Abstract
The study addressed the ecology and life cycle of two charophyte species, which are both at risk of extinction and on several Red Lists in Europe. Populations of Nitella opaca and N. gracilis, sometimes occurring together in three wetland ponds, were monitored for several years, and environmental data were also recorded. The pattern and timing of emergence (new shoots) and maturation of the sexual organs (antheridia, oogonia, and ripe oospores) were determined on plant samples collected regularly. The species phenophases were related to the environmental data. The results showed that species distribution can be linked to the water calcium content, a parameter that most differed in the three ponds. N. gracilis developed population only in ponds with very low calcium concentrations, whereas N. opaca had a wider tolerance. Following a pond bed air exposure in previous year, dense populations of N. opaca and N. gracilis were observed. None, or only a few individuals, were found when the waterbodies remained flooded the whole preceding year. Light and temperature affect differently the emergence of sex organs and the maturation of oospores. Both species differed in the timing of their phenophases. N. opaca appeared in March, reproduced and disappeared in July. N. gracilis was generally observed later in spring, and after full reproduction, the plants started a second cycle in late summer, while new oospore germination occurred. N. gracilis was perennial and reproduced all year long. Taking these results into account will help to design conservation plans for N. opaca and N. gracilis.
Introduction
Aquatic macrophytes have key functions in biochemical cycles (Kleeberg et al. Citation2010), which make them essential for supporting the healthy ecological status of aquatic ecosystems (Bornette and Puijalon Citation2009). Therefore, it is important to preserve their communities. Among aquatic macrophytes, charophytes are benthic macroalgae closely related to modern land plants (Karol et al. Citation2001) that can be found in fresh to brackish waters in permanent or temporary ecosystems.
Besides key roles in biochemical cycles, charophytes provide a multitude of ecosystem services, which include water purification, food for aquatic animals as well as farm stock, fertilizers, polishes, mud baths, therapeutic applications, sugar purification, fish culture and insect control (Schneider et al. Citation2015). On the other hand, charophytes are sensitive to environmental changes, such as eutrophication (Krause Citation1981; Blindow Citation1992). They are already affected by moderate eutrophication in many locations and are therefore often used as bio-indicators for oligotrophic to mesotrophic waters. Beside eutrophication, climate change might impact on charophyte reproduction, affecting in turn their geographical distribution and the survival of some species (Auderset Joye and Rey-Boissezon Citation2015).
Distribution analyses in Europe have shown that several charophyte species have disappeared, and many have declined over time (Simons and Nat Citation1996; Auderset Joye, Castella, and Lachavanne Citation2002; Blaženčič et al. Citation2006a; Baastrup-Spohr et al. Citation2013). These observations have resulted in the evaluation of species extinction risk, reported in Red Lists (Stewart and Church Citation1992, Blaženčič et al. Citation2006b; Auderset Joye and Schwarzer Citation2012; Korsch et al. Citation2013; Fredriksen et al. Citation2015; ArtDatabanken Citation2015). All lists registered a high proportion of species with a more or less severe risk. To conserve the endangered charophytes, we need to improve our knowledge of their ecology. Charophytes are often pioneer plants in newly created or inundated wetlands, and may form perennial beds in permanent lakes (Hutchinson Citation1975). However, many of the most endangered species are colonizing wetlands and small ponds. Under the current temperature increase, small and shallow ecosystems are subject to a higher unpredictability and frequency of pond bed air exposure, making sexual reproduction more important for charophyte populations. Their survival in such unpredictable habitats depends, in part, on the timing and pattern of oospores germination, when the seed bank is inundated (Casanova and Brock Citation1990). Indeed, the timing of reproduction critically defines plant reproductive success and species dynamics, affecting dispersal and colonization rates and the geographic distribution of plants (Chuine and Beaubien Citation2001). Nevertheless, in the literature, the relationships between phenology and species range attributes are under-explored (Morellato et al. Citation2016). This is especially true for plants living in freshwater ecosystems. Among environmental factors, water quality, solar radiation and temperature affect phenology by directly influencing biochemical processes and the developmental rates of the organisms (Franklin Citation2009).
In this study, we monitored the phenology of N. gracilis (monoecious) and N. opaca (dioecious) in three neighbouring wetland ponds for a medium-long-term period (2007 to 2016). Both species are cosmopolitan and quite threatened in Switzerland and in Europe. The opportunity of finding two rare species present in a set of adjacent ecosystems enhanced the analytical power of the data. All three ponds experienced similar temperatures and light conditions, but contrasting water regimes.
The aim of this study was to answer the following questions: (i) Why did N. opaca and N. gracilis colonize different ponds located in the same wetland? (ii) What impact does the pond bed air exposure have on Nitella populations? (iii) Do both species show the same life cycle? (iv) Within their reproductive cycles, which light and heat conditions support their development from one phenophase to the next? Summarizing, we tried to understand how water chemistry, hydrology, light and a climatic factor associating time with temperature (growing degree day) affect the reproduction phenology of the two freshwater Nitella species.
Materials and methods
Study sites
Prés-de-Villette is a 22 ha wetland located in the south-western part of Switzerland at 470 m altitude (canton Geneva 46°15′14.481″N 6°17′14.559″E). The climate of Geneva is heavily influenced by the Atlantic Ocean. The mild humid sea breeze that reaches the country has a cooling effect during summer and a warming effect during winter (MétéoSuisse Citation2015). For the period 1981–2010, the typical annual mean temperature was around 11°C with 1800 h sun and a total of 1000 mm rain (MeteoSuisse Citation2017). Prés-de-Villette is a protected area of national importance situated on an impermeable layer of clay with glacial origin (Fig. ). Several ponds were created inside the wetland, which is surrounded by woods. The origin of the water is rain and soil leaching. The wetland is dominated by communities of Caricion gracilis (Phragmito-Magnocaricetea, Prunier et al. Citation2014) and the three ponds harbour charophytes. Pond Vaucher (VAU) was created in the 1960s and cured in 1999. Pond with an island (ILE) was dug at the end of 1999 and the third pond (OUT) in autumn 2011. Water flows from the NE part to the SW part of the wetland. A dam was constructed in 2011 and since then ponds ILE and OUT, connected together, have remained flooded. Located behind the dam, VAU is not connected to the two other ponds and has dried out regularly. All ponds are shallow (maximum depth <1 m).
Figure 1. Location of wetland Prés-de-Villette in Switzerland, and detail map of ponds Prés-de-Villette VAU: pond Vaucher (46°15′13.706″N 6°17′06.906″E), ILE: pond with an island (46°15′12.922″N 6°17′18.140″E), OUT: pond in front of the dam (46°15′14.584″N 6°17′12.794″E) (source: https://ge.ch/carte).
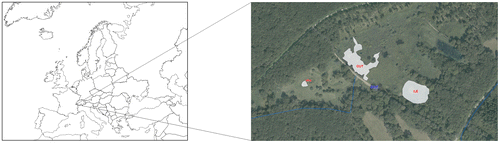
Pond VAU is colonized by Nitella opaca and a few Chara globularis plants, which formed patches scattered between the vascular plants (Ranunculus trichophyllus, R. circinatus, R. flammula, Schoenoplectus tabernaemontani, Carex elata and Iris pseudacorus).
In pond ILE, N. opaca, N. gracilis, Chara virgata, Potamogeton berchtoldii, and Utricularia australis are developing into small patches, growing below the emergent plants, mainly Eleocharis palustris aggr. and a few Typha latifolia.
In pond OUT, the abundance of N. opaca varied from year to year, sometimes forming dense patches covering a large area. N. gracilis was found in one location within N. opaca stands, accompanied by few patches of Potamogeton berchtoldii, Myriophyllum spicatum, M. verticillatum, Callitriche sp. and Hydrocharis morsus-ranae. Charophytes were identified following Krause (Citation1997) and vascular plants following Lauber, Wagner, and Gigax (Citation2012).
Period of monitoring
The period of monitoring depended on the circumstances. Charophyte species were surveyed from spring 2007 to spring 2016. Physical and chemical water variables were measured from 2007 to 2015. Finally, probes with a data logger recording water and air pressure, as well as temperature, were installed in 2009 and removed in 2014.
Water chemistry
Physical and chemical variables were measured in situ once a week or fortnight, using portable field equipment for oxygen (Hach LDO1 Meter), turbidity (Hach 2100Qis) and conductivity (WTW m3210). Water samples were collected on several occasions between 2007 and 2015. Total phosphorus (μg/l) was measured after a persulphate digestion, according to the ascorbic method (Clesceri, Greenberg, and Eaton Citation1999). Nitrate, sulphate, chloride, calcium and magnesium concentrations (mg/l) were measured by ionic chromatography (Dionex ICS-3000).
In situ temperature and water-level recording
A permanent data logger (HOBO; onsetcomp.com) recording water temperature and pressure every 4 h was installed in the deepest parts of each of the three ponds. In situ data were collected in ponds VAU and ILE from September 2009 to November 2014 and in pond OUT from September 2013 to November 2015. Water pressure was corrected for atmospheric pressure (recorded by a logger fixed in a tree) and transformed into water depth by the barometric compensation assistant of HOBO software (https://www.onsetcomp.com). Data collected by the loggers allowed the calculation of water-level fluctuations in VAU and ILE for almost six years.
Calculations related to water temperature
To describe and compare the species’ phenology in the three ponds, we calculated the water mean weekly temperature (WMT). Growing degree days (GDDs) is a measure of heat accumulated over time. GDDs are used for agricultural applications, such as estimating harvest time or pest growth, and provide a more accurate physiological estimate than counting calendar days (Lancashire et al. Citation1991). GDDs were first applied to charophytes by Boissezon (Citation2014). GDD parameters were calculated with the actual temperature method (no cutoff) from the HOBOware® Pro GDD Assistant (Onset Computer Corporation, Bourne, MA, USA). This method performs a numerical integration and uses the sampling interval of temperature data (4 h in this case) to compute the area between the daily temperature data and the low threshold. There is no well-defined low-temperature threshold for the growth of charophytes (Boissezon Citation2014), but many charophytes tolerate water temperature down to at least 4°C, the minimum temperature recorded in temperate ponds. At this temperature, water has the highest density and sinks to the bottom of the pond, causing the less dense ice forming on water surface, with the rest of the pool at 4°C. Further, we used 4°C as the low-temperature threshold to allow for comparisons with previous studies (Boissezon Citation2014; Calero et al. Citation2017). The first day of January of each year was set as the starting date for GDD calculation (biofix).
Light
We attempted to collect relative light level data in situ with an underwater sensor provided by a data logger. However, we did not get reliable light measurements, because the captor cell needed to be cleaned more frequently than was possible. Instead, to test for the specific effect of light on the emergence and production of oospores, we related phenophases to the mean day length (DL) during the week of the biological observations (Monday to Sunday, https://dateandtime.info/fr/citysunrisesunset.php?id=2660646).
Charophyte sampling, observation and analysis
To analyse the life cycle of both Nitella species, charophyte samples were collected by hand, or using a hook, every week or fortnight in spring and summer and once a month in autumn and winter, for the accurate detection of reproductive events. After washing the fresh material to remove sediment and organic matter, charophytes were kept in the refrigerator or fixed in 70% ethanol for further observation. Between 10 and 20 specimens were observed on each sampling date (less frequently when charophytes were sterile) with a stereomicroscope (Leica Camera M205C maximum magnifications of 160×). The whole thallus, including side whorls, was observed to monitor the emergence and maturation of sexual organs (called gametangia in algae).
Every plant’s phenological stage was identified at each date of collection (Table ): presence of species recorded as oospores in the sediment only (phase 99), sterile shoots (phase 0), emergence of the first antheridia (male gametangia, phase 1). The unripe antheridia change colour from yellow (N. gracilis) to light orange (N. opaca), whereas the ripe antheridia turn dark orange with marked shield cells that open out to release spermatozoids when maturation is complete. The next step is the emergence of the first oogonia (female gametangia, phase 3). At this phase, at least some antheridia are ripe (on the same shoots in N. gracilis or on different shoots in N. opaca). In both species, oogonia change colour to green or light orange and are considered to be ripe when swollen. After that phase, oogonia either become abortive or are fertilized, forming the oosporangium. Inside this organ, oospores (the products of fertilization of gametes) start ripening. The first ripe oospores (phase 4) and many ripe oospores (>10%, phase 5) were recorded as the oospores cell wall (made of resistant organic matter) became russet to dark brown (N. opaca) or grey-brown (N. gracilis). At the end of the reproductive cycle, N. opaca declined and decomposed (phase 7), whereas N. gracilis started a second reproductive cycle (phase 6). In some cases, N. gracilis was declining (phase 7), while new oospores germination occurred (phase 8).
Table 1. Phenophases observed in the dioecious N. opaca and monoecious N. gracilis between 2007 and 2016.
Analyses
In total, 221 observations of N. opaca and 132 of N. gracilis were recorded and used for analyses. Relations between phenophases and environmental variables were analysed using the boxplot function in R software (R Development Core Team Citation2016) as proposed by Tukey (Citation1977). Data collected from the three ponds were analysed together.
Results
Water chemistry
Ponds in Prés-de-Villette have mesotrophic waters (OCDE Citation1982), as indicated by the total phosphorus and nitrate concentrations (Table ). The conditions of oxygenation fluctuated in time, varying from almost anoxic (5%) to hyper-saturated (256%), but no stratification occurred in the water column. In all three ponds, the water was moderately mineralized (conductivity < 200 μS.cm−1), mainly because it originated from precipitation and drainage of clay soil. Magnesium, chlorides and sulphates were found at low concentrations in all three ponds. Calcium and magnesium concentrations were quite weak, although ILE had a mean concentration (<15 mg/l) less than a half that of VAU (>38 mg/l).
Table 2. Synthesis of the physical and chemical measurements in Prés-de-Villette ponds VAU, ILE and OUT (all data). Legend: Min: minimum; Mean: mean; Max: maximum. N: number of measures. Cond25: conductivity, reference 25°C [μS/cm]; temp: temperature [°C]; O2: oxygen [mg/l]; O2%: oxygen saturation; turbidity [NTU];
: ammonia nitrogen [mg N/l];
N-NO3–: Nitrates [mg N/l], P-Ptot: total phosphorus [μg P/l]; Cl–: chlorides [mg/l];
: sulphates [mg/l]; Ca2+: calcium [mg/l]; Mg2+: magnesium [mg/l].
Hydrology
VAU dried out every summer, and the duration of pond bed air exposure varied from several months (2009, 2010 and 2011) to only a few weeks (2014) (Fig. ). During our survey, pond ILE dried out only in 2009 and 2015 (observed, not measured). The construction of a dam in 2011 (Fig. ) modified the hydrological regime of pond ILE, which has remained permanently flooded since then (2012 to 2015). Pond OUT’s water level followed the same pattern as ILE, to which it is connected. In 2015, during a very hot summer, all three ponds under study were completely dehydrated from June to autumn.
Temperatures
All three ponds exhibited a similar pattern of temperature (Fig. ). During the period of observation, water temperature varied to a greater extent over the year than from pond to pond. For example, the water exceeded 25°C in summer 2009 more frequently than in the following years. The coldest year of the series was 2014, as summer temperatures stayed below 25°C. Ponds were totally covered with ice for a short period in winter. All three ponds experienced a mean temperature of 13–14°C in spring (March to May), 21–22°C in summer (June to August), 12–13°C in autumn (September to November) and 5°C in winter (December to February).
Occurrence of Nitella opaca and Nitella gracilis between 2007 and 2016
The abundance of both species differed between ponds and from year to year (Table ). In pond VAU, N. opaca was found every year from 2007 to 2016, except in 2011. In pond ILE, N. opaca was observed sporadically: plants germinated and established themselves in small patches in 2007, 2008 and 2010. No plants were observed from 2011 to 2015, despite a close monitoring of the species, but occurred again in 2016. N. opaca also colonized pond OUT and appeared the second year after the digging of the pond. The population was most abundant and widespread in 2014. In 2015, only a few patches were present, but plants formed dense mats again in 2016.
Table 3. Abundance of Nitella gracilis and Nitella opaca in Prés-de-Villette ponds.
N. gracilis colonized ponds ILE and OUT exclusively, and has never been observed in VAU. During the survey, the species was quite abundant in pond ILE in 2007, 2010 and 2011. A decline of the population was observed in 2012, and only a few shoots were recorded in 2013 and 2014. In 2016, the species germinated and established, forming a large number of small patches. In pond OUT, N. gracilis appeared the second year after the digging (2013), while in 2014 only a few shoots were observed. Since then, no shoots were observed in pond OUT.
Species life cycle
The number of phenophases, represented by a boxplot, differ between the two species (Figure A and B). No phases 6 and 8 are shown for N. opaca, as no second reproduction was observed, and no new germinations were observed at the time of plant senescence. Besides, some phenophases were observed more rarely than others, because they lasted a shorter time than the interval between two successive observations. For example, N. gracilis emergence of the first oogonia and plant senescence were observed only once. Therefore, the boxplot shows no variation and is represented by a single bar only (phases 2 and 7; see Table ). Nitella opaca and N. gracilis exhibited different life cycles with reproductive phenophases occurring under different light and temperature conditions within the ponds of Prés-de-Villette (Fig. ).
Figure 4. (A) Nitella opaca, (B) Nitella gracilis. Phenophases recorded relative to the week of the year (A1, B1), water temperature (WMT) (A2, B2), growing degree day (GDD) (A3, B3), day length (A4, B4), and water level (A5, B5). Legend of phenophases: (0) sterile plants, (1) first antheridia, (2) first oogonia, (3) ripe antheridia and mature oogonia, (4) first ripe oospore, (5)>10% ripe oospores, (6) plants with ripe oospores starting new reproductive cycle (N. gracilis only), (7) plants senescent, (8) plants senescent and new germinations (N. gracilis only), (9) oospores in sediment. (See Table for more details.) Distribution based on 221 (N. opaca) and 132 observations (N. gracilis). The box is defined by the lower and upper quartiles. The median is indicated by the bold line. The whiskers indicate the minimum and maximum, except the outliers. The outliers are defined as the data outside 1.5× the interquartile range, computed from the upper and lower quartiles respectively.
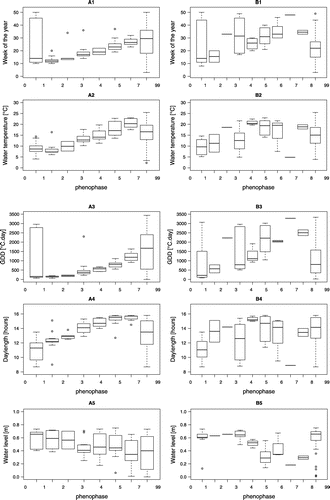
Nitella opaca
In spring, the first sterile shoots of N. opaca (phase 0) were observed in March (weeks 10–12), when the daylight period (DL) was about 12 h, the water temperature was 7–8°C (WMT), and the water had accumulated about 120 degree days (GDDs) since the beginning of the year. Antheridia (phase 1) started to appear 1–2 weeks after the shoots (DL around 12 h, WMT 7–8°C, 130 GDDs). In the beginning, a few male plants were present in a sterile cluster of plants; their number then increased to about 50% of the shoots, and then the other 50% developed into female plants. First oogonia (phase 2) developed about one week after the first antheridia (week 12, DL about 13 h, WMT 10°C, 200 GDDs). The first antheridia matured (phase 3) and released spermatozoids progressively, and during this phase oogonia developed (week 19, WMT 13°C, DL 14 h, 350 GDDs).
The first ripe oospores (phase 4) were detected from the end of April to the beginning of June (weeks 17–23, at DL about 15 h, WMT 13–14°C, 500 GDDs). The oospores matured in June (phase 5, week 22, WMT 18°C, DL > 15 h, around 800 GDDs) and remained on the plants. Each fertile whorl produced six to 18 oospores (mean 12). When the water temperature exceeded 20°C, the plants often disconnected from the sediment, but the oospores continued to ripen on the floating plants that finally decomposed (phase 7). During some summers, new male shoots appeared and ripened until the plants finally become senescent and disappeared completely (phase 7). A few shoots that originated from new germinations (phase 0) have also been occasionally observed in autumn (2013–2014) and could survive until the next season. These marginal events have been noted only a few times during the years of observations. With the progression of the season, the size of the plants increased and reached 40 cm, occasionally up to 70 cm. Oospores were dark brown and subspheric, with a diameter of 320–360 μm.
In summary, Nitella opaca was fertile from March to July (phase 1 to 5) and was present as a seed bank (phase 99) from August to March of the next year in the three ponds of Prés-de-Villette.
Nitella gracilis
In spring, the first sterile shoots of N. gracilis developed from oospores were observed in March to April (phase 0, week 11–15, DL 12–13 h, WMT 10–14°C, 250 GDDs). Plants bearing very young antheridia (phase 1) were recorded soon after the sterile plants appeared, as the DL exceeded 12 h (WMT around 12°C and 500 GDDs). This monoecious species displayed both antheridia and oogonia on the same shoots very soon after the onset of antheridia (phase 2, WMT around 12°C and 600 GDDs). Antheridia ripened and released spermatozoids, and oogonia matured during April to May (phase 3, week 18–21, DL 14–15 h, WMT 16°C and 660 GDDs). The first ripe oospores were observed from mid-May to mid-July (phase 4, weeks 20–30, DL > 15 h, WMT around 20–21°C and 1300 GDDs). Each whorl generally produced six oospores, which detached from the plants as soon as they were ripe. More than 10% of oospores, formed in the spring, were ripe between the end of May and the end of July (light > 15 h a day, WMT over 20–22°C, 1400 GDDs). As soon as they are ripe, oospores detached from the plants and fell on the pond bed.
A second reproductive cycle started at the end of July: plants, developed from spring germination, started new lateral branches bearing young antheridia and oogonia (phase 6, DL 15 h, WMT 20°C, 2000 GDDs, water around 30 cm depth). In the middle of August to October, plants from the spring continued their cycle (phenophases 1 to 5 coexisted). In the meantime, new oospore germination occurred (phase 8, water level 30 cm), and some shoots declined (phase 7). From December to March, the plants either were sterile or bore immature gametangia (formed in autumn) and survived the winter, even when the ponds were frozen. The next spring, plants continued their life cycle started in the preceding year. The size of the plants reached 10 cm, occasionally 15 cm. Oospores were light brown, and they were typically 300 μm in length and 250 μm in width.
In summary, N. gracilis was a perennial species, forming oogonia and antheridia from spring to winter. Oospores germinated twice a year: in the spring and in the autumn. However, plants disappeared totally after 2–3 years’ growth in the ponds of Prés-de-Villette.
Discussion
What roles are played by water chemistry, hydrology and climate variables in the establishment of N. gracilis and N. opaca, and the development of their reproductive organs?
Species and water chemistry
The calcium content of the water probably explained the distribution of both species in the ponds studied. Nitella gracilis was found in pond ILE (3.5–21 mg/l Ca2+) and pond OUT but never in pond VAU (27–43 mg/l Ca2+). It has colonized pond ILE regularly since 2007 but failed to establish in pond OUT after 2013–2014. Other localities, where N. gracilis was recorded in Switzerland, had very low calcium concentrations from 4.5 to 6.0 mg/l Ca2+ (Rey-Boissezon and Auderset Joye Citation2015). In Germany, N. gracilis is also known as a species recorded in water containing 15 to a maximum of 30 mg/l Ca2+ (Doege et al. Citation2016). The calcium content in pond VAU is above this tolerance threshold, probably explaining the absence of the species. Unlike N. gracilis, N. opaca was abundant in pond VAU, in which water calcium content exceeded the minimum required for this species (17.6 mg/l Ca2+), according to Doege et al. (Citation2016). In pond ILE, where N. opaca was less abundant, the mean calcium content was only 14.5 mg/l, a value close to the minimum required for this species. The other parameters measured in ponds VAU and ILE were quite similar, and according to the nutriments concentration, they can be considered mesotrophic (OCDE Citation1982).
Species and water-level decrease
Water-level changes affect the reproduction of both Nitella species. We observed the decline of N. opaca (phase 7) when the water decreased under a depth of 40 cm. This decay, however, happened when most oospores were ripe, so the decrease did not threaten the plant reproductive success. On the other hand, when the water level decreased to a depth of 20–40 cm, N. gracilis showed accelerated oospores maturation and late summer new oospore germination (Fig. B5). Low water level exposed N. gracilis to intensified light, and these conditions seemed to favour its reproduction.
Timing of the emergence and maturation of sexual organs of C. hispida was reported to be dependent on the water depth (Calero, Colom, and Rodrigo Citation2015), and this is probably the case for some other species, too. In laboratory experiments, Casanova (Citation1994) compared the effect of water-level changes on the perennial Chara australis and the annual Nitella sonderi. Decreased water level triggered the sexual maturation of plants of C. australis (a dioecious perennial), whereas the plants in water depths over 54 cm remained sterile. In contrast, the annual N. sonderi reproduced under all water-level conditions. Casanova and Brock (Citation1999) also showed that the monoecious annual C. muelleri invested a greater proportion of its biomass in sexual reproduction under a slowly falling water level than in rising water. In addition, a rapid decrease in the water-level accelerated the maturation of oospores, which appeared up to 4 weeks earlier than in the flooded treatment. These responses to water-level drawdown should ensure that sexual propagules are produced before the habitat dries out completely, whereas greater resource allocation to shoot elongation and reproduction is deferred when the water depth increases (Casanova Citation1994).
Species and drying out
Besides water-level changes, a pond totally drying out and the timing of this event affected the reproduction of both Nitella species. All the ponds studied generally had their maximal water levels from winter to mid-summer. This period was long enough for N. opaca and N. gracilis to achieve their reproductive cycle. Every year, N. opaca germinated in early spring, established, produced a large number of oospores and achieved its life cycle before total desiccation of pond VAU, except in 2011, when the pond had already dried up in April.
Like N. opaca, N. gracilis germinated in spring, established and started a first reproductive cycle in spring. In summer, when the water level dropped, N. gracilis initiated a second reproductive cycle by forming new fertile shoots on the same plants. In years when the pond ILE dried out during summer, N. gracilis produced new germlings in autumn after re-inundation, reproduced until winter and potentially continued its cycle the next spring. By contrast, in permanently flooded conditions N. gracilis did not reappear the second year after the last drying out of the sediment.
During our survey, every year following a drying out of the ponds, both N. opaca and N. gracilis germinated and developed dense and fertile populations. This was evident almost every year in pond VAU (colonized by N. opaca), as well as in pond OUT, since the second year after its creation. In the same way, the number of shoots issued from N. gracilis oospores was particularly abundant in pond ILE one year after the pond bed air exposures (2010 and 2016). On the other hand, when the ponds remained flooded the whole year, only a few sparse individuals germinated and established the following year. Enhanced oospore germination following a dewatering of the ponds indicated that pond bed air exposure plays an important role in the new plants of N. opaca and N. gracilis emerging from the seed bank.
Numerous studies have demonstrated the critical role of flooding and pond bed air exposure in the development of plant communities, germinating from soil seed banks of variable wetland habitats (Seabloom, van der Valk, and Moloney Citation1998; Casanova and Brock Citation2000; Crosslé and Brock Citation2002; Nicol, Ganf, and Pelton Citation2003; Warwick and Brock Citation2003; Coops, Beklioglu, and Crisman Citation2003). An enhanced development of charophytes after drying out of the sediment was also reported by several authors. Germination experiments performed by Casanova and Brock (Citation1996) on the Australian charophyte species showed that drying the seed bank enhanced the germination of Nitella cristata var. ambigua, N. sonderi and N. subtilissima. Harwell and Havens (Citation2003) examined the potential for recovery of submerged vegetation from a desiccated and re-inundated seed bank in a tropical lake. They observed a faster and more extensive emergence of Chara ssp. oospores and suggested that the drying out of the sediments may have led to an increased germination response in Chara. Moreover, many Mediterranean waterbodies that harbour a large number of charophyte species are temporary habitats characterized by an annual sequence of inundation and pond bed air exposure (Grillas Citation1990; Soulié-Märsche Citation1998; Rodrigo, Alonso-Guillén, and Soulié-Märsche Citation2010). In Mediterranean temporary pools, the time of flooding after a pool bed air exposure was shown to impact the composition of the vegetation. Early flooding (September) favours the establishment of species-rich communities, whereas late flooding (March) results in a reduction in the number of species and in dominance by opportunist species (Gauthier and Grillas Citation2004). Moreover, enhanced oospore germination after a pond bed air exposure seems to be species-specific. For example, Rodrigo, Alonso-Guillén, and Soulié-Märsche (Citation2010) showed that the oospores of Chara hispida, which germinated and developed germlings, originated from sediment that had not been exposed to air. A long-term study on the occurrence and distribution of Nitellopsis obtusa supported the hypothesis that germination and establishment of dense meadows occurred after several years of flooded conditions (Boissezon Citation2014; Boissezon, Auderset Joye and Garcia Citation2017 this issue).
Species, temperature and associated GDDs
In spring, the onset of first sprouts varied between years, depending on seasonal temperatures and the heat the water had accumulated (GDDs) from the beginning of the year. Nitella opaca started to reproduce at a colder temperature than N. gracilis and needed less heat energy (GDD). For example, N. opaca’s first oospores (phase 4) were ripe when the WMT reached 13–14°C (500–600 GDDs) and those of N. gracilis around 20°C (1000–1200 GDDs). Therefore, to achieve this phase, N. gracilis needed twice the amount of heat accumulated by N. opaca. When N. gracilis oospores (300 μm long, 250 μm wide) began to ripen, all those of N. opaca were already ripe (diameter 320–360 μm).
The entire life cycle of N. opaca required about 1200 GDDs, whereas to fill its first reproductive cycle, N. gracilis needed 2000 GDDs. To achieve a second reproductive cycle, starting on the same plants at the end of the summer, only 1000 GDDs were needed. This finding suggests that beside accumulated heat, light is also required for the maturation of reproductive organs. Indeed, if only temperature was required, 2000 GDDs would also have been necessary for N. gracilis to achieve its second cycle.
Plants of N. opaca, but not N. gracilis, decomposed when the temperature exceeded 20°C. Very similar results have been published by Soulié-Märsche (Citation2004), who described fructification of N. opaca between 12 and 18°C, and decay of the plants when the temperature exceeded 20°C. N. opaca is characterized as a vernal species in the Mediterranean area, as well as in Switzerland.
Our results suggest that temperature affected N. opaca and N. gracilis oospore germination and additionally, later in their life cycle, oospore maturation. In addition, antheridia and oospores of both species, collected in late spring in the ponds Prés-de-Villette and kept at room temperature (20–22°C) under ambient light conditions, ripened more rapidly than those of plants living under the natural conditions (10–12°C). However, N. opaca and N. gracilis collected sterile and kept under the same room conditions as above struggled to grow antheridia and oogonia, suggesting that light is an important driver in the first phenophases of their sexual reproduction.
Several authors have reported that temperature can affect oospore germination (Casanova Citation1994; Casanova and Brock Citation1996), and the rate at which oospores germinate can be related to the degree days they have experienced (Boissezon Citation2014). The relationship between the charophyte germination and temperature has been demonstrated experimentally by Bonis, Lepart, and Laloé (Citation1996). Germination experiments performed on several Mediterranean macrophyte species at different temperatures showed that the rate of seedling emergence is modified significantly only for charophytes, with a strong decrease in cold conditions. Moreover, each species has its own germination pattern, and the next phenophase of reproduction might depend on temperature, as well as on light. Calero et al.’s (Citation2017) results also suggest that temperature (besides radiation) is one of the most important drivers of charophyte reproductive phenology, and accumulated heat (GDDs) is a better predictor than temperature alone on phenological events in organisms, such as submerged macrophytes.
Species and light
According to De Winton, Casanova, and Clayton (Citation2004), experimental evidence shows that although germination can take place in darkness, light is required for establishment of young thallus. This seems also the case for the emergence and maturation of sex organs, at least for the species studied here. While the ponds studied experienced similar temperatures, N. opaca and N. gracilis appeared and matured their sexual organs under different light conditions. N. opaca antheridia appeared in the spring when the day was 12 h long and N. gracilis when it lasted almost 14 h. Oogonia appeared soon after antheridia at similar DL. Both species matured their oospores during the longest days (16 h).
In August, N. gracilis started a second reproduction cycle when the day lasted 15 h. Oospores, developed from this late reproductive cycle, matured in the autumn when the DL declined (12 to 9 h). This suggests that a higher amount of light is needed to produce antheridia and oogonia than to ripen oospores. However, as oospore maturation took place late in the season, the irradiance and the quantity of light they have received, since the beginning of their growth, might be at least as important as photoperiod (DL). It is difficult to separate these two effects based on our observations. Yet, according to an experiment on Chara vulgaris, Wang, Yu, and Xiao (Citation2008) have shown that both irradiance and photoperiod had positive effects on the emergence of sex organs (male and female) and the maturation of oospores, except that increased photoperiod did not accelerate the maturation of oospores. Wang concluded that compared with irradiance, photoperiod seemed to be a less important factor affecting growth and reproduction of plants. Laboratory experiments would be necessary to deconstruct the impact of irradiance and photoperiod on the different reproductive phenophases of both Nitella.
Conclusions
This study is a medium-long survey dealing with charophyte reproductive phenology, the longest one published up to now. In the wetland studied, N. opaca and N. gracilis colonized distinct ponds, and their distribution differed according to the calcium content of the water. N. gracilis colonized water with a very low calcium content, while N. opaca tolerated water richer in calcium.
The air exposure of the pond bed had significant impact on N. opaca and N. gracilis populations. After air exposure, enhanced oospore germination from the seed bank of the sediment was evidenced for both species. This phenomenon happened regularly during our monitoring.
Nitella opaca and N. gracilis showed a different timing in their phenology. Under the local conditions, N. opaca was an annual plant, achieved sexual reproductive cycle from spring to summer and deposited a large number of ripe oospores in the sediment. Nitella gracilis was a perennial plant, reproduced throughout the whole year and produced a large number of oospores as well. For many charophyte species, the dispersion, colonization and maintenance of populations depend entirely on the oospore bank (Bonis and Grillas Citation2002). In our ponds, which experienced irregular flooding and air exposure of the pond bed, the survival of N. opaca and N. gracilis depends entirely on the production of resistant dormant propagules.
Temperature as well as light conditions turned out to be important for the sexual reproduction of both species. Temperature appeared to be more important for oospore germination and maturation, whereas light seemed to have a major effect on the emergence and ripening of sexual organs.
Our results are in accordance with those of Blindow (Citation1992), who found that light, climate and disturbance were more important determinants of charophyte abundance than phosphorus levels. In this study, pond disturbances such as air exposure and flooding of the pond bed showed a prevalent effect, stronger than light and climate in triggering germination of N. opaca and N. gracilis oospores. For their part, temperature and light have predominant effects on growth and sexual reproduction.
Ponds can be one of the easiest and most rewarding semi-natural habitats to recreate, and by applying simple principles to their design and location, most ponds can quickly develop into useful wildlife habitats (Williams et al. Citation1997). Our results show that environmental conditions favourable for the establishment and reproduction of N. opaca and N. gracilis can be easily replicated, when creating new ponds and defining management plans involving charophytes. For example, taking into account hydrology, especially water quality and water level could help to promote populations of Nitella opaca and N. gracilis, and probably other charophyte species.
Notes on contributors
Dominique Auderset Joye is an aquatic ecologist and researcher at the University of Geneva. For the last 20 years, she has been working on the distribution and ecology of macrophytes from freshwater ecosystems. Principal author of the Swiss Red List of Charophytes, she was involved with action planning to reverse the extinction risk of threatened populations.
Contribution: planned research work, collected and analysed the data, and wrote the manuscript.
Aurélie Boissezon is an ecologist and researcher at the University Geneva. Her thesis focused on the impact of environmental factors on the distribution and dynamics of charophyte communities. She is currently working as a botanist involved in both terrestrial and aquatic plant ecology.
Contribution: planned research work, collected data and reviewed the manuscript.
Disclosure statement
No potential conflict of interest was reported by the authors.
Acknowledgements
We are grateful to DGNP who partially financed the study. We acknowledge all our colleagues from F.-A. Forel Institute who have assisted during this long-term study: Philippe Arpagaus, Daniel Palomino and Serge Stoll for providing laboratory assistance. All our acknowledgements are also addressed to our colleagues, students and close relatives for efficient help on fieldwork: Camille Augier, Olga Béguin, Tamara Garcia, David McCrae, Hélène Mayor-Siméant, Gaëtan Rey and Dominique Joye. Many thanks to the reviewers for their careful reading and very valuable comments on the manuscript. We are also very grateful to Mary Beilby, who improved the English language, ensuring effective communication of the written concept.
References
- ArtDatabanken. 2015. Rödlistade arter i Sverige 2015. [The 2015 Red List of Swedish Species]. Uppsala: ArtDatabanken SLU. http://www.artsdatabanken.no/Rodliste/Artsgruppene/Alger
- Auderset Joye, D., and A. Rey-Boissezon. 2015. “Will Charophyte Species Increase or Decrease Their Distribution in a Changing Climate?” Aquatic Botany 120: 73–83. 10.1016/j.aquabot.2014.05.003
- Auderset Joye D., and A. Schwarzer. 2012. Liste Rouge Characées. Espèces menacées en Suisse, état 2010. [The 2010 Red List of Characeae. Threatened Species in Switzerland]. Office fédéral de l’environnement, Berne; Laboratoire d’ écologie et de biologie aquatique de l’Université de Genève. L’ environnement pratique no 1213, 72. Berne: OFEV.
- Auderset Joye, D., E. Castella, and J. B. Lachavanne. 2002. “Occurrence of Characeae in Switzerland over the Last Two Centuries.” Aquatic Botany 72: 369–385. 10.1016/S0304-3770(01)00211-X
- Baastrup-Spohr, L., L. Lønsmann Iversen, J. Dahl-Nielsen, and K. Sand-Jensen. 2013. “Seventy Years of Changes in the Abundance of Danish Charophytes.” Freshwater Biology 58: 1682–1693. 10.1111/fwb.12159
- Blaženčič, J., B. Stevanovič, Z. Blaženčič, and V. Stevanovič. 2006a. “Distribution and Ecology of Charophytes Recorded in the West and Central Balkans.” Cryptogamie: Algologie 27: 311–322.
- Blaženčič, J., B. Stevanovič, Z. Blaženčič, and V. Stevanovič. 2006b. “Red Data List of Charophytes in the Balkans.” Biodiversity and Conservation 15: 3445–3457.
- Blindow, I. 1992. “Decline of Charophytes during Eutrophication: Comparison with Angiosperms.” Freshwater Biology 28: 9–14. 10.1111/fwb.1992.28.issue-1
- Boissezon, A. 2014. “Distribution et dynamique des communautés de Characées: Impact des facteurs environnementaux régionaux et locaux.” [Distribution and dynamics of Characeae communities: Effects of regional and local environmental factors. PhD diss. no 4668, University of Geneva. https://archive-ouverte.unige.ch/unige:38416
- Boissezon, A. and D. Auderset Joye, and T. Garcia. 2017. « Temporal and Spatial Changes in Population Structure of the Freshwater macroalgae Nitellopsis obtusa (Desv.) J. Groves. » Botany Letters (this issue). doi: 10.1080/23818107.2017.1356239
- Bonis, A., and P. Grillas. 2002. “Deposition, Germination and Spatio-temporal Patterns of Charophyte Propagule Banks: A Review.” Aquatic Botany 72: 235–248. 10.1016/S0304-3770(01)00203-0
- Bonis, A., J. Lepart, and F. Laloé. 1996. “Effet de la température sur l’installation et la croissance des plantes annuelles de marais temporaires méditerranéens.” [Effects of water temperature on the establishment and growth of annual plants in temporary Mediterranean marshes] Canadian Journal of Botany 74: 1086–1094. 10.1139/b96-133
- Bornette, G., and S. Puijalon. 2009. “Macrophytes: Ecology of Aquatic Plants.” In: Encyclopedia of Life Sciences (ELS), Vol. 55, 1–9. Chichester: John Wiley & Sons. http://www.els.net/WileyCDA/ElsArticle/refId-a0020475.html
- Calero, S., W. Colom, and M. A. Rodrigo. 2015. “The Phenology of Wetland Submerged Macrophytes Related to Environmental Factors.” Limnetica 34: 425–438.
- Calero, S., D. Auderset Joye, A. Rey-Boissezon, and M. A. Rodrigo. 2017. “Time and Heat for Sexual Reproduction: Comparing the Phenology of Chara hispida of Two Populations at Different Latitudes.” Aquatic Botany 136: 71–81. 10.1016/j.aquabot.2016.09.011
- Casanova, M. T. 1994. “Vegetative and Reproductive Responses of Charophytes to Water Level Fluctuations in Permanent and Temporary Wetlands in Australia.” Australian Journal Marine and Freshwater Research 45: 1409–1419. 10.1071/MF9941409
- Casanova, M. T., and M. A. Brock. 1990. “Charophyte Germination and Establishment from the Seed Bank of an Australian Temporary Lake.” Aquatic Botany 36: 247–254. 10.1016/0304-3770(90)90038-M
- Casanova, M. T., and M. A. Brock. 1996. “Can Oospore Germination Patterns Explain Charophyte Distribution in Permanent and Temporary Wetlands?” Aquatic Botany 54: 297–312. 10.1016/0304-3770(96)01032-7
- Casanova, M. T., and M. A. Brock. 1999. “Charophyte Occurrence, Seed Banks and Establishment in Farm Dams in New South Wales.” Australian Journal of Botany 47: 437–444. 10.1071/BT97099
- Casanova, M. T., and M. A. Brock. 2000. “How Do Depth, Duration and Frequency of Flooding Influence the Establishment of Wetland Plant Communities?” Plant Ecology 147: 237–250. 10.1023/A:1009875226637
- Chuine, I., and E. G. Beaubien. 2001. “Phenology is a Major Determinant of Tree Species Range.” Ecology Letters 4: 500–510. 10.1046/j.1461-0248.2001.00261.x
- Clesceri, L. S., A. E. Greenberg, and A. D. Eaton. 1999. Standard Methods for the Examination of Water and Wastewater. Washington DC: American Public Health Association.
- Coops, H., M. Beklioglu, and T. L. Crisman. 2003. “The Role of Water Level Fluctuations in Shallow Lake Ecosystems – Workshop Conclusions.” Hydrobiologia 506: 23–27. 10.1023/B:HYDR.0000008595.14393.77
- Crosslé, K., and M. A. Brock. 2002. “How Do Water Regime and Clipping Influence Wetland Plant Establishment from Seed Banks and Subsequent Reproduction?” Aquatic Botany 74: 43–56. 10.1016/S0304-3770(02)00034-7
- De Winton, M. D., M. T. Casanova, and J. S. Clayton. 2004. “Charophyte Germination and Establishment under Low Irradiance.” Aquatic Botany 79: 175–187. 10.1016/j.aquabot.2004.01.013
- Doege, A., K. van de Weyer, R. Becker, and H. Schubert. 2016. “Bioindication mit Characeen” [Bioindication with Characeae] Chapter 8 in Armleuchteralgen. Die Characeen Deutschlands, edited by Arbeitsgruppe Characeen Deutschlands, 97–137. Berlin: Springer.
- Franklin, K. A. 2009. “Light and Temperature Signal Crosstalk in Plant Development.” Current Opinion in Plant Biology 12: 63–68. 10.1016/j.pbi.2008.09.007
- Fredriksen, S., V. Husa, S. Schneider and K. Sjøtun. 2015. “Alger (Cyanophyta, Rhodophyta, Chlorophyta og Ochrophyta).” In Norsk rødliste for arter 2015. [Algae. In: The 2015 Norwegian Red List for Species]. Trondheim: Artsdatabanken.
- Gauthier, P. and P. Grillas. 2004. “The Hydrological Regime and the Composition of the Vegetation”. In: Mediterranean Temporary Pools. Volume 1. Issues Relating to Conservation, Functioning and Management, edited by P. Grillas, P. Gauthier, N. Yavercovski and C. Perennou. La Tour du Valat: Medwet. https://medwet.org/publications/mediterranean-temporary-pools.
- Grillas, P. 1990. “Distribution of Submerged Macrophytes in the Camargue in Relation to Environmental Factors.” Journal of Vegetation Science 1: 393–402. 10.2307/3235716
- Harwell, M. C., and K. E. Havens. 2003. “Experimental Studies on the Recovery Potential of Submerged Aquatic Vegetation after Flooding and Desiccation in a Large Subtropical Lake.” Aquatic Botany 77: 135–151. 10.1016/S0304-3770(03)00101-3
- Hutchinson, G. E. 1975. A Treatise on Limnology, Vol. 3. Limnological Botany. New York: John Wiley & Sons.
- Karol, K. G., R. M. McCourt, M. T. Cimino, and C. F. Delwiche. 2001. “The Closest Living Relatives of Land Plants.” Science 294: 2351–2353. 10.1126/science.1065156
- Kleeberg, A., J. Kohler, T. Sukhodolova, and A. Sukhodolov. 2010. “Aquatic Macrophytes on Organic Matter Deposition, Resuspension and Phosphorus Entrainment in a Lowland River.” Freshwater Biology 55: 326–345. 10.1111/fwb.2010.55.issue-2
- Korsch, H., A. Doege, U. Raabe, and K. van de Weyer. 2013. “Rote Liste der Armleuchteralgen (Charophyceae) Deutschlands.” [Red List of Charophytes in Germany] Haussknechtia Beiheft 17: 1–32.
- Krause, W. 1981. “Characeen als Bioindikatoren für den Gewässerzustand.” [Characeae as Bioindicator for Water Conditions] Internationale Revue der gesamten Hydrobiologie und Hydrographie 2: 126–152.
- Krause, W. 1997. “Charales (Charophyceae).” In Süsswasserflora von Mitteleuropa, edited by H. Ettl, G. Gärtner, H. Heynig and D. Mollenhauer, Vol. 18, 202. Stuttgart: Fischer.
- Lancashire, P. D., H. Bleiholder, T. van den Boom, P. Langeluddeke, R. Stauss, E. Weber, and A. Witzenberger. 1991. “A Uniform Decimal Code for Growth Stages of Crops and Weeds.” Annals of Applied Biology 119: 561–601. 10.1111/aab.1991.119.issue-3
- Lauber, K., G. Wagner, and A. Gigax. 2012. Flora Helvetica: Flore illustrée de Suisse. 4th ed. Berne: Haupt.
- MeteoSuisse. 2017. Bulletin climatologique de l’année 2016. Genève. https://www.meteosuisse.admin.ch/content/dam/meteoswiss/fr/Klima/Gegenwart/doc/bulletin_climato_annee_2016_f.pdf
- MétéoSuisse. 2015. Climate of Switzerland. Genève: Federal Office of Meteorology and Climatology MeteoSwiss . https://www.meteoswiss.admin.ch/.
- Morellato, L. P. C., B. Alberton, S. T. Alvarado, B. Borges, E. Buisson, M. G. G. Camargo, L. F. Cancian, et al. 2016. “Linking Plant Phenology to Conservation Biology.” Biological Conservation 195: 60–72. 10.1016/j.biocon.2015.12.033
- Nicol, J. M., G. G. Ganf, and G. A. Pelton. 2003. “Seed Banks of a Southern Australian Wetland: The Influence of Water Regime on the Final Floristic Composition.” Plant Ecology 168: 191–205. 10.1023/A:1024430919811
- OCDE. 1982. Eutrophisation des eaux: Méthodes de surveillance, d’évaluation et de lutte. [Eutrophication of Waters: Monitoring, Assessment and Control]. Paris: Organisation for Economic Co-operation and Development.
- Prunier, P., F. Greulich, C. Béguin, R. Delarze, O. Heeg, F. Klötzli, R. Pantke, P. Steffen, P. Steiger, and P. Vittoz. 2014. “PhytoSuisse: un référentiel pour les associations végétales de Suisse”. https://www.infoflora.ch/fr/milieux/phytosuisse/
- R Development Core Team. 2016. R: A Language and Environment for Statistical Computing. Vienna, Austria: R Foundation for Statistical Computing. https://www.r-project.org.
- Rey-Boissezon, A., and D. Auderset Joye. 2015. “Habitat Requirement of Charophytes – Evidence of Species Discrimination through Distribution Analysis.” Aquatic Botany 120: 84–91. 10.1016/j.aquabot.2014.05.007
- Rodrigo, M. A., J. L. Alonso-Guillén, and I. Soulié-Märsche. 2010. “Reconstruction of the Former Charophyte Community out of the Fructifications Identified in Albufera de València Lagoon Sediments.” Aquatic Botany 92: 14–22. 10.1016/j.aquabot.2009.09.002
- Schneider, S., A. Garcia, C. Martin-Closas, and A. R. Chivas. 2015. “The Role of Charophytes (Charales) in Past and Present Environments: An Overview.” Aquatic Botany 120: 2–6. 10.1016/j.aquabot.2014.10.001
- Seabloom, E. W., A. G. van der Valk, and K. A. Moloney. 1998. “The Role of Water Depth and Soil Temperature in Determining Initial Composition of Prairie Wetland Coenoclines.” Plant Ecology 138: 203–216. 10.1023/A:1009711919757
- Simons, J., and E. Nat. 1996. “Past and Present Distribution of Stoneworts (Characeae) in the Netherlands.” Hydrobiologia 340: 127–135. 10.1007/BF00012744
- Soulié-Märsche, I. 1998. “Fossil Lamprothamnium Papulosum (Charophyta), a Biomarker for Seasonal Rainfall in Northern Mauritania.” Paleoecology of Africa and the Surrounding Islands 25: 65–76.
- Soulié-Märsche, I. 2004. “The Role of Charophytes in Temporary Pools.” In Mediterranean Temporary pools. Vol. 2 – Species information sheets, edited by P. Grillas, P. Gauthier, N. Yavercovski, and C. Perennou, 55–57. La Tour du Valat: Medwet. https://medwet.org/publications/mediterranean-temporary-pools
- Stewart, N. F., and J. M. Church. 1992. Red Data Books of Britain and Ireland. Peterborough: The Joint Nature Conservation Committee.
- Tukey, J. W. 1977. Exploratory Data Analysis. Boston, MA: Addison-Wesley.
- Wang, H., D. Yu, and K. Xiao. 2008. “The Interactive Effects of Irradiance and Photoperiod on Chara vulgaris L.: Concerted Responses in Morphology, Physiology, and Reproduction.” Hydrobiologia 610: 33–41. 10.1007/s10750-008-9420-2
- Warwick, N. W. M., and M. A. Brock. 2003. “Plant Reproduction in Temporary Wetlands: The Effects of Seasonal Timing, Depth, and Duration of Flooding.” Aquatic Botany 77: 153–167. 10.1016/S0304-3770(03)00102-5
- Williams, P., J. Biggs, A. Corfield, G. Fox, D. Walker, and M. Whitfield. 1997. “Designing New Ponds for Wildlife.” British Wildlife 8: 137–150.