Abstract
Theobroma bicolor Bonpl. 1806 is distributed in the Neotropics from southern Mexico to the Peruvian and Brazilian Amazon. High-throughput sequencing of T. bicolor from Peru (KUELAP2926) resulted in the assembly of its complete plastid genome (GenBank accession number OQ557154). The chloroplast genome of T. bicolor is A + T-rich (62.97%), having 160,317 bp in size and containing 130 genes; including a pair of inverted repeat regions (IRs) of 25,462 bp separated by a large single copy region (LSC) of 89,221 bp and a small single copy region (SSC) of 20,172 bp. This plastid genome is similar in length, content, and organization to other members of the genus Theobroma. Phylogenetic analyses of T. bicolor support its sistership to the clade comprising T. cacao and T. grandiflorum. This study may contribute valuable information to the phylogenetic relationships within the genus Theobroma.
Introduction
The genus Theobroma L. belongs to the family Malvaceae and comprises 22 species (Bayer et al. Citation1999). One of this species, Theobroma bicolor has a wide distribution in the Neotropics, ranging from southern Mexico to the Peruvian and Brazilian Amazon (Aikpokpodion Citation2012; GBIF Citation2020). It is also considered to have significant economic potential (Ponce-Sánchez et al. Citation2021). In Colombia and Peru, T. bicolor is called as macambo or maraco (Barrera et al. Citation2006). The pulp of this species is used to make juices, jams and traditional beverages (Kufer and McNeil Citation2006; Gálvez-Marroquín et al. Citation2016). The seeds are used as snacks and are used to make a type of chocolate called bacalate (Mantilla et al. Citation2008; Gonzáles and Torres Citation2010), which has good quality butter containing 42.0% of fatty acids (Torres et al. Citation2002). T. bicolor beans have lower phenol levels compared to T. cacao, which has the potential to improve the flavor and nutritional quality of cocoa products (Febrianto and Zhu Citation2022). Additionally, T. bicolor is resistant to pests and diseases. For instance, Moniliophthora roreri and Moniliophthora perniciosa commonly attack T. cacao, but do not generate strong affection on T. bicolor (Lagneaux et al. Citation2021). These special traits in T. bicolor offer great potential in the genetic improvement of T. cacao and also might enhance the flavor and nutritional quality of T. cacao derivatives such as chocolate, butter and cocoa powder (Torres et al. Citation2002; Febrianto and Zhu Citation2022). However, few studies related to its chemical characterization, post-harvesting management, and genomic analyses are available in comparison to its relative T. cacao (González et al. Citation2016; Ponce-Sánchez et al. Citation2021). It was confirmed that the plastidial genome has useful information for topics such as population genetics, evolution, and biotechnology due to its maternal inheritance (Rogalski et al. Citation2015). Accordingly, the aim of this study was decoding the complete chloroplast genome of T. bicolor from Peru using high throughput sequencing technology.
Materials and methods
The specimen of T. bicolor was collected in March 2022. This specimen was an adult flowering plant, approximately 30 years old (). Tissue samples of approximately 50 mm2 were taken from leaf tips () for genomic analyses and placed in prelabelled 1.5 mL Safelock Eppendorf tubes with the addition of silica gel. In addition, samples of leaves (), flowers and fruits () of T. bicolor were collected () and deposited at the herbarium of Universidad Nacional Toribio Rodriguez de Mendoza (KUELAP, https://www.untrm.edu.pe, Curator Eli Pariente, email: [email protected]) under the voucher number KUELAP2926 (collected by Oswaldo Ananco from Condorcanqui, Peruvian Amazonas region; −4.245242 S, −77.722025 W).
Figure 1. Morphology of Theobroma bicolor (KUELAP-2926). (A) Habit showing tree with branched clusters. (B) Complex leaf. (C) Immature fruits oblong-ellipsoid in shape. All images have been obtained from Oswaldo Ananco from the province of Condorcanqui, Amazonas region.
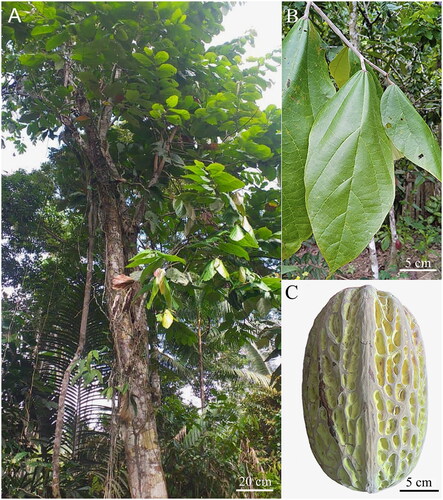
DNA genomic was extracted from T. bicolor (Specimen Voucher- KUELAP-2926) using the kit NucleoSpin (Macherey-Nagel, Düren, Germany) following the manufacturer’s instructions. The 150 bp PE Illumina library construction and sequencing was performed using the HiSeq 6000 platform by Macrogen (Seoul, South Korea). The genome was assembled using default de novo settings in MEGAHIT (Li et al. Citation2015) and Geneious Prime 2023.2 (https://www.geneious.com) to close gaps. Sequencing depth and coverage was calculated following Yang et al. (Citation2023). The genes were annotated manually using blastx, NCBI ORFfinder, and tRNAscan-SE 2.0 (Lowe and Chan Citation2016). The T. bicolor plastid genome was aligned to other plastomes using MAFFT (Katoh and Standley Citation2013). The Maximum Likelihood and Bayesian Inference phylogenetic tree was constructed using IQ-TREE v.2.2.0 software (Trifinopoulos et al. Citation2016) with 1500 ultrafast bootstrap replicates and the test model (-m TEST) (Minh et al. Citation2020). The tree was visualized with TreeDyn 198.3 at Phylogeny.fr (Dereeper et al. Citation2008).
Results
Genome organization and composition
The plastid genome of T. bicolor is 160,317 bp in length and contains 130 genes (), including a pair of inverted repeat regions (IRs) of 25,462 bp separated by a large single-copy region (LSC) of 89,221 bp and a small single copy (SSC) region of 20,172 bp. The maximum, minimum and average sequencing depth was 1926, 29, and 121.63, respectively (Figure S1). This plastid genome is A + T rich (62.97%), including 25 ribosomal proteins, 37 tRNA (trnA, trnG, trnN, and trnT occur in duplicates; trnS, trnR and trnV occur in triplicates, trnI and trnL occur in quadruplicate), 21 photosystem I and II, six ycf, seven cytochrome b/f complex, six ATP synthase, four RNA polymerase, eight rRNA, and 16 other genes (accD, cemA, ccsA, ndhA, ndhB, ndhC, ndhD, ndhE, ndhF, ndhG, ndhH, ndhI, ndhJ, ndhK, psbN). Additionally, 13 cis-splicing (atpF, clpP1, ndhA, ndhB, petB, petD, rpoC1, rpl2, rpl16, rps16, ycf1) and one trans-splicing genes were identified (rps12) (Figures S2 and S3). Fifty-four of the 130 genes are transcribed on the forward strand and the remaining 76 are coded on the reverse strand (; ).
Figure 2. Schematic map of overall features of the chloroplast genome of Theobroma bicolor. The map contains six tracks in default. From the center outward, the first track shows the dispersed repeats connected with arcs. The second track shows the long tandem repeats as short bars. The third track shows the short tandem repeats or microsatellite sequences as short bars. The small single-copy (SSC), inverted repeat (IRs), and large single-copy (LSC) regions are shown on the fourth track. The GC content along the genome is plotted on the fifth track. The genes are shown on the sixth track. The optional codon usage bias is displayed in the parenthesis after the gene name. Genes are coded by their functional classification. The transcription directions for the inner and outer genes are clockwise and anticlockwise, respectively. The functional classification of the genes is shown in the bottom left corner.
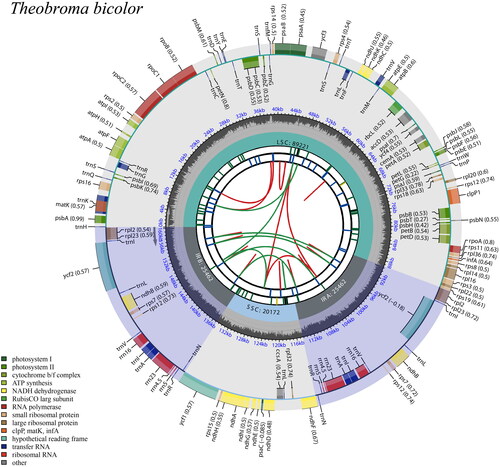
Figure 3. Maximum likelihood phylogram of Theobroma bicolor (OQ557154) and related genera. These genera are grouped in their subfamilies. For instance, the subfamiliy Grewioideae is based on the genus Grewia (Narkthai & Chantaranothai, Citation2020); whereas the subfamiliy Byttnerioideae is based on the genus Byttneria (Colli-Silva & Pirani Citation2020). Numbers along branches are RaxML bootstrap supports based on 1500 replicates. The legend below represents the scale for nucleotide substitutions.
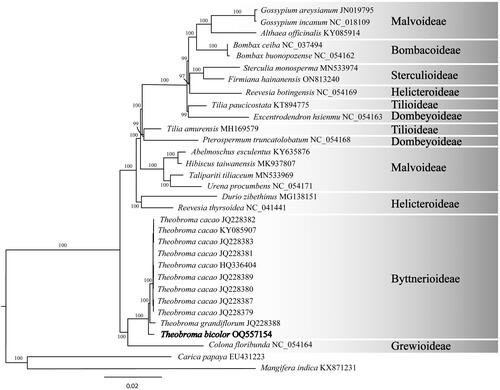
Table 1. Characteristics of plastid genome among species of T. bicolor, T. grandiflorum and T. cacao.
Phylogenetic analysis
Highly supported nodes in the phylogenetic analysis of plastid genome sequences of T. bicolor and other species of Malvales confirmed that T. bicolor is sister to the clade comprising T. grandiflorum and T. cacao. These three species are grouped in the subfamily Byttnerioideae, which is in a sister relationship to the subfamily Grewioideae.
Discussion
Twenty species have been accepted in the genus Theobroma (Govaerts et al. Citation2021) and this study has assembled the third complete plastid of genome for this genus. The plastid genome of T. bicolor is highly conserved in length, content, and organization to other species currently assigned to Theobroma (, Figure S4). Although the plastid genome of T. bicolor is slightly shorter (160,317 bp) than T. cacao (160,604 bp) and T. grandiflorum (160,619 bp) (Abdullah et al. Citation2020), the total GC content is slightly higher in T. bicolor. This increase is justified by the higher percentages of GC content in LSC, SSC, and rRNA. Conversely, it was suggested that GC content is substantially higher in IR genes (Yang et al. Citation2022). Phylogenomic analysis of the T. bicolor plastid genome fully resolved it in a clade sister to T. grandiflorum and T. cacao. This evolutionary relationship is congruent to recent multilocus phylogenies among Theobroma species (Niu et al. Citation2019; Abdullah et al. Citation2020). Pairwise genetic distances of the plastid genomes of T. bicolor and T. grandiflorum are 0.37%; whereas T. bicolor and T. cacao are 0.32%. These interspecific differences are useful for establishing a genetic threshold to delimit species in the genus Theobroma (Kuhn et al. Citation2010; Liu et al.Citation2018). Conclusively, this contribution provide a useful resource for conservation and a valuable framework to fully resolve the phylogenetic relationships of the genus Theobroma.
Authors contributions
DT, DEB, and MSC designed the research. DT, OA, JLM, and MO generated data for analysis. DEB, MSC, DT, AFHP, and OA analyzed the data and conducted experiments. DEB, MSC, JLM, and MO supervised the research. DEB, MSC, DT, AFHP, OA, JLM, and MO drafted the work and revised it critically. All authors agree to be accountable for all aspects of the work. All authors have read and approved the manuscript.
Ethics statement
Permit for sample collection and scientific research of wild flora (AUT-IFL-2020-0051-MINAGRI-SERFOR-DGGSPFFS-DGSPF) was provided by Servicio Nacional Forestal y de Fauna Silvestre (SERFOR).
Supplemental Material
Download JPEG Image (853.8 KB)Supplemental Material
Download JPEG Image (308.5 KB)Supplemental Material
Download JPEG Image (1.4 MB)Supplemental Material
Download JPEG Image (942 KB)Disclosure statement
The authors report no conflicts of interest. The authors alone are responsible for the content and writing of this article.
Data availability statement
The genome sequence data that support the findings of this study are openly available in GenBank of NCBI at https://www.ncbi.nlm.nih.gov/ under the accession no. OQ557154. The associated BioProject, BioSample, and SRA numbers are PRJNA944261, SAMN33745908, and SRR23852829, respectively.
Additional information
Funding
References
- Abdullah, Waseem S, Mirza B, Ahmed I, Waheed MT. 2020. Comparative analyses of chloroplast genomes of Theobroma cacao and Theobroma grandiflorum. Biologia. 75(5): 761–771. doi:10.2478/s11756-019-00388-8.
- Aikpokpodion P. 2012. Phenology of fowering in cacao (Theobroma cacao) and its related species in Nigeria. Afr J Agric Res. 7:3395–3402.
- Barrera JA, Hernández MS, Vargas G, Martínez O, Melgarejo LM, Casas AE, Zambrano JE, Bedoya CD. 2006. Caracterización del crecimiento y desarrollo vegetativo de especies promisorias del género Theobroma bajo condiciones de la Amazonia colombiana. 1st edn. Bogotá, Colombia: Theobroma; p. 65–100.
- Bayer C, Fay MF, Bruijn AY, Savolainen V, Morton CM, Kubitzki K, Alverson WS, Chase MW. 1999. Support for an expanded family concept of Malvaceae within a recircumscribed order Malvales: a combined analysis of plastid atpB and rbcL DNA sequences. Bot J Linn Soc. 129(4):267–303. doi:10.1111/j.1095-8339.1999.tb00505.x.
- Colli-Silva M, Pirani JR. 2020. Estimating bioregions and undercollected areas in South America by revisiting Byttnerioideae, Helicteroideae and Sterculioideae (Malvaceae) occurrence data. Flora. 271:151688. doi:10.1016/j.flora.2020.151688.
- Dereeper A, Guignon V, Blanc G, Audic S, Buffet S, Chevenet F, Dufayard JF, Guindon S, Lefort V, Lescot M, et al. 2008. Phylogeny.fr: robust phylogenetic analysis for the non-specialist. Nucleic Acids Res. 36:W465–W469. doi:10.1093/nar/gkn180.
- Febrianto NA, Zhu F. 2022. Comparison of bioactive components and flavor volatiles of diverse cocoa genotypes of Theobroma grandiflorum, Theobroma bicolor, Theobroma subincanum and Theobroma cacao. Food Res Int. 161:111764. doi:10.1016/j.foodres.2022.111764.
- Gálvez-Marroquín L, Reyes-Reyes A, Avendaño-Arrazate C, Hernández-Gómez E, Díaz-Fuentes VH, Mendoza-López A. 2016. Pataxte (Theobroma bicolor Humb. & Bonpl.): especie subutilizada en México. Agroproductividad. 9:41–47.
- GBIF. 2020. Theobroma bicolor Humb. & Bonpl. in GBIF Secretariat. Global Biodiversity Information Facility Backbone Taxonomy. Checklist dataset doi:10.15468/dl.vjk22s%0A. Accessed via GBIF.org
- González AA, Moncada J, Idarraga A, Rosenberg M, Cardona CA. 2016. Potential of the amazonian exotic fruit for biorefineries: the Theobroma bicolor (Makambo) case. Ind Crops Prod. 86:58–67. doi:10.1016/j.indcrop.2016.02.015.
- Gonzáles A, Torres G. 2010. Manual Cultivo de Macambo. 1st ed. Perú: IIAP.
- Govaerts R, Nic Lughadha E, Black N, Turner R, Paton A. 2021. The world checklist of vascular plants, a continuously updated resource for exploring global plant diversity. Sci Data. 8(1):215. doi:10.1038/s41597-021-00997-6.
- Katoh K, Standley DM. 2013. MAFFT multiple sequence alignment software version 7: improvements in performance and usability. Mol Biol Evol. 30(4):772–780. doi:10.1093/molbev/mst010.
- Kufer J, McNeil C. 2006. The jaguar tree (Theobroma bicolor Bompl.). A cultural history of Cacao. In: Chocolate in Mesoamerica, Cameron Mc. Ainesville: University Press of Florida; p. 90–104.
- Kuhn DN, Figueira A, Lopes U, Motamayor JC, Meerow AW, Cariaga K, Freeman B, Livingstone DS, Schnell RJ. 2010. Evaluating Theobroma grandiflorum for comparative genomic studies with Theobroma cacao. Tree Genet. Genomes. 6(5):783–792. doi:10.1007/s11295-010-0291-0.
- Lagneaux E, Andreotti F, Neher CM. 2021. Cacao, copoazu and macambo: Exploring Theobroma diversity in smallholder agroforestry systems of the Peruvian Amazon. Agroforest Syst. 95(7):1359–1368. doi:10.1007/s10457-021-00610-0.
- Li D, Liu C-M, Luo R, Sadakane K, Lam T-W. 2015. MEGAHIT: an ultra-fastsingle-node solution for large and complex metagenomics assembly via succinct de Bruijn graph. Bioinformatics. 31(10):1674–1676. doi:10.1093/bioinformatics/btv033.
- Liu J, Niu YF, Ni SB, He XY, Zheng C, Liu ZY, Cai HH, Shi C. 2018. The whole chloroplast genome sequence of Macadamia tetraphylla (Proteaceae). Mitochondrial DNA B Resour. 3(2):1276–1277. doi:10.1080/23802359.2018.1532836.
- Lowe TM, Chan PP. 2016. tRNAscan-SE on-line: search and contextual analysis of transfer RNA Genes. Nucleic Acids Res. 44(W1):W54–W57. doi:10.1093/nar/gkw413.
- Mantilla L, Piñeres R, Fonseca D. 2008. Colombia Frutas de la amazonia. Bogotá, Colombia: Instituto Sinchi.
- Minh BQ, Schmidt HA, Chernomor O, Schrempf D, Woodhams MD, von Haeseler A, Lanfear R. 2020. IQ-TREE 2: new models and efficient methods for phylogenetic inference in the genomic era. Mol Biol Evol. 37(5):1530–1534. doi:10.1093/molbev/msaa015.
- Narkthai P, Chantaranothai P. 2020. Taxonomic notes on the subfamily Grewioideae (Malvaceae) in Thailand. Thai Forest Bull. 48(1):72–76. doi:10.20531/tfb.2020.48.1.12.
- Niu YF, Ni SB, Liu J. 2019. The complete chloroplast genome of Theobroma grandiflorum, an important tropical crop. Mitochondrial DNA B Resour. 4(2):4157–4158. doi:10.1080/23802359.2019.1693291.
- Ponce-Sánchez J, Zurita-Benavides MG, Peñuela MC. 2021. Reproductive ecology of white cacao (Theobroma bicolor Humb. & Bonpl.) in Ecuador, western Amazonia: floral visitors and the impact of fungus and mistletoe on fruit production. Braz J Bot. 44:479–489.
- Rogalski M, do Nascimento VL, Fraga HP, Guerra MP. 2015. Plastid genomics in horticultural species: importance and applications for plant population genetics, evolution, and biotechnology. Front Plant Sci. 6:586. doi:10.3389/fpls.2015.00586.
- Trifinopoulos J, Nguye LT, von Haeseler A, Minh BQ. 2016. W-IQ-TREE: a fast online phylogenetic tool for maximum likelihood analysis. Nucleic Acids Res. 44(W1):W232–W235. doi:10.1093/nar/gkw256.
- Torres DEG, Assunção D, Mancini P, Torres RP, Mancini-Filho J. 2002. Antioxidant activity of macambo (Theobroma bicolor L.) extracts. Eur J Lipid Sci Technol. 104(5):278–281. doi:10.1002/1438-9312(200205)104:5<278::AID-EJLT278>3.0.CO;2-K.
- Yang N, Jingling L, Chang Z, Chang L. 2023. Generating sequencing depth and coverage map for organelle genomes. Protocols io. doi:10.17504/protocols.io.4r3l27jkxg1y/v1.
- Yang T, Sahu SK, Yang L, Liu Y, Mu W, Liu X, Strube ML, Liu H, Zhong B. 2022. Comparative analyses of 3,654 plastid genomes unravel insights into evolutionary dynamics and phylogenetic discordance of green plants. Front Plant Sci. 13:808156. doi:10.3389/fpls.2022.808156.