Abstract
Reconstruction of Brown’s Class III maxillary defect can be challenging due to the complex geometry of maxilla. We aimed to introduce an improved method for maxillary reconstruction with a composite deep circumflex iliac artery (DCIA) flap aided by virtual surgical planning and intraoperative navigation. A 27-year-old woman diagnosed with left maxillary fibromyxoma was admitted to our institution in December 2018. Pre-operative facial and iliac computed tomography data were obtained for virtual surgical planning. Personalized cutting template, tooth-supported surgical guide, and rapid prototype model with reconstructed orbital floor were printed for pre-operative preparation. Surgery was completely guided by the intraoperative navigation system. The root mean square estimate of the reconstructed area was 3.68 mm. The average errors measured on the lateral and medial DCIA segments were 0.61 and 0.85 mm, respectively. Application of virtual surgical planning and intraoperative navigation could potentially enhance the reconstruction outcomes.
1. Introduction
Reconstruction of maxillary defects following tumor ablation remains one of the most technically challenging procedures in oral and maxillofacial surgery. Maxilla primarily provides structural support to the globe and framework to facial musculature and is also crucial in providing the oronasal, oroantral partition, and foundation for dentoalveolar units [Citation1]. Its unique hexahedron shape [Citation2] and complex curvatures makes reconstruction even more challenging. Choices of reconstruction may differ from obturators to locoregional pedicled flaps or vascularized free flaps depending on the types of maxillary defects.
In this study, we primarily focused on reconstruction of Brown’s Class III maxillary defects. Brown’s Class III vertical maxillary defects primarily involve dentoalveolar and orbital components while preserving the globe [Citation3]. Such defects often result in extensive bone loss that significantly alters facial appearance, the original orbital apparatus, and masticatory functions. Numerous methods of reconstruction for maxillary defects have been published, among which the temporalis flap, rectus abdominus, and fibula flap are well-accepted choices [Citation3]. However, none of these reconstructive procedures are single-handedly sufficient to achieve all aforementioned reconstructive goals [Citation4].
Deep circumflex iliac artery (DCIA) flap is a reliable option for reconstruction of Class III and Class IV maxillary defects considering its superior bone stock and soft tissue bulk in restoring both bony and soft tissue defects [Citation3,Citation5]. However, manipulation of the bone flap is tricky as the ordinary contour of the iliac crest does not coincide with the natural facial profile [Citation6]. Traditionally, most surgical procedures are heavily relied on the surgeon’s experience, and the outcomes of reconstruction are occasionally unsatisfactory. With the development of computer-assisted techniques, the reconstructive procedures were improved and better clinical outcomes can be achieved [Citation7,Citation8]. In this study, multiple computer-assisted techniques including the use of cutting guides, patient-specific titanium mesh and intraoperative navigation system were applied. Based on these techniques, we demonstrated a feasible and practical surgical flow which enhanced the final surgical outcomes of extensive maxillary defects reconstruction.
2. Patient and methods
2.1. Demographic data
A 27-year-old woman was referred to our center in December 2018 for management of left maxillary tumor. Histopathological examination following an incisional biopsy revealed left maxillary fibromyxoma. Orthopantomogram (OPG) and computed tomography (CT) scan revealed a multilocular radiolucent lesion with sclerotic margins of left maxilla, with cortical thinning and buccal plate perforation at the anterior part of left maxillary sinus.
2.2. Virtual surgical planning
High-resolution preoperative CT scan of head and neck and pelvis with 1.0 mm slice thickness (field of view, 20 cm; pitch, 1.0; slice, 0.75 mm; 120Y280 mA) were performed. Digital Imaging and Communications in Medicine (DICOM) format data was uploaded onto image-guided surgery (IGS) software, iPlan CMF 3.0 (BrainLAB, Feldkirchen, Germany) for tumor mapping and surgical simulation. Tumor mapping allows direct three-dimensional (3D) visualization of the tumor and its relationship with adjacent vital structures.
Proplan CMF 3.0 (Materialise, Leuven, Belgium) was used for data conditioning, segmentation, and virtual surgical planning. 3D rendering was executed to produce a 3D model, subsequently, manual segmentation of the maxilla and mandible was performed. Virtual maxillectomy was done based on clinical, radiological findings, and 3D tumor mapping (). Virtual reconstruction of the affected orbital floor and maxillary defect was performed by simulating the opposing unaffected orbit and maxilla using mirroring technique while making allowance for future rehabilitation options to ensure optimal position of the iliac crest segment (). Rapid prototype model with the reconstructed orbital floor was printed for contouring of the titanium mesh pre-operatively ().
Figure 3. Three-dimensional model of the reconstructed maxilla and titanium mesh was precontoured on the model.
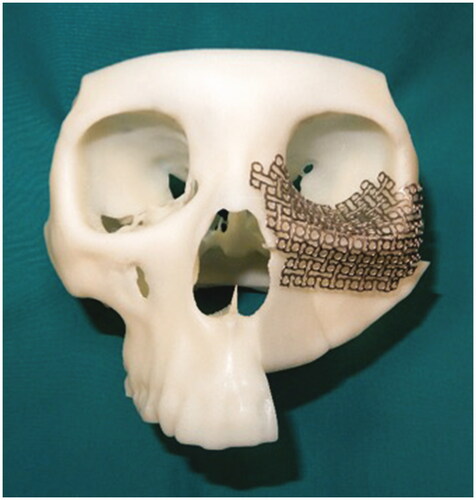
Based on the reconstructed model, the iliac crest cutting template and tooth-supported stereolithographic surgical guide were designed and fabricated using 3D printing technology to facilitate harvesting and intraoperative positioning of the bony segments.
Each components of the surgical plan, including osteotomized maxilla and iliac crest segment, were imported into iPlan CMF 3.0 (BrainLAB, Feldkirchen, Germany) via Standard Tessellation Language (STL) files and registered with the original CT data. Final navigation data was exported and transferred to the navigation work station for further handling during surgery.
2.3. Navigation surgery
The navigation system used in the study was iPlan CMF 3.0. Following scalp incision, the skull post was firmly anchored to the cranium with a 6-mm self-tapping screw. Following attachment of dynamic reference frame (DRF) to the skull post, laser surface scanning (z-touch®) was used to perform facial skin surface matching, primarily at the periorbital region, which allowed registration of patient’s orientation with the intra-operative navigation dataset.
Tumor resection was guided by intraoperative navigation system with planned surgical margins of approximately 1.0 cm to ensure tumor-free margins. Each osteotomy line was made using fissure bur and confirmed with intraoperative navigation system, completed with reciprocating saw.
Harvesting of the DCIA flap was performed by simultaneous two-team approach aided by the 3D printed cutting template. The internal and external oblique muscles were harvested to obliterate the maxillary defect and to restore the palatal defect, respectively. The position of the prefabricated titanium mesh was confirmed using the navigation system and fixed to the zygoma and nasal bone. The harvested DCIA flap was transferred to the recipient site () with its pedicle tunneled through the left temporal region for anastomosis with left superficial temporal artery and vein. The position of the iliac crest segments was guided by the surgical template and confirmed with the intraoperative navigation ().
3. Results
The flap survived uneventfully, and the patient was followed up for 10 months. The patient was pleased with her appearance (). Ophthalmic assessment revealed normal visual acuity and ocular motility without any signs of diplopia. The internal oblique muscle was fully mucosalized, with good oronasal and oroantral separation achieved. No remarkable complications were noted at both recipient and donor sites.
Post-operative plain facial CT scan was performed to evaluate the final surgical outcomes. To further analyze accuracy of the reconstruction, the post-operative 3D model was superimposed with the pre-operative surgical model using Geomagic Studio 2013 (3D System). Using manual and global registration, the preoperative surgical model was superimposed to the postoperative 3D model and areas of interest (the DCIA flap) were selected manually. The accuracy of the reconstructed area was assessed by calculating the root mean square (RMS) estimate, which was 3.68 mm. RMS value indicated the direct mean deviation by directly comparing the reconstructed area, which was generated automatically and expressed on color map, hence representing the accuracy of the reconstruction. A lower RMS value denoted a smaller difference between the two models, thus representing lesser error of reconstruction [Citation9] (). The accuracy of osteotomies between the two DCIA segments was also evaluated, the average errors measured on the lateral and medial segments were 0.61 and 0.85 mm, respectively.
4. Discussion
Maxillary defects following tumor ablation commonly result in Brown’s Class II or III maxillary defects. Such defects can drastically affect the facial appearance, functions, quality of life, and often leaving debilitating physiological and psychological impact on both patients and their families. Reconstruction of maxillary defects, particularly Brown’s Class III defects, remains one of the most complex procedures owing to its substantial loss of both soft and hard tissues. Free fibula flaps have been widely used for reconstruction of maxillary defects, particularly Brown’s Class II maxillary defects [Citation3,Citation5,Citation10]. Nevertheless, the bony and soft tissue volume are usually insufficient thus resulting in facial depression as the defects frequently involve the zygomatic complex and the orbital floor [Citation5,Citation10]. Vascularized DCIA composite flap is preferred for such extensive defect in view of its bone stock quality and the mucosalized internal oblique muscle offers a superior environment for future dental rehabilitation. However, complex harvesting process and its short vascular pedicle length may limit the utilization in reconstruction of maxillary defects [Citation11]. Ong et al. [Citation6] described a reproducible technique for maxillary reconstruction with the DCIA flap using personalized cutting guides. Considering that ideal functional and esthetic reconstruction of extensive maxillary defects is challenging, we improved such surgical techniques and incorporated virtual surgical planning with intraoperative navigation in this study to improve accuracy of the reconstruction, and the surgical outcomes were promising. To our knowledge, there are only few reports that described the utilization of multiple computer-assisted techniques in oral and maxillofacial reconstruction, particularly in reconstruction of extensive maxillary defect with DCIA flap. This is the first report that incorporated multiple computer-assisted surgery (CAS), particularly intraoperative navigation, into reconstruction of an extensive maxillary defect with a vascularized DCIA flap.
In this study, we used CAS, which includes preoperative virtual surgical planning, stereolithographic models, personalized cutting guides, and intraoperative navigation. The guidance of intraoperative navigation allowed accurate resection of the tumor according to the preoperative virtual surgical plan.
Stereolithographic models and personalized surgical guides have been routinely used in our reconstructive procedures in the past 5 years, and these are particularly valuable in precontouring of titanium plates or meshes [Citation7,Citation12,Citation13]. With the aid of custom cutting guides for harvesting of the DCIA flap, the amount of bone can be estimated preoperatively, thus shortening the total operating and ischemic time as well as reducing unnecessary harvesting of bone flap and muscle stripping. Several studies have incorporated personalized cutting guides and positioning templates for maxillary reconstruction to improve precision of surgical outcomes [Citation6,Citation14]. In this case, the personalized tooth-supported surgical guide provided guidance for shaping and positioning, particularly in the buccopalatal aspect. Fitting of the surgical guide can be improved by rendering additional space for the muscle attachment during virtual surgical planning.
Precontoured titanium meshes based on 3D printed models allow precise replication of the complex anatomy of the orbital floor. Additionally, it significantly minimizes the total operating time as the titanium mesh can be prepared pre-operatively. However, the accuracy of stereolithographic models is largely dependent on the quality of image data sets as the orbital floors are often poorly reconstructed in stereolithographic models. Furthermore, streak artifacts and noise from metals such as dental restorations may undesirably affect the accuracy and quality of such models [Citation15]. Considering the potential errors from these rapid prototyping models and guides, we combined intraoperative navigation in this study to further improve the precision of reconstruction. Intraoperative navigation is particularly valuable in verifying the final position of the titanium mesh during surgery without impinging orbital structures. In our previous studies, satisfactory clinical outcomes were achieved using computer-assisted personalized titanium meshes combined with intraoperative navigation [Citation12,Citation16]. Several registration methods were available for patient registration in computer-assisted navigation surgery. Skin surface registration was utilized in this study as this is less invasive and the accuracy of the registration is adequate, approximately 2.4 ± 1.7 mm (range 1–9 mm). Furthermore, higher precision was noted in surgical field with closer proximity to the registration site [Citation17].
Reconstruction of the orbital floor following globe-sparing total maxillectomy is particularly crucial for maintenance of the globe position. Several methods have been advocated, including alloplastic materials such as titanium meshes, Medpor and autogenous bone grafts such as iliac bone, cranial bone, or rib grafts [Citation10,Citation15]. Individualized prefabricated titanium meshes were used in our study to reconstruct orbital floor defects for its simplicity and flexibility in simulating the irregular orbital floor contour.
While the maxillary alveolus can be reconstructed with the iliac crest, reconstruction of such palatal defects can be challenging in consideration of future dental restoration. One of the most remarkable advantages of the DCIA flap was the adequate soft tissue could be harvested. In this study, the internal oblique muscle was used to obliterate the dead space and to line the nasal passage, while the external oblique muscle with fascia was used to reconstruct the palatal mucosa. DCIA flap still present with limitations, particularly comparing with fibula flaps; its short pedicle length commonly makes anastomosis more technically demanding as the distance from the facial vessels is noticeably further away. Various options were discussed such as vein grafts or anastomosis with ipsilateral superficial temporal vessels or facial vessels via the intraoral approach. In this study, we opted for superficial temporal vessels due to its consistent anatomical course [Citation18].
Although intraoperative navigation is ingenious in providing reliable and precise control in surgical fields, the high technical costs and potential systematic errors could limit its use in routine reconstructive surgery [Citation12]. Furthermore, mastering such advanced technology certainly warrants a learning curve for surgeons.
5. Conclusion
Reconstruction of Brown’s Class III maxillary defect is a technically demanding procedure. Composite DCIA flap is nowadays a good reconstructive options for such defect. This present case showed a feasible and practical surgical flow which enhanced the final surgical outcomes. The use of cutting guides is advantageous in harvesting and shaping bone graft, while the incorporation of navigation system is important in ultimate verification. Computer-assisted techniques including virtual surgical planning and intraoperative navigation are prospective techniques in improving accuracy of reconstruction and achieving favorable clinical outcomes. However, there were a number of limitations in this study and would benefit from further research by increasing the sample size to further validate the accuracy of the current technique.
Disclosure statement
None of the authors has a financial interest in any of the products, devices, or drugs mentioned in this manuscript.
Additional information
Funding
References
- Fattahi T. Surgical anatomy of the maxillary region. Atlas Oral Maxillofac Surg Clin North Am. 2007;15(1):1–6.
- Cordeiro PS. A classification system and algorithm for reconstruction of maxillectomy and midfacial defects. Plast Reconstr Surg. 2000;105:2347–2348.
- Brown JS, Shaw RJ. Reconstruction of the maxilla and midface: Introducing a new classification. Lancet Oncol. 2010;11(10):1001–1008.
- Futran ND. Primary reconstruction of the maxilla following maxillectomy with or without sacrifice of the orbit. J Oral Maxillofac Surg. 2005;63(12):1765–1769.
- Peng X, Mao C, Yu GY, et al. Maxillary reconstruction with the free fibula flap. Plast Reconstr Surg. 2005;115(6):1562–1569.
- Ong HS, Yu M, Liu JN, et al. Modified maxillary reconstruction technique for Brown’s class III defects by subdividing a rhomboid shaped iliac crest into 2 subunits. Head Neck. 2018;40:1824–1833.
- Zhang WB, Wang Y, Liu XJ, et al. Reconstruction of maxillary defects with free fibula flap assisted by computer techniques. J Cranio-Maxillofacial Surg. 2015;43(5):630–636.
- Yu Y, Zhang WB, Liu XJ, et al. Three-dimensional accuracy of virtual planning and surgical navigation for mandibular reconstruction with free fibula flap. J Oral Maxillofac Surg. 2016;74(7):1503.e1–1503.e10.
- Jeong YG, Lee WS, Lee KB. Accuracy evaluation of dental models manufactured by CAD/CAM milling method and 3D printing method. J Adv Prosthodont. 2018;10(3):245–251.
- Nd F, Jt W, D V, et al. Midface reconstruction with the fibula free flap. Arch Otolaryngol - Head Neck Surg. 2002;128:161–166.
- Massimo Maranzano AA. The versatility of vascularized iliac crest with internal oblique muscle flap for composite upper maxillary reconstruction. Microsurgery. 2007;27(1):37–42.
- Zhang WB, Mao C, Liu XJ, et al. Outcomes of orbital floor reconstruction after extensive maxillectomy using the computer-assisted fabricated individual titanium mesh technique. J Oral Maxillofac Surg. 2015;73(10):2065.e1–2065.e15.
- Yu Y, Zhang WB, Wang Y, et al. A revised approach for mandibular reconstruction with the vascularized iliac crest flap using virtual surgical planning and surgical navigation. J Oral Maxillofac Surg. 2016;74(6):1285.e1–1285.e11.
- Shen Y, Sun J, Li J, et al. Special considerations in virtual surgical planning for secondary accurate maxillary reconstruction with vascularised fibula osteomyocutaneous flap. J Plast Reconstr Aesthet Surg. 2012;65(7):893–902.
- Edwards SP. Computer-assisted craniomaxillofacial surgery. Oral Maxillofac Surg Clin North Am. 2010;22(1):117–134.
- Markiewicz MR, Dierks EJ, Bell RB. Does intraoperative navigation restore orbital dimensions in traumatic and post-ablative defects? J Cranio-Maxillofac Surg. 2012;40(2):142–148.
- Raabe A, Krishnan R, Wolff R, et al. Laser surface scanning for patient registration in intracranial image-guided surgery. Neurosurgery. 2002;50(4):797–803.
- Koziej M, Wnuk J, Polak J, et al. The superficial temporal artery: a meta-analysis of its prevalence and morphology. Clin Anat. 2020;33(8):1130–1137.