Abstract
Salicornia europaea is a salt-tolerant eudicot species in the Amaranthaceae family that is widely distributed in coastal wetlands and other saline-alkali lands. In this study, the complete chloroplast genome of S. europaea was determined using Illumina paired-end sequencing technology. The genome was 153,163 bp in length with 132 genes, including 87 protein-coding genes, 37 tRNA genes, and eight rRNA genes. The genome displayed a quadripartite structure consisting of a small single-copy (SSC) region of 18,953 bp, a large single-copy (LSC) region of 84,566 bp, and two inverted repeats (IRs) of 49,644 bp, respectively. The phylogenetic analysis indicated that Salicornia europaea exhibited the closest relationship with S. bigelovii. This study contributes to the pool of salt-tolerant gene species and serves as a valuable reference for further research on Salicornia.
Introduction
Understanding the mechanisms behind salt tolerance in plants is crucial, as soil salinization has significantly impacted approximately 10% of the global surface and 50% of irrigated land (Duan et al. Citation2015; Zhang et al. Citation2020). Salicornia europaea (Linnaeus Citation1753), a highly salt-tolerant herb (Voronin et al. Citation2019; Duan et al. Citation2022) belonging to the Amaranthaceae family (Nikalje et al. Citation2018), plays a vital role in protecting coastal soils and improving coastal ecology (Cárdenas-Pérez et al. Citation2021). Plant salt tolerance, a polygenic trait, is regulated by various factors (Shabala and Cuin Citation2008). While the morphometric features of its chloroplasts have been investigated (Voronin et al. Citation2019), the complete genome of chloroplast of S. europaea is still unknown. It has been observed that S. europaea accumulated significant amounts of Na+, K+, Mg2+, and Ca2+ in shoots (Ozawa et al. Citation2009), making the genomic analysis of chloroplast in leaves particularly favorable for studying their salt tolerance.
Salicornia europaea possesses a unique salt tolerance mechanism (Shi et al. Citation2006), although only a limited number of salt tolerance genes have been identified (Harada et al. Citation2011). Many genes induced by salt stress are parts of the molecular network and play essential roles in multiple pathways (Berthomieu et al. Citation2003), such as photosynthesis, energy metabolism, signal recognition, and transmission (Rezaei Qusheh Bolagh et al. Citation2021). In this study, we have successfully obtained the whole chloroplast genome library of S. europaea. These findings will provide valuable genomic information regarding the chloroplast of salt-tolerant plants and serve as a basis for isolating and studying salt-tolerance genes.
Materials
The salt-tolerant plant, S. europaea (), was collected from Heze City, Shandong Province, China (35°14′54.6″ N, 115°26′42″ E) and photographed by Tianlong Liu. This species has been deposited in the Marine Natural Products Research and Development Laboratory, First Institute of Oceanography, Ministry of Natural Resources, China (Changfeng Qu, [email protected]) with the voucher number FIO2021081033.
Figure 1. Community (A), single plants (B), and macroscopic details (C) characteristics of Salicornia europaea. This photograph, taken by TianLong Liu in Heze City, Shandong Province, has been used with the author’s permission. High salt accumulation can be observed at the nodes of scale-like stems, where the tips of the pale green, sharp leaves do not develop. Conversely, salt accumulation is low at the base of connate, sheath-like, membranous-margined, multi-branched stems.
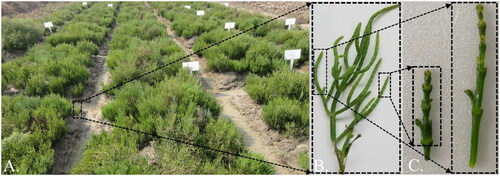
Methods
The whole genome library of S. europaea chloroplast was constructed by Shanghai Lingen Biological Technology Co. Ltd. (Shanghai, China). Genomic paired-end sequencing was performed using the Illumina NovaSeq 6000 platform. To improve the accuracy of subsequent assembly, Trimmomatic v0.39 (http://www.usadellab.org/cms/index.php?page=trimmomatic) was used for quality shearing of the original data. NOVOPlasty v4.2 software (https://github.com/ndierckx/NOVOPlasty) was utilized to assemble the chloroplast genome of S. europaea. The depth of coverage was calculated by mapping the reads onto the chloroplast genome sequence using bowtie2 v2.3.4.3, enabling assessment of the assembly correctness (Langmead and Salzberg Citation2012). Chloroplast Scaffolds were confirmed by alignment of NT libraries and sequences were linked according to overlap (Dierckxsens et al. Citation2017). The chloroplast genome’s protein-coding genes, tRNA, and rRNA were predicted using GeSeq software (https://chlorobox.mpimp-golm.mpg.de/geseq.html/). Chloroplast Genome Viewer (CPGView) was used to draw the circular map of the chloroplast genome (Liu et al. Citation2023).
To establish the phylogenetic relationship of S. europaea with other plant species in Amaranthaceae, the complete chloroplast genome sequences of 15 species from the Amaranthaceae family were obtained from GenBank. Additionally, Kalidium foliatum (NC_062599.1) was included as the out-groups. The sequences were aligned using MAFFT v7.388 (Katoh et al. Citation2002) and trimmed appropriately using trimAl (Capella-Gutiérrez et al. Citation2009). In MEGA 7.0, a phylogenetic tree was constructed: the statistical method was the neighbor-joining method, the test of phylogeny was the bootstrap method, the model was p-distance, and the number of bootstrap replications was 500.
Results
The total gene length of S. europaea is 153,163 bp, with an average depth of ×7344.92 (Supplementary 1). The G + C content in the chloroplast genome is 36.28%. Among the 132 genes in the chloroplast genome, there are 87 protein-coding genes, 37 tRNA genes, and eight rRNA genes. The circular genome is composed of a large single-copy (LSC, 84,566 bp) region, a small single-copy (SSC, 18,953 bp) region, and a pair of inverted repeat (IR, 49,644 bp) regions (). Fourteen genes contain one intron: rps16, atpF, rpoC1, petB, petD, rpl16, ndhB, ndhA, trnK-UUU, trnG-UCC, trnL-UAA, trnC, trnI-GAU, and trnA-UGC. The clpP, rps12, and ycf3 genes contain two introns. Furthermore, gene structure analysis was conducted for rps16, atpF, rpoC1, ycf3, clpP1, petB, petD, rpl16, ndhA, and ndhB, which were challenging to annotate (Supplementary 2). Meanwhile, all genes in the chloroplast genome were categorized and counted (Supplementary 3).
Figure 2. Chloroplast genome map of Salicornia europaea. The species name is labeled in the upper left corner. The map consists of six tracks from inside to outside. The sequence of forward and reverse repeats connected via the red and green arcs is represented by the first track (A). The blue bars on the second track (B) show a tandem repeat sequence. The microsatellite sequence is labeled on the yellow and green bars of the third track (C). The fourth track (D) shows the small single-copy (SSC), inverted repeat (IRa and IRb), and large single-copy (LSC) regions. The GC content and gene names of the genome are shown on track five (E) and track six (F), respectively. The contents of the round brackets after the outermost gene name indicate optional codon usage deviations. Genes drawn outside the outer circle are transcribed counterclockwise, and genes drawn inside the outer circle are transcribed clockwise. Genes belonging to different functional groups are color-coded. The different colored legends in the bottom left corner indicate genes with different functions.
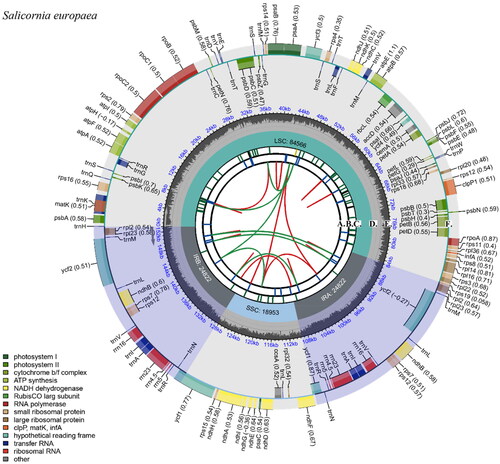
The phylogenetic tree indicated that S. europaea was most closely related to S. bigelovii (KJ629117) (). Therefore, the complete chloroplast genome of S. europaea will provide valuable information for molecular identification, genetic diversity analysis, and gene resource exploration of Salicornia.
Figure 3. Phylogenetic tree constructed based on MEGA 7.0. The accession numbers of the coding sequences used for the tree are as follows: Salicornia europaea OL449699, Salicornia bigelovii KJ629117.1 (Jamdade et al. Citation2022), Salicornia brachiata KJ629115.1 (Jamdade et al. Citation2022), Salsola abrotanoides NC057096.1, Salsola collina OK189514.1, Suaeda malacosperma NC039180.1, Suaeda salsa NC045302.1, Amaranthus tricolor OM419215.1, Amaranthus hybridus NC053787.1, Amaranthus blitum MT526777.1, Amaranthus albus NC065857.1, Salsola affinis ON080842.1, Beta macrocarpa NC069016.1, Beta patula NC059009.1, and Kalidium foliatum NC062599.1. The bootstrap support values are shown on the nodes.
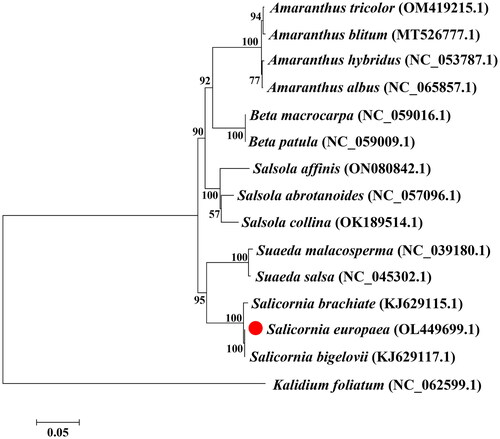
Discussion and conclusions
This study reported the complete chloroplast genome of Salicornia europaea. Consistent with previous studies, the structure and classification of this chloroplast genome both suggested that the involved salt tolerance genes of S. europaea may play a role in several biological processes such as photosynthesis, energy conversion, and material decomposition, which coexist in a larger molecular network. This finding will provide genomic information on the salt tolerance mechanism of Salicornia. However, further study of salt tolerance genes and their specific functions is necessary for a more comprehensive exploration.
Author contributions
Drafting the manuscript and acquisition, analysis, and interpretation of data for the work: Tianlong Liu; sample collection and acquisition, interpretation of data: Zhicong Zuo, Yingying He, and Jinlai Miao; final approval of the version to be published, revising the manuscript critically, conception and design of the work: Jia Yu and Changfeng Qu. All the listed authors have approved the manuscript, and agree to be accountable for all aspects of the work.
Ethical approval
Ethical approval for the study was obtained from the Ethical Committee of Qingdao University.
Supplemental Material
Download PDF (5.4 KB)Supplemental Material
Download PDF (6.7 KB)Supplemental Material
Download PDF (142.1 KB)Supplemental Material
Download MS Word (406.7 KB)Supplemental Material
Download PDF (697.6 KB)Disclosure statement
No potential competing interest was reported by the authors.
Data availability statement
The genome sequence data of this study are available in GenBank of NCBI at https://www.ncbi.nlm.nih.gov/ under accession no. OL449699, and the associated Bio-Project, SRA, and Bio-Sample numbers are PRJNA834948, SRR19078398, and SAMN30723140, respectively.
Additional information
Funding
References
- Berthomieu P, Conéjéro G, Nublat A, Brackenbury WJ, Lambert C, Savio C, Uozumi N, Oiki S, Yamada K, Cellier F, et al. 2003. Functional analysis of AtHKT1 in Arabidopsis shows that Na+ recirculation by the phloem is crucial for salt tolerance. EMBO J. 22(9):2004–2014. doi: 10.1093/emboj/cdg207.
- Capella-Gutiérrez S, Silla-Martínez JM, Gabaldón T. 2009. trimAl: a tool for automated alignment trimming in large-scale phylogenetic analyses. Bioinformatics. 25(15):1972–1973. doi: 10.1093/bioinformatics/btp348.
- Cárdenas-Pérez S, Piernik A, Chanona-Pérez JJ, Grigore MN, Perea-Flores MJ. 2021. An overview of the emerging trends of the Salicornia L. genus as a sustainable crop. Environ Exp Bot. 191:104606. doi: 10.1016/j.envexpbot.2021.104606.
- Dierckxsens N, Mardulyn P, Smits G. 2017. NOVOPlasty: de novo assembly of organelle genomes from whole genome data. Nucleic Acids Res. 45(4):e18. doi: 10.1093/nar/gkw955.
- Duan H, Tiika RJ, Tian F, Lu Y, Zhang Q, Hu Y, Cui G, Yang H. 2022. Metabolomics analysis unveils important changes involved in the salt tolerance of Salicornia europaea. Front Plant Sci. 13:1097076. doi: 10.3389/fpls.2022.1097076.
- Duan H-R, Ma Q, Zhang J-L, Hu J, Bao A-K, Wei L, Wang Q, Luan S, Wang S-M. 2015. The inward-rectifying K+ channel SsAKT1 is a candidate involved in K+ uptake in the halophyte Suaeda salsa under saline condition. Plant Soil. 395(1–2):173–187. doi: 10.1007/s11104-015-2539-9.
- Harada T, Torii Y, Morita S, Onodera R, Hara Y, Yokoyama R, Nishitani K, Satoh S. 2011. Cloning, characterization, and expression of xyloglucan endotransglucosylase/hydrolase and expansin genes associated with petal growth and development during carnation flower opening. J Exp Bot. 62(2):815–823. doi: 10.1093/jxb/erq319.
- Jamdade R, Al-Shaer K, Al-Sallani M, Al-Harthi E, Mahmoud T, Gairola S, Shabana HA. 2022. Multilocus marker-based delimitation of Salicornia persica and its population discrimination assisted by supervised machine learning approach. PLOS One. 17(7):e0270463. doi: 10.1371/journal.pone.0270463.
- Katoh K, Misawa K, Kuma K, Miyata T. 2002. MAFFT: a novel method for rapid multiple sequence alignment based on fast Fourier transform. Nucleic Acids Res. 30(14):3059–3066. doi: 10.1093/nar/gkf436.
- Langmead B, Salzberg SL. 2012. Fast gapped-read alignment with Bowtie 2. Nat Methods. 9(4):357–359. doi: 10.1038/nmeth.1923.
- Linnaeus C. 1753. Species Plantarum. Sweden: Arkose Press.
- Liu S, Ni Y, Li J, Zhang X, Yang H, Chen H, Liu C. 2023. CPGView: a package for visualizing detailed chloroplast genome structures. Mol Ecol Resour. 23(3):694–704. doi: 10.1111/1755-0998.13729.
- Nikalje GC, Srivastava AK, Pandey GK, Suprasanna P. 2018. Halophytes in biosaline agriculture: mechanism, utilization, and value addition. Land Degrad Dev. 29(4):1081–1095. doi: 10.1002/ldr.2819.
- Ozawa T, Miura M, Fukuda M, Kakuta S. 2009. Cadmium tolerance and accumulation in a halophyte Salicornia europaea as a new candidate for phytoremediation of saline soils. Sci Rep Grad Sch Life Environ Sci. 60:1–8.
- Rezaei Qusheh Bolagh F, Solouki A, Tohidfar M, Zare Mehrjerdi M, Izadi-Darbandi A, Vahdati K. 2021. Agrobacterium-mediated transformation of Persian walnut using BADH gene for salt and drought tolerance. J Hortic Sci Biotechnol. 96(2):162–171. doi: 10.1080/14620316.2020.1812446.
- Shabala S, Cuin TA. 2008. Potassium transport and plant salt tolerance. Physiol Plant. 133(4):651–669. doi: 10.1111/j.1399-3054.2007.01008.x.
- Shi X, Han H, Shi W, Li Y. 2006. NaCl and TDZ are two key factors for the improvement of in vitro regeneration rate of Salicornia europaea L. J Integr Plant Biol. 48(10):1185–1189. doi: 10.1111/j.1744-7909.2006.00342.x.
- Voronin PY, Ivanova LA, Ronzhina DA, Myasoedov NA, Balnokin YV. 2019. Morphometric characterization of halophytic plant chloroplasts. Russ J Plant Physiol. 66(5):707–711. doi: 10.1134/S1021443719050200.
- Zhang H, Feng H, Zhang J, Ge R, Zhang L, Wang Y, Li L, Wei J, Li R. 2020. Emerging crosstalk between two signaling pathways coordinates K+ and Na+ homeostasis in the halophyte Hordeum brevisubulatum. J Exp Bot. 71(14):4345–4358. doi: 10.1093/jxb/eraa191.