Abstract
The humped rockcod, Gobionotothen gibberifrons, is an Antarctic fish of the genus Gobionotothen in the family Nototheniidae and order Perciformes. To date, little biological information has been recorded about the genus Gobionotothen. Here, we report the first complete mitogenome of the genus Gobionotothen. The mitochondrial genome of G. gibberifrons is 18,631 bp in length, comprising 13 protein-coding genes, 24 tRNA genes (trnP-UGG and trnT-UGU were duplicated), 2 rRNA genes, and non-coding control regions. The base composition was 53.74% for A + T and 46.26% for G + C. This new mitochondrial genome of G. gibberifrons provides basic information for further phylogenetic analysis, suggesting the necessity to exploit a variety of newly discovered mitogenome sequences to infer inconclusive evolutionary relationships in Antarctic fishes.
Introduction
The humped rockcod Gobionotothen gibberifrons, also known as the humphead notothen, belongs to the family Nototheniidae. They have been found along the northwestern Antarctic Peninsula, the South Sandwich Islands, and South Georgia (Matschiner et al. Citation2009). According to FishBase (Froese and Pauly Citation2021), there are five species in the genus Gobionotothen. Currently, there is no complete mitochondrial genome from the Gobionotothen genus and only basic biological information is available. In this study, we report the first complete mitochondrial genome from G. gibberifrons (GenBank accession No. OP804623) along with a phylogenetic tree, which will provide information for a better understanding of evolutionary relationships among Antarctic fish.
Materials and methods
The G. gibberifrons sample was obtained from the Jeong Il Corporation. The sample was caught as a by-catch species from subareas 48.1 and 48.2 of the convention area (Supplementary Figure S1) between December 2020 and February 2021. The sample was frozen at −20 °C and transported to the Korea Polar Research Institute (KOPRI), Incheon, Korea. The specimen was deposited at the KOPRI (https://www.kopri.re.kr/eng/, Jin-Hyoung Kim, [email protected]) under the voucher number (Antarctic fish_013).
Total genomic DNA was extracted from muscle tissue using a standard phenol-chloroform-isoamyl alcohol extraction method (Sambrook et al. Citation1989). The specimen underwent morphological identification based on distinctive characteristics, such as a humped forehead, a yellowish coloring on the upper part of the head and body embellished with irregular dark spots and blotches, along with fins marked by brown spots, consistent with descriptions in previous studies (Gon and Heemstra Citation1990; DeWitt et al. Citation1990; Miller Citation1993) (). Subsequently, molecular identification of the species was conducted through the amplification of the mitochondrial cytochrome c oxidase subunit 1 (COX1) gene using universal Fish F1 and R1 primers (Ward et al. Citation2005), followed by sequencing and comparison through a NCBI BLASTn search (Camacho et al. Citation2009). Sequencing libraries of 550 bp insert size were prepared using the Illumina TruSeq Nano DNA Library Prep Kit (Illumina Inc., San Diego, CA) by manufacturer’s instructions. Sequencing was performed on an Illumina MiSeq platform (Illumina Inc., San Diego, CA) at the PHYZEN Co. Total 2,432,019,800 bp were generated from 8,079,800 reads. Before assembly, raw reads were quality-filtered and trimmed using a base quality threshold of Q20 using the QIAGEN CLC Assembly Cell package (Version 4.21, CLC Inc., Aarhus, Denmark). The trimmed data was assembled into a complete mitochondrial genome using the QIAGEN CLC Assembly Cell package. The coverage depth map is presented in Supplementary Figure S2, with an average mapping depth of 288.19. The genome was annotated using the GeSeq program (Tillich et al. Citation2017) and the Artemis annotation tool (Rutherford et al. Citation2000) with NCBI BLASTn search results for five closely related species, including Aethotaxis mitopteryx, Notothenia coriiceps, N. rossii, Pleuragramma antarcticum, and Pogonophryne albipinna (Camacho et al. Citation2009). The tRNA genes were checked by using the tRNAscan-SE (Lowe and Chan Citation2016; Sato et al. Citation2018).
Figure 1. A photo of the humped rockcod Gobionotothen gibberifrons (Lönnberg 1905). The photo has been taken by Jin-Hyoung Kim.
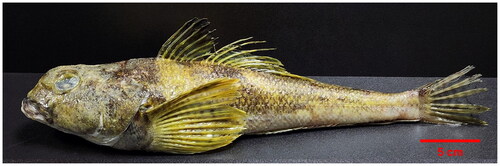
Phylogenetic analysis was conducted using the mitogenome sequences of 13 Antarctic fish species belonging to the family Nototheniidae and one non-Antarctic fish species as an outgroup, with all sequences obtained from NCBI GenBank. The amino acid sequences of 13 protein-coding genes from 14 species were aligned individually using Clustal W (Larkin et al. Citation2007) with default parameters. The alignments were refined by removing gaps or poorly aligned sites through Gblocks 0.91b (Castresana Citation2000; Dereeper et al. Citation2008) with default parameters. Then, all refined alignments were concatenated into a single multiple sequence alignment. The phylogenetic tree was analyzed using a maximum likelihood method in MEGA11 (Tamura et al. Citation2021) with 1,000 bootstrap replicates and a JTT + G + I + F model (Jones et al. Citation1992). The best-fit substitution model was selected using the Find Best DNA/Protein Model tool in MEGA 11. Gadus morhua was chosen as an outgroup, which has less close relationship with the ingroup but shares a common ancestor.
Results
The complete mitogenome of G. gibberifrons (GenBank accession No. OP804623) is 18,631 bp in length, containing 13 protein-coding genes, 24 tRNA genes (trnP-UGG and trnT-UGU were duplicated), 2 rRNA genes, and non-coding control regions (). Notably, the mitogenome contained a large repeat structure (788 bp) at positions 9,631 to 10,418 and 11,417 to 12,204, and two duplicated tRNA genes were part of this repeat structure (Supplementary Figure S3). The tRNA analysis indicated the trnP-UGG and trnT-UGU genes were identified as functional genes, not pseudogenes. In the case of two low coverage depth regions (around 8,333 bp and 10,775 bp) highlighted by red arrows in Supplementary Figure S2, it was confirmed by Sanger sequencing that these regions contained repetitive C sequences, consistent with the NGS assembly results (Supplementary Figures S3 and S4). The composition of this mitogenome is biased toward A + T (53.74%) exceeded that of G + C (46.26%). Within the analyzed set of 13 protein-coding genes, COX1 uniquely initiates with GTG as its start codon, diverging from the typical ATG. Nine of these genes (COX1, COX2, COX3, ND1, ND2, ND3, ND4L, ND4, and CYTB) utilize a TAA stop codon. However, for six of these (COX2, COX3, ND2, ND3, ND4, and CYTB), the stop codon may be extended by an adenine nucleotide during transcription to mRNA, a process facilitated by 3′ polyadenylations (Taanman Citation1999) Furthermore, three genes (ATP6, ATP8, and ND5) conclude with a TAG stop codon, while ND6 employs an AGG stop codon. The phylogenetic tree showed that G. gibberifrons formed an independent lineage as one of the basal branches diverged after P. antarcticum within the family Nototheniidae ().
Figure 2. Circular gene map of the Gobionotothen gibberifrons mitochondrial genome, created and visualized by the MitoFish webserver (Sato et al. Citation2018) and Chloroplot software (Zheng et al. Citation2020), respectively. The inner circle of the image represents the GC% per every 5 bp of the mitogenome, with darker lines indicating higher GC% levels. The arrows inside and outside the innermost circle indicate the transcription direction of genes encoded on the light and heavy strands, displayed inside and outside the outermost circle, respectively.
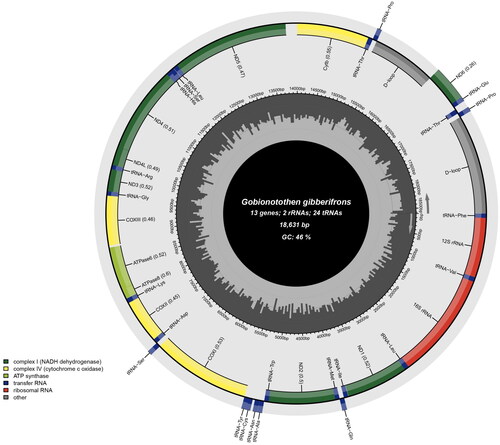
Figure 3. The maximum likelihood phylogenetic tree for Gobionotothen gibberifrons with Gadus morhua as outgroup. The tree was constructed using the amino acid sequences of 13 protein-coding genes. The following GenBank accession numbers of sequences were used: T. lepidorhinus MN864240; T. loennbergii MT447073 (Choi et al. Citation2021); T. nicolai MZ779013 (Patel et al. Citation2022); T. bernacchii MZ779012 (Patel et al. Citation2022); P. borchgrevinki MZ779011 (Patel et al. Citation2022); T. newnesi OP432732 (Nguyen et al. Citation2023); A. mitopteryx MT232658; D. eleginoides JAOVFM010000447; D. mawsoni LC138011; N. coriiceps JF933906 (Oh et al. Citation2016); N. rossii MN893242; P. antarcticum JF933905 (Lee et al. Citation2015); G. morhua X99772 (Johansen and Bakke Citation1996). The species in this study is highlighted by a black dot with bold font.
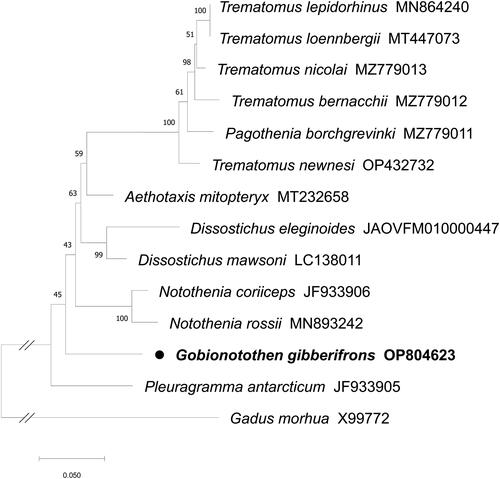
Discussion and conclusion
Through this study, we present the first complete mitogenome of G. gibberifrons, a species belonging to the genus Gobionotothen, for which mitogenome information had not been previously identified. We discovered the presence of two duplicated genes of trnP-UGG and trnT-UGU within the large repeat structure in the G. gibberifrons mitogenome. This duplication has also been reported in other Antarctic fish species such as A. mitopteryx, P. antarcticum, N. angustata, and N. rossii (Zhuang and Cheng Citation2010; Lee et al. Citation2015; Jo et al. Citation2020; Papetti et al. Citation2021; Patel et al. Citation2022), while inferred to have occurred by a tandem duplication and partial random loss (TD-PRL) event between basal non-Antarctic species and the Antarctic common ancestor (Zhuang and Cheng Citation2010; Papetti et al. Citation2021; Patel et al. Citation2022). Until now, there has been controversy for the basal nodes of the Nototheniidae clade (Near et al. Citation2012; Dornburg et al. Citation2017; Near et al. Citation2018; Papetti et al. Citation2021). The phylogenetic tree constructed in this study shows that P. antarcticum and then G. gibberifrons were branched off at the base of the Nototheniidae with a low bootstrap supporting value. The poor resolution is likely due to the absence of intermediary taxa, which has also caused difficulties in understanding the evolutionary pathway in the study of mitochondrial gene order evolution within Antarctic fish (Papetti et al. Citation2021). Therefore, it is imperative to conduct further phylogenetic and evolutionary studies along with diverse mitogenome sequence of Antarctic fishes to comprehend the evolutionary relationship of the genus Gobionotothen within Antarctic fishes. Given the absence of mitogenome research on the genus Gobionotothen, our study will provide valuable information for future Gobionotothen phylogeographical studies.
Authors’ contributions
Conceptualization, JHK, PTN, SL, JK; methodology, PTN, SL, JJ, JK, EJ DWH; resources, DWH, ICK; writing original draft, JK, PTN, SL, JJ; writing review and editing JHK, EJ, SAH, ICK; interpretation of data, PTN, SL, DWH, SAH, ICK, EJ SHK, and JHK; project administration, JHK; funding acquisition, JHK. All authors have read and agreed to the published version of the manuscript.
Ethics statement
All handling and experimental procedures followed the ethical guidelines regulated by the Animal Experimental Ethics Committee established by the Korea Polar Research Institute (KACC2201-008).
Supplemental Material
Download MS Word (632.9 KB)Acknowledgements
We would like to thank PHYZEN Co. for their assistance with software application and analysis. All authors are grateful to the Jeong Il Corporation for supplying the fish sample.
Disclosure statement
The authors declare that no conflict of interests exist.
Data availability statement
The genome sequence data that support the findings of this study are openly available in the GenBank of NCBI at https://www.ncbi.nlm.nih.gov/under the accession no. OP804623. The associated BioProject, SRA, and BioSample numbers are PRJNA881762, SRR22406005, and SAMN31867186, respectively.
Additional information
Funding
References
- Camacho C, Coulouris G, Avagyan V, Ma N, Papadopoulos J, Bealer K, Madden TL. 2009. BLAST+: architecture and applications. BMC Bioinfo. 10(1):421. doi:10.1186/1471-2105-10-421.
- Castresana J. 2000. Selection of conserved blocks from multiple alignments for their use in phylogenetic analysis. Mol Biol Evol. 17(4):540–552. doi:10.1093/oxfordjournals.molbev.a026334.
- Choi E, Im T-E, Lee SJ, Jo E, Kim J, Kim SH, Chi YM, Kim J-H, Park H. 2021. The complete mitochondrial genome of Trematomus loennbergii (Perciformes, Nototheniidae). Mitochondrial DNA B Resour. 6(3):1032–1033. doi:10.1080/23802359.2021.1899070.
- Dereeper A, Guignon V, Blanc G, Audic S, Buffet S, Chevenet F, Dufayard J-F, Guindon S, Lefort V, Lescot M, et al. 2008. Phylogeny.fr: robust phylogenetic analysis for the non-specialist. Nucleic Acids Res. 36(Web Server issue):W465–9. doi:10.1093/nar/gkn180.
- DeWitt H, Heemstra P, Gon O. 1990. Nototheniidae. Fishes of the southern ocean. Grahamstown: JLB Smith Institute of Ichthyology; p. 279–331.
- Dornburg A, Federman S, Lamb AD, Jones CD, Near TJ. 2017. Cradles and museums of Antarctic teleost biodiversity. Nat Ecol Evol. 1(9):1379–1384. doi:10.1038/s41559-017-0239-y.
- Froese R, Pauly D. 2021. FishBase. Version (06/2021). World Wide Web Electronic Publication. Paris, France. www.fishbase.org.
- Gon O, Heemstra PC. 1990. Fishes of the southern ocean. 1st ed. Grahamstown, South Africa: J.L.B. Smith Institute of Ichthyology.
- Jo E, Cho YH, Lee SJ, Choi E, Kim J-H, Chi YM, Kim J-H, Park H. 2020. The complete mitochondrial genome of the Antarctic marbled rockcod, Notothenia rossii (Perciformes, Nototheniidae). Mitochondrial DNA B Resour. 5(3):2421–2422. doi:10.1080/23802359.2020.1775507.
- Johansen S, Bakke I. 1996. The complete mitochondrial DNA sequence of Atlantic cod (Gadus morhua): relevance to taxonomic studies among codfishes. Mol Mar Biol Biotechnol. 5(3):203–214.
- Jones DT, Taylor WR, Thornton JM. 1992. The rapid generation of mutation data matrices from protein sequences. Comput Appl Biosci. 8(3):275–282. doi:10.1093/bioinformatics/8.3.275.
- Larkin MA, Blackshields G, Brown NP, Chenna R, McGettigan PA, McWilliam H, Valentin F, Wallace IM, Wilm A, Lopez R, et al. 2007. Clustal W and Clustal X version 2.0. Bioinformatics. 23(21):2947–2948. doi:10.1093/bioinformatics/btm404.
- Lee J, Lee H, Lee J, Choi J, Park H. 2015. Complete mitochondrial genome of the Antarctic silverfish, Pleuragramma antarcticum (Perciformes, Nototheniidae). Mitochondrial DNA. 26(6):885–886. doi:10.3109/19401736.2013.861443.
- Lowe TM, Chan PP. 2016. tRNAscan-SE on-line: integrating search and context for analysis of transfer RNA genes. Nucleic Acids Res. 44(W1):W54–W57. doi:10.1093/nar/gkw413.
- Matschiner M, Hanel R, Salzburger W. 2009. Gene flow by larval dispersal in the Antarctic notothenioid fish Gobionotothen gibberifrons. Mol Ecol. 18(12):2574–2587. doi:10.1111/j.1365-294X.2009.04220.x.
- Miller RG. 1993. History and atlas of the fishes of the Antarctic Ocean. p. 792.
- Near TJ, Dornburg A, Kuhn KL, Eastman JT, Pennington JN, Patarnello T, Zane L, Fernández DA, Jones CD. 2012. Ancient climate change, antifreeze, and the evolutionary diversification of Antarctic fishes. Proc Natl Acad Sci USA. 109(9):3434–3439. doi:10.1073/pnas.1115169109.
- Near TJ, MacGuigan DJ, Parker E, Struthers CD, Jones CD, Dornburg A. 2018. Phylogenetic analysis of Antarctic notothenioids illuminates the utility of RADseq for resolving Cenozoic adaptive radiations. Mol Phylogenet Evol. 129:268–279. doi:10.1016/j.ympev.2018.09.001.
- Nguyen PT, Lee S, Jeong J, Kim J, Han D-W, Kim I-C, Lee JH, Park J, Kim J-H. 2023. Complete mitochondrial genome of Trematomus newnesi (Perciformes, Nototheniidae). Mitochondrial DNA B Resour. 8(11):1196–1199. doi:10.1080/23802359.2023.2194456.
- Oh JS, Ahn DH, Lee J, Choi J, Chi YM, Park H. 2016. Complete mitochondrial genome of the Antarctic bullhead notothen, Notothenia coriiceps (Perciformes, Nototheniidae). Mitochondrial DNA A DNA Mapp Seq Anal. 27(2):1407–1408. doi:10.3109/19401736.2014.947603.
- Papetti C, Babbucci M, Dettai A, Basso A, Lucassen M, Harms L, Bonillo C, Heindler FM, Patarnello T, Negrisolo E, et al. 2021. Not frozen in the ice: large and dynamic rearrangements in the mitochondrial genomes of the Antarctic fish. Genome Biol Evol. 13(3):evab017. doi:10.1093/gbe/evab017.
- Patel S, Evans CW, Stuckey A, Matzke NJ, Millar CD. 2022. A unique mitochondrial gene block inversion in Antarctic trematomin fishes: a cautionary tale. J Hered. 113(4):414–420. doi:10.1093/jhered/esac028.
- Rutherford K, Parkhill J, Crook J, Horsnell T, Rice P, Rajandream MA, Barrell B. 2000. Artemis: sequence visualization and annotation. Bioinformatics. 16(10):944–945. doi:10.1093/bioinformatics/16.10.944.
- Sambrook J, Fritsch EF, Maniatis T. 1989. Molecular cloning: a laboratory manual. Cold Spring Harbor, NY: Cold Spring Harbor Laboratory Press.
- Sato Y, Miya M, Fukunaga T, Sado T, Iwasaki W. 2018. MitoFish and MiFish pipeline: a mitochondrial genome database of fish with an analysis pipeline for environmental DNA metabarcoding. Mol Biol Evol. 35(6):1553–1555. doi:10.1093/molbev/msy074.
- Taanman J-W. 1999. The mitochondrial genome: structure, transcription, translation and replication. Biochim Biophys Acta. 1410(2):103–123. doi:10.1016/s0005-2728(98)00161-3.
- Tamura K, Stecher G, Kumar S. 2021. MEGA11: molecular evolutionary genetics analysis version 11. Mol Biol Evol. 38(7):3022–3027. doi:10.1093/molbev/msab120.
- Tillich M, Lehwark P, Pellizzer T, Ulbricht-Jones ES, Fischer A, Bock R, Greiner S. 2017. GeSeq – versatile and accurate annotation of organelle genomes. Nucleic Acids Res. 45(W1):W6–W11. doi:10.1093/nar/gkx391.
- Ward RD, Zemlak TS, Innes BH, Last PR, Hebert PDN. 2005. DNA barcoding Australia’s fish species. Philos Trans R Soc Lond B Biol Sci. 360(1462):1847–1857. doi:10.1098/rstb.2005.1716.
- Zheng S, Poczai P, Hyvönen J, Tang J, Amiryousefi A. 2020. Chloroplot: an online program for the versatile plotting of organelle genomes. Front Genet. 11:576124. doi:10.3389/fgene.2020.576124.
- Zhuang X, Cheng C-HC. 2010. ND6 gene “lost” and found: evolution of mitochondrial gene rearrangement in Antarctic notothenioids. Mol Biol Evol. 27(6):1391–1403. doi:10.1093/molbev/msq026.