetic tree fully resolved the position of C. dichotoma within the Asian Callicarpa group, thus supporting the monophyly of the genus Callicarpa (). The sister relationship of C. dichotoma with the clade of C. peichieniana Chun & S.L.Chen ex H.Ma & W.B.Yu, C. siongsaiensis F.P.Metcalf, and C. brevipes (Benth.) Hance was not well resolved since the branches were very short (see the phylogram in Figure S4). The sister relationship of Callicarpa (subfamily Callicarpoideae) and Dicrastylis (subfamily Prostantheroideae) was well supported, comparable with the results of Zhao et al. (Citation2021).
Conclusions
The cp genome of Callicarpa dichotoma, reported for the first time, had a length of 154,110 bp, and a similar cp genome structure, quadripartite structure, with that of other Callicarpa species. The cp genome was divided into four regions: LSC, SSC, and two IRs with the length of 84,915 bp, 17,783 bp, and 25,706 bp each, respectively. It had 114 unique, different genes, comprising 80 protein-coding genes, 30 tRNA genes, and four rRNA genes. The phylogenetic analysis supported the position of C. dichotoma within the Asian Callicarpa group. The complete cp sequence of C. dichotoma will contribute to future studies on population genetics, biogeography, evolution, and conservation of the family.
Author contributions
Conceptualization: Q. C. Phan, Y. Inoue, and H. Tsubota; data curation: Q. C. Phan, R. Nagasaki, Y. Inoue, and H. Tsubota; formal analysis: H. Tsubota; funding acquisition: H. Tsubota; methodology: Q. C. Phan, R. Nagasaki, Y. Inoue, and H. Tsubota; investigation: H. Tsubota; writing – original draft preparation: Q. C. Phan; writing – review and editing: R. Nagasaki, Y. Inoue, and H. Tsubota; resources: Q. C. Phan, Y. Inoue, and H. Tsubota; all authors read and approved the final manuscript.
Ethical approval
Research on plant organelle genome sequencing does not affect the population and does not require ethical approval. The analyzed species is widely distributed in Japan, and the material was not obtained from nature reserves.
Supplemental Material
Download PDF (197.6 KB)Supplemental Material
Download PDF (204.7 KB)Supplemental Material
Download PDF (182.2 KB)Supplemental Material
Download PDF (1.8 MB)Supplemental Material
Download PDF (34.4 KB)Supplemental Material
Download PDF (74.8 KB)Acknowledgements
DNBSEQ sequencing was performed by Bioengineering Lab. Co., Ltd. (Sagamihara, Japan). Dr. Miho Nakahara-Tsubota and Ms. A. Tsubota helped us with the sample collection. We would like to thank Professor R. D. Seppelt (Bundall, Queensland, Australia) for checking the English text and suggestions.
Disclosure statement
The authors report no potential conflict of interest.
Data availability statement
The genome sequence data that supports the findings of this study are openly available at NCBI (https://www.ncbi.nlm.nih.gov/) under the accession no. LC775283. The associated BioProject, BioSample, and SRA/DRA numbers are PRJDB15898, SAMD00611820, and DRX478534, respectively.
Additional information
Funding
References
- Atha D, Gunderson M, Whitaker E, Alvarez R, Young M. 2019. First report of Callicarpa dichotoma and Callicarpa japonica (Lamiaceae) spontaneous and potentially invasive in New York and New Jersey. Phytoneuron. 17:1–3.
- Bachmann J. 2002. Woody ornamentals for cut flower growers. Appropriate technology transfer for rural areas. National Center for Appropriate Technology; [accessed 2023 Aug 16]. https://citeseerx.ist.psu.edu/document?repid=rep1&type=pdf&doi=3b3c8d32e3104027160ee734598bda05766faab3.
- Bramley GL. 2009. The genus Callicarpa (Lamiaceae) on Borneo. Bot J Linn Soc. 159(3):416–455. doi: 10.1111/j.1095-8339.2009.00907.x.
- Cai H, Liu X, Su Z, Ma Z. 2021. The complete chloroplast genome of Callicarpa rubella Lindl. (Lamiaceae) from Guangxi, China: genome structure and phylogenetic analysis. Mitochondrial DNA B Resour. 6(11):3280–3282. doi: 10.1080/23802359.2021.1993106.
- Chan PP, Lowe TM. 2019. tRNAscan-SE: searching for tRNA genes in genomic sequences. Methods Mol Biol. 1962:1–14.
- Chen S, Zhou Y, Chen Y, Gu J. 2018. fastp: an ultra-fast all-in-one FASTQ preprocessor. Bioinformatics. 34(17):i884–i890. doi: 10.1093/bioinformatics/bty560.
- Darriba D, Posada D, Kozlov AM, Stamatakis A, Morel B, Flouri T. 2020. ModelTest-NG: a new and scalable tool for the selection of DNA and protein evolutionary models. Mol Biol Evol. 37(1):291–294. doi: 10.1093/molbev/msz189.
- Du Y, Liu Y, Liu B, Wang T. 2020. Complete chloroplast genome of Callicarpa formosana Rolfe, a famous ornamental plant and traditional medicinal herb. Mitochondrial DNA B Resour. 5(3):3383–3384. doi: 10.1080/23802359.2020.1820399.
- Greiner S, Lehwark P, Bock R. 2019. OrganellarGenomeDRAW (OGDRAW) version 1.3.1: expanded toolkit for the graphical visualization of organellar genomes. Nucleic Acids Res. 47(W1):W59–W64. doi: 10.1093/nar/gkz238.
- Gu C, Zhang Q, Zhang D, Zhang Y, Liang J. 2021. The complete chloroplast genome of Callicarpa integerrima var. chinensis (Lamiaceae) from Guilin, China, a potential medicinal plant. Mitochondrial DNA B Resour. 6(9):2779–2780. doi: 10.1080/23802359.2021.1969696.
- He SL, Yang Y, Tian Y. 2020. Characteristic and phylogenetic analyses of chloroplast genome for Mentha haplocalyx (Lamiaceae). Mitochondrial DNA B Resour. 5(3):2099–2100. doi: 10.1080/23802359.2019.1680322.
- Hung NH, Quan PM, Dai DN, Satyal P, Huong LT, Giang LD, Hung LT, William N. 2023. Environmentally-friendly pesticidal activities of Callicarpa and Karomia essential oils from Vietnam and their microemulsions. Chem Biodivers. 20(3):e202200210. doi: 10.1002/cbdv.202200210.
- Jin J-J, Yu W-B, Yang J-B, Song Y, dePamphilis CW, Yi T-S, Li D-Z. 2020. GetOrganelle: a fast and versatile toolkit for accurate de novo assembly of organelle genomes. Genome Biol. 21(1):241. doi: 10.1186/s13059-020-02154-5.
- Jo S, Kim HW, Kim YK, Cheon SH, Joo MJ, Hong JR, Kwak M, Kim KJ. 2021. Three complete plastome sequences from the families of Lamiaceae, Mazaceae, and Phrymaceae (Lamiales). Mitochondrial DNA B Resour. 6(1):224–226. doi: 10.1080/23802359.2020.1861563.
- Jones WP, Kinghorn AD. 2008. Biologically active natural products of the genus Callicarpa. Curr Bioact Compd. 4(1):15–32. doi: 10.2174/157340708784533393.
- Katoh K, Rozewicki J, Yamada KD. 2019. MAFFT online service: multiple sequence alignment, interactive sequence choice and visualization. Brief Bioinform. 20(4):1160–1166. doi: 10.1093/bib/bbx108.
- Kawazu K, Inaba M, Mitsui T. 1967. Studies on fish-killing components of Callicarpa candicans. Part I. Isolation of callicarpone and its toxicity to fish. Part II. Structure of callicarpone. Agric Biol Chem. 31(4):494–506. doi: 10.1080/00021369.1967.10858825.
- Kawazu K. 1965. A survey of some piscicide plants in Malaysia. Jpn J Southeast Asian Stud. 3(3):161–165. doi: 10.20495/tak.3.3_161
- Kozlov AM, Darriba D, Flouri T, Morel B, Stamatakis A. 2019. RAxML-NG: a fast, scalable and user-friendly tool for maximum likelihood phylogenetic inference. Bioinformatics. 35(21):4453–4455. doi: 10.1093/bioinformatics/btz305.
- Kumar S, Stecher G, Tamura K. 2016. MEGA7: molecular evolutionary genetics analysis version 7.0 for bigger datasets. Mol Biol Evol. 33(7):1870–1874. doi: 10.1093/molbev/msw054.
- Li B, Cantino PD, Olmstead RG, Bramley GLC, Xiang C-L, Ma Z-H, Tan Y-H, Zhang D-X. 2016. A large-scale chloroplast phylogeny of the Lamiaceae sheds new light on its subfamilial classification. Sci Rep. 6(1):34343. doi: 10.1038/srep34343.
- Liu S, Ni Y, Li J, Zhang X, Yang H, Chen H, Liu C. 2023. CPGView: a package for visualizing detailed chloroplast genome structures. Mol Ecol Resour. 23(3):694–704. doi: 10.1111/1755-0998.13729.
- Liu X, Cai H-M, Wang W-Q, Lin W, Su Z-W, Ma Z-H. 2023. Why is the beautyberry so colourful? Evolution, biogeography, and diversification of fruit colours in Callicarpa (Lamiaceae). Plant Divers. 45(1):6–19. doi: 10.1016/j.pld.2022.10.002.
- Liu Y, Guo S, Bei J, Wu W. 2022. The complete chloroplast genome of Callicarpa macrophylla Vahl. Mitochondrial DNA B Resour. 7(10):1845–1847. doi: 10.1080/23802359.2022.2134749.
- Mabberley DJ. 2017. Mabberley’s plant-book: a portable dictionary of plants, their classification and uses. 4th ed. Cambridge: Cambridge University Press; xix + 1102 pp.
- Pham HH. editor. 2003. Verbenaceae. In: An illustrated flora of Vietnam. Vol. 2. Ho Chi Minh City: Tre Publishing House; p. 811–845.
- Sugiura N. 1978. Further analysis of the data by Akaike’s information criterion and the finite corrections. Commun Stat Theory Methods. 7(1):13–26. doi: 10.1080/03610927808827599.
- Tillich M, Lehwark P, Pellizzer T, Ulbricht-Jones ES, Fischer A, Bock R, Greiner S. 2017. GeSeq: versatile and accurate annotation of organelle genomes. Nucleic Acids Res. 45(W1):W6–W11. doi: 10.1093/nar/gkx391.
- Tu Y, Sun L, Guo M, Chen W. 2013. The medicinal uses of Callicarpa L. in traditional Chinese medicine: an ethnopharmacological, phytochemical and pharmacological review. J Ethnopharmacol. 146(2):465–481. doi: 10.1016/j.jep.2012.12.051.
- Wang CY, Chen LH, He HL, Liu Y, Wang Q. 2021. The complete chloroplast genome of Callicarpa longifolia Lamk. var. floccosa Schauer (Lamiaceae). Mitochondrial DNA B Resour. 6(12):3473–3474. doi: 10.1080/23802359.2021.2002210.
- Wang CY, Luo JH, He HL, Wang Q. 2021. The complete chloroplast genome of Callicarpa bodinieri (Lamiales, Lamiaceae), an ornamental and medicinal plant from Chongqing, China. Mitochondrial DNA B Resour. 6(3):1229–1230. doi: 10.1080/23802359.2021.1875932.
- Wang HX, Chen L, Cheng XL, Chen WS, Li LM. 2019. Complete plastome sequence of Callicarpa nudiflora Vahl (Verbenaceae): a medicinal plant. Mitochondrial DNA B Resour. 4(2):2090–2091. doi: 10.1080/23802359.2019.1613197.
- Weakley AS. 2015. Flora of the Southern and Mid-Atlantic States. University of North Carolina Herbarium; [accessed 2023 Aug 16]. https://citeseerx.ist.psu.edu/document?repid=rep1&type=pdf&doi=86006155df72cc7bc778a3ed51c5397372feea72.
- Wick RR, Holt KE. 2022. Polypolish: short-read polishing of long-read bacterial genome assemblies. PLoS Comput Biol. 18(1):e1009802. doi: 10.1371/journal.pcbi.1009802.
- Xie Y, Luo S, Zhang L, Huang H, Zhang Q, Lai M, Deng C. 2021. The complete chloroplast genome of Callicarpa siongsaiensis Metcalf (Lamiaceae) from Fujian Province, China: genome structure and phylogenetic analysis. Mitochondrial DNA B Resour. 6(5):1634–1635. doi: 10.1080/23802359.2021.1926364.
- Xu L, Chen F, Xie Y. 2013. Cross-breeding between two species of Callicarpa. J Huazhong Agric Univ. 32:23–27.
- Yamazaki T. 1993. Verbenaceae. In: Iwatsuki K, Yamazaki T, Boufford DE, Ohba H (editors). Flora of Japan. Vol. IIIa: Angiospermae-Dicotyledoneae: Sympetalae(a). Tokyo: Kodansha Ltd.; p. 259–271.
- Zhao F, Chen Y-P, Salmaki Y, Drew BT, Wilson TC, Scheen A-C, Celep F, Bräuchler C, Bendiksby M, Wang Q, et al. 2021. An updated tribal classification of Lamiaceae based on plastome phylogenomics. MBC Biol. 19:1–27.
- Zhao F, Li B, Drew BT, Chen YP, Wang Q, Yu WB, Liu ED, Salmaki Y, Peng H, Xiang CL. 2020. Leveraging plastomes for comparative analysis and phylogenomic inference within Scutellarioideae (Lamiaceae). PLOS One. 15(5):e0232602. doi: 10.1371/journal.pone.0232602.