Abstract
Luisia hancockii Rolfe 1896 is an epiphytic orchid species. In our present study, the whole chloroplast genome sequence of L. hancockii was de novo assembled by using high-throughput Illumina reads, and phylogenetic analysis was conducted within species of subtribe Aeridinae. The complete chloroplast genome sequence of L. hancockii was 146,243 bp in length, with a typical quadripartite structure, and its large single-copy, small single-copy, and inverted repeat were 84,441 bp, 11,412 bp, and 25,195 bp long, respectively. The GC content of the whole chloroplast genome was 36.6%, while the GC contents of LSC, SSC, and IR were 33.8%, 27.5%, and 43.3%, respectively. The chloroplast genome consisted of 129 genes, including 74 protein-coding genes, eight rRNAs, 38 tRNAs, and nine pseudogenes. Phylogenic tree was generated using the best model GTR + R, and the results showed that L. hancockii was sister to Holcoglossum and Vanda species, with a support of 100%.
Introduction
Orchidaceae is the second largest family in angiosperm, which consists of approximately 800 genera and over 25,000 species (Chase et al. Citation2015; Phillips et al. Citation2020). In China, there are more than 200 genera and over 1600 species, with 682 endemic to China (Zhou et al. Citation2016). Luisia is a small genus in Orchidaceae, and it is comprised of about 40 species in the world, and they are distributed in tropical and subtropical Asia, such as Bhutan, China, and India (Wu et al. Citation2009; Karuppusamy and Ravichandran Citation2019). About 11 Luisia species are distributed in China, and five are endemic. They are all herbs, epiphytic or lithophytic (Wu et al. Citation2009). L. hancockii is a perennial herb with tufted stems, obtuse leaves, and floral bracts broadly ovate, and it is often found on boulders, in rock crevices, or on tree trunks of Cinnamomum camphora (L.) Presl 1753, Castanopsis sclerophylla (Lindl. & Paxton) Schottky 1912, and Pterocarya stenoptera C. DC. 1862, according to our field investigation. L. hancockii is a medicinal plant that is commonly used to treat rheumarthritis, carbuncles, and laryngitis in folk medicine (Yao and Xiong Citation2016). To explore the phylogenomic relationship with other plants in subtribe Aeridinae (Orchidaceae), the chloroplast genome of L. hancockii was assembled, and a phylogenetic tree was generated. Our objectives were to investigate the features of L. hancockii chloroplast genome and to elucidate its phylogenetic relationship.
Materials and methods
Plant sampling
Leaf samples were harvested from Huayan Mountain (28°41′24″N, 121°06′51″E), Huangyan District, Taizhou City, Zhejiang Province, China (). A voucher specimen assigned CHS20180244 is deposited in Molecular Biology Innovation Laboratory at Taizhou University (Ming Jiang, [email protected]).
Figure 1. Luisia hancockii Rolfe 1896. (A) Habitat; (B) Flowers. Both photos were taken by Ming Jiang. L. hancockii is a perennial herb with a height of 10–20 cm. The flowers are fleshy, with sepals and petals in yellow-green color. The lip is nearly ovate-oblong, and lip hypochile is purple-red. The flowering period is from May to June.
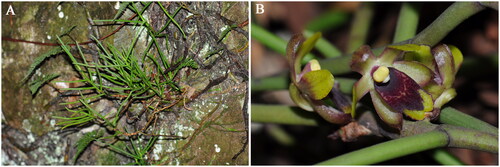
DNA isolation, sequencing, assembling, and annotation of the chloroplast genome
The CTAB (cetyltrimethylammonium bromide) method was used for DNA extraction (Doyle and Doyle Citation1987). A genomic DNA library was then constructed and sequenced using an Illumina Hiseq X Ten sequencing platform. Clean reads were obtained by filtering low-quality raw data reads with a Perl-based stand-alone program package NGSQCToolkit v2.3.3 (Patel and Jain Citation2012). As a result, a total of 11,362,518 high quality clean reads were generated, representing 3.41 G bases of nucleotide sequences. The whole chloroplast genome was de novo assembled by a Perl-based program NOVOPlasty (Dierckxsens et al. Citation2017). Annotation of the plastid genome was conducted using the Dual Organellar GenoMe Annotator (Wyman et al. Citation2004) combined with manual correction. tRNAscan-SE 2.0 was employed to predict tRNA, and RNAmmer was applied to identify rRNA genes (Lagesen et al. Citation2007; Chan and Lowe Citation2019). The whole chloroplast genome map of L. hancockii was drawn using CPGview (http://www.1kmpg.cn/cpgview/) (Liu et al. Citation2023).
Phylogenetic analysis
To determine the phylogenetic position of L. hancockii within subtribe Aeridinae (Orchidaceae), 17 chloroplast genome sequences downloaded from NCBI, along with a sequence of Cymbidium erythraeum Lindl. 1858 (MK820373) as the outgroup. Multiple alignments were then performed with MAFFT v7.450 (Katoh and Standley Citation2013). A phylogenetic tree was created using whole plastome sequences based on maximum-likelihood method by PhyML 3.1 (Guindon et al. Citation2010), under the best-fit substitution model GTR + R.
Results
The results revealed that the total length of the Luisia hancockii chloroplast genome covers 146,243 bp, exhibiting a typical circular quadripartite structure, with an average depth of ×460.68 (Figure S1). Its large single-copy (LSC), small single-copy (SSC), and inverted repeats (IRA and IRB) were 84,441 bp, 11,412 bp, and 25,195 bp long, respectively (). The GC content of the whole plastome was 36.6%, while those of LSC, SSC, and IRs were 33.8%, 27.5%, and 43.3%, respectively. The chloroplast genome sequence of L. hancockii is released and available now in GenBank under an accession number of OR030420.
Figure 2. The chloroplast genome map of Luisia hancockii. The map contains six tracks. From the center outward, the first track shows the dispersed repeats, which consist of direct and palindromic repeats, connected with red and green arcs. The second track indicates the long tandem repeats as short blue bars. The third track reveals the short tandem repeats or microsatellite sequences as short bars with different colors. The colors, type of repeat they represent, and the description of the repeat types are as follows: black: c (complex repeat); green: p1 (repeat unit size = 1); yellow: p2 (repeat unit size = 2); purple: p3 (repeat unit size = 3); blue: p4 (repeat unit size = 4); orange: p5 (repeat unit size = 5); red: p6 (repeat unit size = 6). The chloroplast genome contains an LSC region, an SSC region, and two IR regions, and they are shown on the fourth track. The GC content along the genome is shown on the fifth track. Genes are color-coded according to their functional classification. The transcription directions for the inner and outer genes are clockwise and anticlockwise, respectively. The bottom left corner indicates the key for the functional classification of the genes.
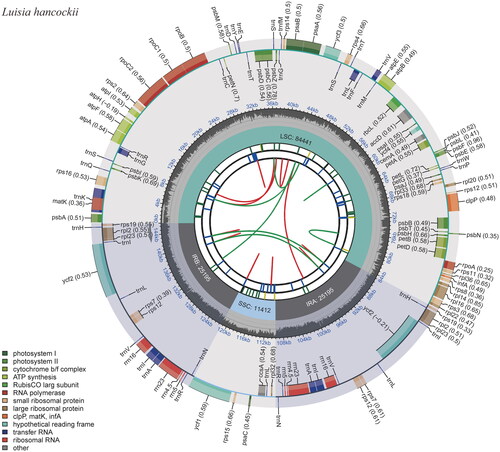
The chloroplast genome consisted of 129 genes, consisting of 74 protein-coding genes, eight rRNAs, 38 tRNAs, and nine pseudogenes. Pseudogenes included seven ndh genes (ndhG, ndhD, ndhE, two copies of ndhB, ndhJ, and ndhK) and two copies of ycf68. Two small subunit of ribosome genes (rpl2 and rpl23), three small subunit of ribosome genes (rps7, rps12, and rps19), four rRNA genes (rrn4.5, rrn5, rrn16, and rrn23), eight tRNA genes (trnA-UGC, trnH-GUG, trnI-CAU, trnI-GAU, trnL-CAA, trnN-GUU, trnR-ACG, and trnV-GAC), and two ycf genes (ycf2 and ycf68) were comprised of two copies. Genes like rps16, atpF, rpoC1, petB, petD, rpl16, and rpl2 contained one intron, while ycf3 and clpP hosted two introns (Figure S2). The rps12 is a trans-splicing gene (Figure S3). Phylogenetic tree based on complete chloroplast genomes revealed that 19 species could be divided into 10 groups, and the results were consistent with traditional morphology-based classification. L. hancockii clustered independently in group VI, and it was sister to Holcoglossum and Vanda species, with a support rate of 100% ().
Figure 3. The maximum-likelihood tree based on complete chloroplast genome sequences of Luisia hancockii and 17 species of subtribe Aeridinae, with Cymbidium erythraeum as the outgroup species. The numbers next to the nodes indicate bootstrap support values. NCBI accession numbers of each genome are shown in the figure. The following sequences were used: V. subconcolor MT180955 (Liu, Tu, Zhang, et al. 2020), Pomatocalpa spicatum MN124411 (Liu, Tu, Zhao, et al. Citation2020), Gastrochilus bigibbus MN124439 (Liu, Tu, Zhao, et al. Citation2020), G. obliquus MN124429 (Liu, Tu, Zhao, et al. Citation2020), G. gongshanensis MN124438 (Liu, Tu, Zhao, et al. Citation2020), G. guangtungensis MN124428 (Liu, Tu, Zhao, et al. Citation2020), G. formosanus MN124435 (Liu, Tu, Zhao, et al. Citation2020), Trichoglottis latisepala MN124432 (Liu, Tu, Zhao, et al. Citation2020), Acampe rigida MN124419 (Liu, Tu, Zhao, et al. Citation2020), A. papillosa MN124418 (Liu, Tu, Zhao, et al. Citation2020), Renanthera citrina NC_066482 (Xiao et al. Citation2022), Holcoglossum subulifolium MK442934 (Li et al. Citation2019), H. nagalandense NC_041514 (Li et al. Citation2019), Vanda brunnea NC_041522 (Li et al. Citation2019), V. concolor MK836105 (Chen et al. Citation2019), Phalaenopsis lowii NC_050652 (Wang et al. Citation2019), P. zhejiangensis MZ326749 (Jiang et al. Citation2021), and Cymbidium erythraeum MK820373 (Huang et al. Citation2019).
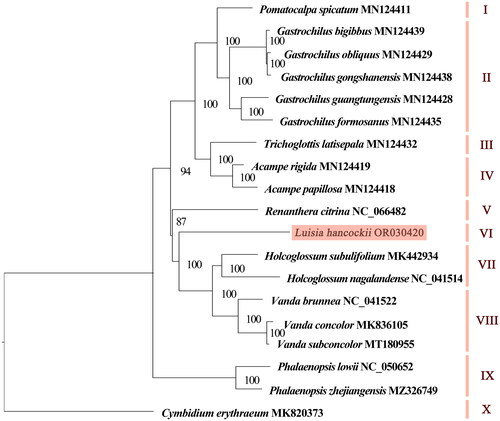
Discussion and conclusions
To our knowledge, this is the first complete chloroplast genome sequence in the genus Luisia. In our study, seven ndh genes were identified as pseudogenes because of the presence of internal stop codons, while ndhC was lost in L. hancockii plastome. The pseudolization and loss of ndh genes in some orchid species like P. equestris (Schauer) Rchb. 1850, Dendrobium officinale Kimura & Migo 1936, Goodyera fumata Thwaites 1861, and Masdevallia picturata Rchb. 1878 are common (Lin et al. Citation2015, Citation2017). Moreover, two copies of ycf68 genes were both pseudolized due to internal stop codons. Gene pseudolization in ycf68 was also reported in Utricularia reniformis Saint-Hilaire 1830 and Salix wilsonii Seemen 1905 (Silva et al. Citation2016; Chen et al. Citation2019).
In conclusion, the L. hancockii chloroplast genome was assembled and annotated for the first time, and the genome was determined to be 146,243 bp in length, containing a total of 129 genes. The phylogenetic tree revealed L. hancockii is closely related to Holcoglossum and Vanda species. Our study will provide insights into genetic conservation and phylogenetic studies in Orchidaceae.
Ethics statement
The collection of leaf samples conformed to the requirement of international ethics, which did not cause damage to the local environment. We complied with the rules and regulations of relevant institutional, national guidelines and legislation.
Author contributions
Ming Jiang and Junfeng Wang were responsible for the conception and design of the study. Leqin Huang, Zhenyu Lu, and Ming Jiang wrote the paper. Junfeng Wang, Leqin Huang, Zhenyu Lu, Ming Jiang, and Huijuan Zhang assembled and annotated the chloroplast genome. All of the authors were involved in the analysis and interpretation of the data, revising the manuscript critically for intellectual content, and gave the final approval of the version to be published. All authors have read and approved the final manuscript.
Supplemental Material
Download PDF (274.1 KB)Disclosure statement
No potential conflict of interest was reported by the authors.
Data availability statement
The data that support the findings of this study are openly available in GenBank of NCBI at https://www.ncbi.nlm.nih.gov/nuccore/OR030420. The associated BioProject, SRA, and Bio-Sample numbers are PRJNA974942, SRR24684304, and SAMN35302027, respectively.
Additional information
Funding
References
- Chan PP, Lowe TM. 2019. tRNAscan-SE: searching for tRNA genes in genomic sequences. Methods Mol Biol. 1962:1–14.
- Chase MW, Cameron KM, Freudenstein JV, Pridgeon AM, Salazar G, van den Berg C, Schuiteman A. 2015. An update classification of Orchidaceae. Bot J Linn Soc. 177(2):151–174. doi:10.1111/boj.12234.
- Chen JL, Tu XD, Liu DK, Ai Y. 2019. Complete plastid genome of Vanda concolor (Orchidaceae, Aeridinae). Mitochondrial DNA B Resour. 4(2):3687–3688. doi:10.1080/23802359.2019.1679049.
- Chen Y, Hu N, Wu H. 2019. Analyzing and characterizing the chloroplast genome of Salix wilsonii. Biomed Res Int. 2019:5190425. doi:10.1155/2019/5190425.
- Dierckxsens N, Mardulyn P, Smits G. 2017. NOVOPlasty: de novo assembly of organelle genomes from whole genome data. Nucleic Acids Res. 45(4):e18. doi:10.1093/nar/gkw955.
- Doyle JJ, Doyle JL. 1987. A rapid DNA isolation procedure for small quantities of fresh leaf tissue. Phytochem Bull. 19:11–15.
- Guindon S, Dufayard JF, Lefort V, Anisimova M, Hordijk W, Gascuel O. 2010. New algorithms and methods to estimate maximum-likelihood phylogenies: assessing the performance of PhyML 3.0. Syst Biol. 59(3):307–321. doi:10.1093/sysbio/syq010.
- Huang J, Chen GZ, Li TZ, Huang ZC, Rao WH, Chen JB. 2019. The complete chloroplast genome of Cymbidium erythraeum (Orchidaceae). Mitochondrial DNA B Resour. 4(2):2517–2518. doi:10.1080/23802359.2019.1638322.
- Jiang M, Zhu Y, Wu Q, Zhang H. 2021. Complete chloroplast genome of a rare and endangered plant species Phalaenopsis zhejiangensis: genomic features and phylogenetic relationship within Orchidaceae. Mitochondrial DNA B Resour. 6(10):2872–2879. doi:10.1080/23802359.2021.1972049.
- Karuppusamy S, Ravichandran V. 2019. A new species of Luisia (Orchidaceae: Epidendroideae: Vandeae) from the Western Ghats of India. Phytotaxa. 387(4):295. doi:10.11646/phytotaxa.387.4.3.
- Katoh K, Standley DM. 2013. MAFFT Multiple Sequence Alignment Software version 7: improvements in performance and usability. Mol Biol Evol. 30(4):772–780. doi:10.1093/molbev/mst010.
- Lagesen K, Hallin P, Rødland EA, Staerfeldt HH, Rognes T, Ussery DW. 2007. RNAmmer: consistent and rapid annotation of ribosomal RNA genes. Nucleic Acids Res. 35(9):3100–3108. doi:10.1093/nar/gkm160.
- Li ZH, Ma X, Wang DY, Li YX, Wang CW, Jin XH. 2019. Evolution of plastid genomes of Holcoglossum (Orchidaceae) with recent radiation. BMC Evol Biol. 19(1):63. doi:10.1186/s12862-019-1384-5.
- Lin CS, Chen JJ, Huang YT, Chan MT, Daniell H, Chang WJ, Hsu CT, Liao DC, Wu FH, Lin SY, et al. 2015. The location and translocation of ndh genes of chloroplast origin in the Orchidaceae family. Sci Rep. 5(1):9040. doi:10.1038/srep09040.
- Lin CS, Chen JJW, Chiu CC, Hsiao HCW, Yang CJ, Jin XH, Leebens-Mack J, de Pamphilis CW, Huang YT, Yang LH, et al. 2017. Concomitant loss of NDH complex-related genes within chloroplast and nuclear genomes in some orchids. Plant J. 90(5):994–1006. doi:10.1111/tpj.13525.
- Liu DK, Tu XD, Zhang S, Li MH. 2020. The complete plastid genome of Vanda subconcolor (Orchidaceae, Aeridinae). Mitochondrial DNA Part B. 5(2):1712–1713. doi:10.1080/23802359.2020.1749169.
- Liu DK, Tu XD, Zhao Z, Zeng MY, Zhang S, Ma L, Zhang GQ, Wang MM, Liu ZJ, Lan SR, et al. 2020. Plastid phylogenomic data yield new and robust insights into the phylogeny of Cleisostoma-Gastrochilus clades (Orchidaceae, Aeridinae). Mol Phylogenet Evol. 145:106729. doi:10.1016/j.ympev.2019.106729.
- Liu SY, Ni Y, Li JL, Zhang XY, Yang HY, Chen HM, Liu C. 2023. CPGview: a package for visualizing detailed chloroplast genome structures. Mol Ecol Resour. 23(3):694–704. doi:10.1111/1755-0998.13729.
- Patel RK, Jain M. 2012. NGS QC Toolkit: a toolkit for quality control of next generation sequencing data. PLOS One. 7(2):e30619. doi:10.1371/journal.pone.0030619.
- Phillips RD, Reiter N, Peakall R. 2020. Orchid conservation: from theory to practice. Ann Bot. 126(3):345–362. doi:10.1093/aob/mcaa093.
- Silva SR, Diaz YC, Penha HA, Pinheiro DG, Fernandes CC, Miranda VF, Michael TP, Varani AM. 2016. The chloroplast genome of Utricularia reniformis sheds light on the evolution of the ndh gene complex of terrestrial carnivorous plants from the Lentibulariaceae family. PLOS One. 11(10):e0165176. doi:10.1371/journal.pone.0165176.
- Wang JY, Liu ZJ, Zhang GQ, Peng CC. 2019. The complete chloroplast genome sequence of Phalaenopsis lowii (Orchidaceae). Mitochondrial DNA B Resour. 4(2):3569–3570. doi:10.1080/23802359.2019.1674715.
- Wu ZY, Raven PH, Hong DY. 2009. Flora of China. Vol. 25. Beijing; St. Louis: Science Press; Missouri Botanical Garden Press.
- Wyman SK, Jansen RK, Boore JL. 2004. Automatic annotation of organellar genomes with DOGMA. Bioinformatics. 20(17):3252–3255. doi:10.1093/bioinformatics/bth352.
- Xiao T, He L, Yue L, Zhang Y, Lee SY. 2022. Comparative phylogenetic analysis of complete plastid genomes of Renanthera (Orchidaceae). Front Genet. 13:998575. doi:10.3389/fgene.2022.998575.
- Yao ZS, Xiong YK. 2016. Summary of medicinal plant resources in Zhejiang. Shanghai: Shanghai Science and Technology Press.
- Zhou XX, Cheng ZQ, Liu QX, Zhang JL, Hu AQ, Huang MZ, Hu C, Tian HZ. 2016. An updated checklist of Orchidaceae in China, with two new national records. Phytotaxa. 276(1):1–148. doi:10.11646/phytotaxa.276.1.1.