Abstract
The aim of the study was to investigate the biomechanical behavior of three-dimensionally (3D)-printed surgical plates used for mandibular defect reconstruction, compare them with conventional surgical plates, and provide experimental evidence for their clinical application. Three-dimensional models were created for the normal mandible and for mandibular body defects reconstructed using free fibula and deep circumflex iliac artery flaps. Three-dimensional finite element models of reconstructed mandibles fixed using 3D-printed and conventional surgical plates were established. Vertical occlusal forces were applied to the remaining teeth and the displacement and Von Mises stress distributions were studied using finite element analysis. The normal and reconstructed mandibles had similar biomechanical behaviors. The displacement distributions for the surgical plates were similar, and the maximum total deformation occurred at the screw hole of the anterior segment of the surgical plates. However, there were differences in the Von Mises stress distributions for the surgical plates. In reconstructed mandibles fixed using 3D-printed surgical plates, the maximum equivalent Von Mises stress occurred at the screw hole of the posterior segment, while in those fixed using conventional surgical plates, the maximum equivalent Von Mises stress was at the screw hole of the anterior segment. In the mandible models reconstructed with the same free flap but fixed with different surgical plates, the plates had similar biomechanical behaviors. The biomechanical behavior of 3D-printed surgical plates was similar to conventional surgical plates, suggesting that 3D-printed surgical plates used to reconstruct mandibular body defects with vascularized autogenous bone grafts could lead to secure and stable fixation.
1. Introduction
Mandibular defects are common conditions in oral and maxillofacial surgery caused by tumor resection or trauma and often result in significant esthetic and functional deficits. Conventionally, individualized mandibular reconstruction is frequently preformed using surgical plates combined with vascularized autogenous bone grafts [Citation1,Citation2]. While, surgical plates required preoperative manual bending to match the contour of the jaw and the bone flap and final adjustments based on actual operating conditions during the procedure, which contributed to stress fatigue and surgical plate fracture [Citation3,Citation4], along with a considerable waste of manpower and time.
The development of three-dimensional (3D) printing techniques has popularized patient-specific surgical plates used for individualized mandibular defect reconstruction [Citation5–7]. Compared to conventional surgical plates, 3D-printed surgical plates achieve higher geometrical accuracy, simplify the operation, and reduce the recovery time [Citation8]. It has also been demonstrated that 3D-printed surgical plates have better mechanical properties [Citation9]. So, in the application of mandibular reconstruction, what is it about the biomechanical behavior of the 3D-printed surgical plates? Whether there is a significant difference between the 3D-printed and conventional surgical plates?
Therefore, the objectives of this study were to investigate the biomechanical behavior of 3D-printed surgical plates used for mandibular reconstructions and compare them with conventional surgical plates. The study aimed to improve the biomechanical performance of 3D-printed surgical plates and provide experimental evidence for clinical applications.
2. Materials and methods
2.1. Patient information and virtual surgical planning
The inclusion criteria were as follows: (1) the age of the volunteer was at least 18 years old, (2) male or female, (3) the volunteer should have a complete dentition and normal occlusion and (4) there was no history of mandibular, fibula, or iliac crest diseases or trauma of the volunteer.
The exclusion criteria were as follows: (1) the age of volunteer was under 18 years old and (2) the volunteer was treated for mandibular, fibula, or iliac crest diseases or trauma.
A healthy 23-year-old male with normal occlusion and no history of mandibular, fibula, or iliac crest diseases volunteered for the study.
Computed tomography (CT) of the maxillofacial skeleton, lower extremities, and the iliac crest was performed with a slice thickness of 1.25 mm (field of view, 20 cm; pitch, 1.0; tube current, 120-280mA). Maxillofacial CT was performed in a stable molar occlusion position. The range of CT scanning was from the calvaria to the menton of the maxillofacial skeleton, from the knee joint to the ankle joint of the lower extremities, and from the crista iliaca to the lower margin of the pubic symphysis of the iliac crest, respectively. CT data in Digital Imaging and Communications in Medicine (DICOM) format were imported to the ProPlan CMF 3.0 software (Materialise, Leuven, Belgium). The mandible and maxilla were segmented, followed by an L-type virtual right mandibulectomy from the distal aspect of the mandibular canine to the anterior margin of the ramus, based on the HCL classification of mandibular defects () [Citation10]. CT data for the lower extremities and iliac crest were also imported into the ProPlan CMF 3.0 software and the donor sites were segmented. The 3D fibular and iliac images were superimposed on the mandibular defect in a position based on the mandibular contour () [Citation11]. Two 3D models of the mandible with the free fibula and deep circumflex iliac artery flaps were constructed.
2.2. Preparation of conventional and 3D-printed surgical plates
After the computer-assisted virtual plans were generated, two mandibular stereolithographic models reconstructed using fibula and iliac crest were 3D printed. Two conventional surgical plates (MatrixMANDIBLE, 96 mm × 2.5 mm; DePuy Synthes, Raynham, MA, USA) were prebent and fixed on the reconstructed mandibular models using nine titanium alloy screws (MatrixMANDIBLE; DePuy Synthes) (). The plates had a thickness of 2.5 mm, a length of 96 mm, a screw hole diameter of 2.4 mm, and an 8.0 mm distance between adjacent holes. The screws had a length of 8.0 mm, a diameter of 2.4 mm, and a thread pitch of 1.0 mm.
Figure 3. Stereolithographic models of mandible reconstructed with free fibula flap (A) or deep circumflex iliac artery flap (B) fixed using manually prebent conventional surgical plates.
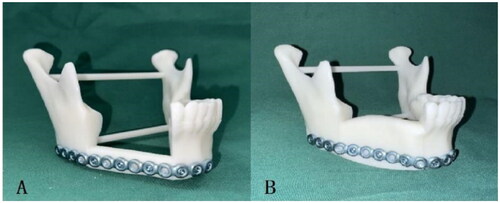
CT scans (field of view, 20 cm; pitch, 1.0; tube current, 120–280 mA) were performed for the two reconstructed mandibular models fixed with surgical plates to obtain positional data. The two surgical plates were scanned using a laboratory scanner (D2000; 3Shape, Copenhagen, Denmark) to obtain accurate data for the contours of the surgical plates. The data in Standard Tessellation Language (.stl) format were imported into the Mimics 19.0 software (Materialise), where the scanned surgical plates were matched to the reconstructed mandibles based on CT positional relationships ().
Figure 4. Conventional surgical plates matched to the mandible reconstructed with free fibula flap (A) and deep circumflex iliac artery flap (B).
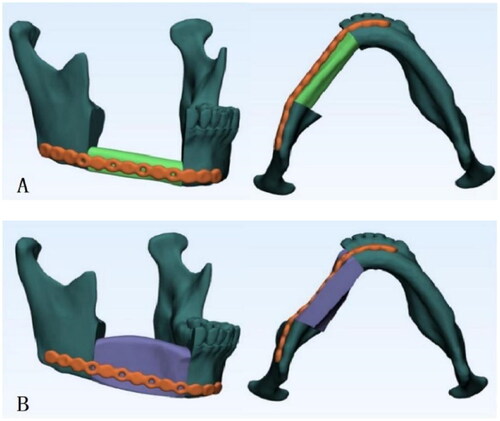
For 3D-printed surgical plates, first, a standardized prototype was designed in SolidWorks 2018 (Dassault Systèmes SE, Vélizy-Villacoublay, France) based on the width, thickness, screw hole positions and diameter, and distance between adjacent holes of a conventional surgical plate with an appropriate length. The design data were in .stl format. These data and the virtual surgical plan were imported into Mimics 19.0 software. The standardized surgical plate was customized specifically for the patient based on the outer surface anatomy of the reconstructed mandible, adjusting the length and screw hole positions in the remaining mandible and bone graft. Further optimization was made to improve the overall appearance and smooth the plate surface and edges, resulting in a perfect match between the customized surgical plate and the reconstructed mandible contour. Design data exported in .stl format were used for the 3D printing of the surgical plates ().
2.3. Model meshing
Since the reconstructed mandibular models had complex geometries, meshing was performed using tetrahedral elements to generate meshed 3D-FE models. The total numbers of nodes and elements in the resulting FE models are summarized in .
Table 1. Total number of nodes and elements of each finite element model.
2.4. FE analysis
2.4.1. Material properties
All components of the FE models, including the mandible, fibula, iliac crest, surgical plates, and screws, were considered homogeneous, isotropic, and linear elastic. The mandible was fully modeled as a cortical bone because it comprised the bulk of the remaining mandible. The 3D-printed surgical plates and fixation screws were made using titanium alloy (Ti-6Al-4V), which is commonly used as the raw material for patient-specific surgical plates [Citation9,Citation12], while conventional surgical plates were made using pure titanium. The properties of related materials used in the FE models are presented in [Citation12–14].
Table 2. Material properties of the materials used in finite element models.
2.4.2. Contact settings
Bonded contacts were used between teeth and the mandible, between screws and the surgical plate, and between screws and the mandible. Frictionless contacts were used between the mandible and the bone graft, between the mandible and the surgical plate, and between the graft and the surgical plate.
2.4.3. Boundaries and loading conditions
The mandibular condyles, coronoid process, and angles were restrained in all directions bilaterally. Considering the complexity of muscle activity, anatomical features that provide attachments to major masticatory muscles (masseter, temporalis, medial pterygoid, and lateral pterygoid) were determined and marked. The degrees of freedom for the nodes on these regions were kept zero [Citation15,Citation16].
Vertical occlusal forces were loaded on the remaining teeth, from the right mandibular canine to the left mandibular second molar, to simulate the centric bite. Bite forces loaded on each tooth are shown in [Citation15,Citation17].
Table 3. Loading values and tooth positions.
FE analysis was performed using the ANSYS Workbench 18.1 software (ANSYS, Inc., Canonsburg, PA, USA) to determine the displacement and Von Mises stress distributions in the mandibular defect models reconstructed using different autogenous bone grafts and fixed using different surgical plates.
3. Results
Four different mandibles were reconstructed using combinations of free fibula and deep circumflex iliac artery flaps with 3D-printed and conventional surgical plates. A comparison of the maximum total deformation and maximum equivalent Von Mises stress in the normal mandible, reconstructed mandibles, and surgical plates is presented in . Detailed displacement and Von Mises stress distributions for the normal mandible, reconstructed mandibles, and surgical plates are shown in , respectively. The effectiveness of the two surgical plates was compared through an analysis of the simulation results.
Figure 6. Displacement distribution (A and B) and Von Mises stress distribution (C and D) of the normal mandible.
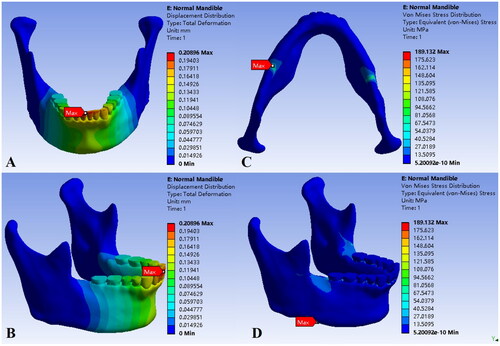
Figure 7. Displacement distribution of the mandibles reconstructed using free fibula flap (A1 and B1), deep circumflex iliac artery flap (C1 and D1), 3D-printed surgical plate (A2 and C2), and conventional surgical plate (B2 and D2).

Figure 8. Von Mises stress distribution of the mandibles reconstructed using free fibula flap (A1 and B1), deep circumflex iliac artery flap (C1 and D1), 3D-printed surgical plate (A2 and C2), and conventional surgical plate (B2 and D2).
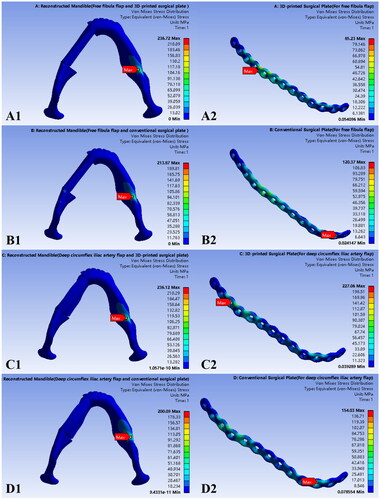
Table 4. Comparison of maximum total deformation and equivalent Von Mises stress of the components of different finite element models.
3.1. Normal mandible
Based on the statics analysis, under bilateral vertical loading in centric occlusion, the displacement distribution of the normal mandible was characterized by continuous changes from the fixed and constrained parts, including the mandibular condyle, coronoid process, and angle on both sides, to the front. The maximum total deformation was 0.2090 mm in the central incisor region. High stress areas were concentrated at the lower edge of the mandibular body, corresponding to mandibular third molars. The maximum equivalent Von Mises stress was 189.13 MPa at the lower edge of the right mandibular body.
3.2. Mandibular defects reconstructed with free fibula flaps
In the models of mandibular body defects reconstructed with free fibula flaps and fixed with 3D-printed or conventional surgical plates, the mandibles and the plates had similar displacement distributions, which was a continuous change from posterior fixed and constrained parts to the anterior mandible. The maximum total deformation values of the reconstructed mandibles were 0.3059 and 0.3044 mm in the right mandibular central incisor region for fixed with 3D-printed and conventional surgical plates, respectively (). The maximum total deformation values for the surgical plates were 0.2268 and 0.2239 mm, respectively, and occurred in front of the anterior osteotomy line at the lower edge of the middle screw hole in the anterior segment of the plate (). The mandibles or surgical plates of the two models exhibited the approximate maximum total deformation.
The reconstructed mandibles had similar maximum equivalent Von Mises stress values, 236.72 and 213.87 MPa for fixed with 3D-printed and conventional surgical plates, respectively, at the lower edge of the left mandibular body, corresponding to the mandibular third molar area (). However, the two surgical plates had differences in the Von Mises stress distribution (). The maximum equivalent Von Mises stress for 3D-printed surgical plates was between the two screw holes corresponding to the posterior osteotomy line, with a value of 85.23 MPa. For conventional surgical plates, the maximum equivalent Von Mises stress was in front of the anterior osteotomy line, near the middle screw hole of the anterior segment of the plate, with a value of 120.37 MPa. It demonstrated that the gap between the maximum equivalent Von Mises stress of mandibles or surgical plates of the two models was small.
3.3. Mandibular body defects reconstructed with deep circumflex iliac artery flaps
The displacement distribution and maximum total deformation for the models of mandibular body defects reconstructed with deep circumflex iliac artery flaps and fixed using 3D-printed or conventional surgical plates are illustrated in . Both models exhibited similar displacement distributions that varied continuously from back to the front. The maximum total deformation values for the reconstructed mandibles were 0.3235 and 0.2981 mm for fixed with 3D-printed and conventional surgical plates, respectively, in the right lateral incisor region (). For the surgical plates, the maximum total deformation values were 0.2687 and 0.2415 mm, respectively, in front of the anterior osteotomy line at the lower edge of the screw hole of the anterior segment of the surgical plates ().
The maximum equivalent Von Mises stress values for the reconstructed mandibles were 236.12 and 200.09 MPa for fixed with 3D-printed and conventional surgical plates, respectively, and occurred at the lower edge of the left mandibular body (). It could be seen from that the maximum equivalent Von Mises stress for the 3D-printed surgical plate was 227.06 MPa behind the posterior osteotomy line, at the screw hole of the posterior segment of the surgical plate, while that for the conventional surgical plate was 154.03 MPa in front of the anterior osteotomy line, at the screw hole at the anterior segment of the surgical plate.
The maximum total deformation values of the mandibles or surgical plates of the two models were similar, while the difference between the maximum equivalent Von Mises stress values was relatively small.
4. Discussion
The 3D printing technology has become the focus of clinical research and applications in mandibular reconstruction. However, further research is required to determine whether their mechanical properties meet the relevant standards and whether they possess biomechanical properties comparable to similar products already in clinical use. The results of these studies may provide an important reference for future research and clinical applications. In a previous study, we demonstrated that 3D-printed surgical plates had better hardness, bending strength, tensile strength, and yield strength compared to conventional surgical plates in mandibular reconstruction [Citation9]. FE analysis is frequently used to analyze the biomechanical behavior of materials based on displacement and stress. FE analysis uses mathematical approximation to simulate complex physical systems, including their geometries and loading conditions, which can approach actual system parameters by using simple interacting elements. Digital technology can be used for virtual designs and surgeries and can confirm the properties of materials under specific experimental conditions to establish a digital 3D model that fulfills the application requirements. FE analysis can be used to simulate different types of loads to explore the biomechanical behavior of 3D models and assess their displacement and stress distributions. This method is widely used in research and application of surgical plates used for jaw defect reconstruction [Citation16,Citation18].
Previous studies have used various methods to set up FE models. As for the systematic review of the previous studies, the National Library of Medicine was searched using MEDLINE by means of PubMed. In order to identify articles relating specifically to mandibular reconstruction with the method of surgical plates manufactured by three dimensional printing technology, we used the Medical Subject Headings term ‘mandible’, ‘mandibular’, ‘reconstruction’, ‘three dimensional printing’, and ‘plate’ as the first search terms to select for articles generally. In addition, a focused search to identify studies which related to our research was performed by using a set of specific terms ‘biomechenical’ or ‘biomechenical behavior’ or ‘finite element’ or ‘finite element analysis’. Finally, the articles or researches which could provide useful references for the study were quoted by the authors.
Knoll et al. [Citation19] established a number of models for mandibular angle defects by designing various surgical plates for reconstruction. They compared different kinds of modified plates with the commercially available plate (standard plate) and mainly focused on the effect of plate geometry as well as the configuration and the diameter of the screws on stress distribution with a single vertical chewing force for loading. As for the study, the reconstruction plate used as control had a big difference in geometry with the experimental reconstruction plates, the mandibular defects were only reconstructed with reconstruction plates and without bone grafts, and more attention was paid on the effects of configuration of screw holes on the modified plates. Zhong et al. [Citation20] validated the biomechanical benefit of the structural optimized patient-specific mandibular reconstruction plates and compared the biomechanical performance between two kinds of related plates, consisting of the generally designed one and the mandibular angle region modified one. Each plate showed different advantages in biomechanical performance. However, likewise, the mandibular reconstruction assemblies were created only with the remaining mandibular bone and two kinds of plates. Therefore, for future research, the topology optimization and biomimetic scaffold should be introduced to further advance the study and application of 3D printed patient-specific reconstruction plates. Cheng et al. [Citation21] used free fibula flaps in different vertical positions to reconstruct segmental mandibular defects of the mandible and found that grafts placed at the intermediate location had the best biomechanics and provided the most favorable conditions for prosthetic rehabilitation. For large mandibular defects, individualized reconstruction using microvascular fibular grafts has advantages over other alternatives in terms of blood supply and good quality of grafted bone. More importantly, the surgical plates play a vital role in the fixation and reconstruction. Therefore, in our opinion, the bone graft and the plate should be studied as an organic whole, however, the study only focused on the bone graft and did not take the surgical plate into consideration, which might have a significant influence on the final results and the conclusions obtained might be not complete or strong enough. Shi et al. [Citation22] preclinically assessed the biomechanical feasibility of a combination of short surgical plates to replace a conventional single long surgical plate for extensive mandibular defect reconstruction with fibula graft. Four different patient-specific customized surgical plates, including three different combinations of long, medium-long and short plates and a conventional single long plate were designed, and analyzed and compared by finite element analysis. They drew the conclusion that compared to the conventional single long surgical plate, only one kind of customized surgical plate, which was a combination of a long plate and a short plate, showed superior biomechanical properties in extensive mandibular defect reconstruction. While the number and position of screws to fix the surgical plates were slightly different among the four cases and theoretically the different screw arrangements would impact the biomechanical results. Careful consideration was needed for the tradeoff between plate length, bone safety and implant fixation stability, and plate length should be carefully designed to obtain reliable biomechanical properties. Tie et al. [Citation15] combined several types of mandibular defects and reconstruction methods with autogenous bone grafts and compared the biomechanics of mandibles reconstructed using free fibula and deep circumflex iliac artery flaps. In the aspect of investigating the biomechanics of the reconstructed mandibles, the stress distribution within each reconstructed mandibular models was only compared and studied by finite element analysis. As a matter of fact, the lack of comparison of displacement distribution might not fully reflect the differences of the biomechanics of the models. In addition, they suggested that the surgical plates were essential to reduce the effects of stress, while the surgical plates were not part of the research contents in the study. The present study investigated mandibular body defects, which are common in clinical practice. In contrast with the previous studies, FE models were established using free fibula and deep circumflex iliac artery flaps in combination with 3D-printed or conventional surgical plates for fixation. The remaining teeth were loaded with vertical bite forces to investigate the biomechanical behavior of the models.
We found that normal and reconstructed mandibles had similar displacement distributions, which continuously varied from posterior fixed and constrained parts, including bilateral mandibular condyles, coronoid processes, and angles, to the central incisors. The maximum total deformation occurred at mandibular incisors. Compared to the normal mandible, the difference in the maximum value for reconstructed mandibles was 0.0891–0.1145 mm. A comparison of stress distribution revealed that high stress areas in the mandible were concentrated at the lower edge of the body of the bilateral mandibular body, with the maximum equivalent Von Mises stress for the normal mandible on the right side and that for the reconstructed mandible on the left side. This may be because the stress trajectories change after reconstruction with autologous bone grafts. Compared to the normal mandible, the difference of the maximum equivalent Von Mises stress value for the reconstructed mandibles was 10.96–47.59 MPa. The biomechanical behavior of reconstructed mandibles was similar to the normal mandible under similar occlusal loads, indicating effective reconstruction with the four reconstruction and fixation methods.
The reconstructed mandibles fixed using the same type of surgical plates had similar maximum total deformation and maximum equivalent Von Mises stress values. The differences in the maximum total deformation values were 0.0176 and 0.0063 mm, while the differences in the maximum equivalent Von Mises stress values were 0.60 and 13.78 MPa for fixed with 3D-printed and conventional surgical plates, respectively. This showed that mandibles reconstructed using different autologous bone grafts but fixed using the same surgical plates had similar biomechanical behaviors and reconstruction outcomes.
A comprehensive comparison of the surgical plates showed that they had similar displacement distributions and that the maximum total deformation occurred in front of the anterior osteotomy line at the screw hole of the anterior segment of the surgical plates. However, stress distribution for the two surgical plates was dissimilar, with the maximum equivalent Von Mises stress near the osteotomy line. The maximum equivalent Von Mises stress for 3D-printed surgical plates occurred behind the posterior osteotomy line at the screw hole of the posterior segment of the surgical plate, while that for conventional surgical plates occurred in front of the anterior osteotomy line at the screw hole of the anterior segment. Mandibular body defect models reconstructed with the same autologous bone graft but fixed using different surgical plates had similar maximum total deformation values, while the difference between the maximum equivalent Von Mises stress values was small. For models reconstructed using free fibula and deep circumflex iliac artery flaps, the differences in maximum total deformation values were 0.0029 and 0.0272 mm, while the differences in maximum equivalent Von Mises stress values were 35.14 and 73.03 MPa, respectively. The maximum yield strengths for titanium and titanium alloy (Ti-6Al-4V) have been reported as 485 MPa and 795–875 MPa, respectively [Citation23,Citation24]. Since the maximum equivalent Von Mises stress values for surgical plates were below the maximum yield strengths for the corresponding materials, the two types of surgical plates had good safety and stability [Citation12]. These results indicate that the biomechanical behavior of 3D-printed surgical plates is similar to conventional surgical plates, and that they may be effective for the reconstruction of mandibular body defects.
In addition, the maximum equivalent Von Mises stress for 3D-printed surgical plates occurred behind the posterior osteotomy line of the posterior segment of the surgical plate, while that for conventional surgical plates occurred in front of the anterior osteotomy line of the anterior segment. The differences in the maximum equivalent Von Mises stress between the two kinds of surgical plates may be related to differences in raw materials and their properties. The 3D-printed surgical plates were made using titanium alloy (Ti-6Al-4V), which is commonly used as the raw material for patient-specific surgical plates, while the conventional surgical plates were made using pure titanium. The properties, including elastic modulus and Poisson’s ratio, of related materials were different. Stress concentration or fatigue in a part of the surgical plate leads to complications, such as surgical plate fracture [Citation4,Citation25]. In a retrospective study, Liu et al. [Citation26] demonstrated that most of the surgical plate fractures after mandibular defect reconstruction occurred at the junction of the bone graft and at the mandibular angle. It has been suggested that this may be related to the interruption of stress at the junction. In order to restore the cracked stress trajectories of the mandible, maintain the stability of the broken area and ensure the normal healing of bone, the corresponding parts of the surgical plate need to bear greater stress. As a result, the surgical plate would break or fail if it is exposed to or bears much higher stress in clinical application [Citation27], therefore, in practice, finite element analysis can be used to investigate the biomechanical behavior of the surgical plate and find out the sites of the surgical plate where the great stress occur and that need to be optimized for prevention. In this study, the differences in stress distribution of two kinds of surgical plates can provide important references for optimization. The conventional surgical plates, which are manufactured by the method of casting process, should be prebent manually in accordance with the reconstructed mandibular models before operation and the sites where the great stress occurs are impossible to be optimized by structural modification or improvement artificially in view of the processing technique. While, for the 3D-printed surgical plates, according to the results of finite element analysis before operation, the sites of the surgical plates where the great stress occurs can be designed into ideal and optimal structures in advance and directly printed into the patient specific surgical plates, which may perform better in biomechanical behavior and match the contour of the remaining mandible and bone graft well, for clinical application. Based on the current available results, specifically speaking, the surgical plate could be optimized by thickening at the sites where the maximum equivalent Von Mises stress occurs, i.e. areas over and adjacent to the osteotomies, or attenuating areas subject to less stress at the same time, which could improve the ability to bear higher tress [Citation27], or by extending the width of the surgical plate, increasing the plate and bone contact area, to reduce the stress [Citation19,Citation28], or by using an auxiliary whole or partial surgical plate at the areas over and adjacent to the osteotomies to reduce the stress distinctly and directly [Citation29].
Surgical plates showed higher maximum equivalent Von Mises stress values in mandibular reconstructions with deep circumflex iliac artery flaps compared to those with free fibula flaps. This may be because the mandible is a stress-bearing bone and its height and volume cannot be effectively restored using free fibula flaps. Meanwhile, deep circumflex iliac artery flaps have a configuration and structure that resembles the mandible and can three-dimensionally restore its contour, height, and volume. Mandibular reconstruction with the iliac crest also allows bite force transmission [Citation15]. Since surgical plates are generally fixed along the lower edge of the mandible, they can bear greater stresses resulting from occlusal forces if deep circumflex iliac artery flap reconstruction is performed.
In contrast with previous studies, the present study established a series of FE models for a comprehensive comparison of the biomechanical behavior of 3D-printed surgical plates used for different types of mandibular reconstructions. There are still some limitations of the present study. On the one hand, vertical occlusal forces were applied to the remaining teeth, while the major masticatory muscles were restrained. Future studies may use the activity of major masticatory muscles or lateral and horizontal occlusal forces to further analyze the displacement and Von Mises stress distributions. On the other hand, the mandibular body defect and conventional surgical plate, which are representative and common in clinical practice, were mainly investigated in the study. Therefore, there will be a supplement of different types of mandibular defects and 3D-printed surgical plates with different dimensions and structures and more volunteers involved in the research in future work to systematically and comprehensively explore the biomechanical behavior of 3D-printed surgical plates and provide useful references for their clinical applications.
5. Conclusions
The biomechanical behaviors of 3D-printed and conventional surgical plates used for mandibular reconstruction were assessed in detail with the method of finite element analysis simulations. It could be found that the 3D-printed surgical plates shared similar biomechanical behavior with the conventional surgical plates in displacement distributions and Von Mises stress distributions under the same experimental conditions–the maximum total deformation occurred in front of the anterior osteotomy line at the anterior segment of the surgical plates, and the maximum equivalent Von Mises stress occurred near the osteotomy lines of the surgical plates. This indicated that 3D-printed surgical plates could be effectively applied to the mandibular body defect reconstructions and could provide internal rigid fixation for the remaining mandible and bone graft. The appropriate management, especially the thickening or extending the width of the surgical plate, or adopting auxiliary fixation, of the sites over and adjacent to the osteotomy lines in the surgical plate could improve the ability to bear higher tress or reduce the local stress and may provide secure and stable fixation.
Ethical approval
The study protocol was approved by the ethical committee of Peking University School and Hospital of Stomatology (PKUSSIRB-202162016) and was performed in accordance with the ethical standards laid down in the 1964 Declaration of Helsinki and its later amendments.
Acknowledgements
We appreciate the Chuangyan Digital Technology Company (Nantong, Jiangsu, China) for the technical and software support of this research.
Disclosure statement
No potential conflict of interest was reported by the author(s).
Additional information
Funding
References
- Azuma M, Yanagawa T, Ishibashi-Kanno N, et al. Mandibular reconstruction using plates prebent to fit rapid prototyping 3-dimensional printing models ameliorates contour deformity. Head Face Med. 2014;10(1):1. doi: 10.1186/1746-160X-10-45.
- Zhang WB, Yu Y, Wang Y, et al. Improving the accuracy of mandibular reconstruction with vascularized iliac crest flap: role of computer-assisted techniques. J Craniomaxillofac Surg. 2016;44(11):1819–13. doi: 10.1016/j.jcms.2016.08.014.
- Katakura A, Shibahara T, Noma H, et al. Material analysis of AO plate fracture cases. J Oral Maxillofac Surg. 2004;62(3):348–352. doi: 10.1016/j.joms.2003.05.009.
- Martola M, Lindqvist C, Hänninen H, et al. Fracture of titanium plates used for mandibular reconstruction following ablative tumor surgery. J Biomed Mater Res B Appl Biomater. 2007;80(2):345–352. doi: 10.1002/jbm.b.30603.
- Rana M, Chin SJ, Muecke T, et al. Increasing the accuracy of mandibular reconstruction with free fibula flaps using functionalized selective laser-melted patient-specific implants: a retrospective multicenter analysis. J Craniomaxillofac Surg. 2017;45(8):1212–1219. doi: 10.1016/j.jcms.2017.04.003.
- Yang W F, Choi WS, Leung YY, et al. Three-dimensional printing of patient-specific surgical plates in head and neck reconstruction: a prospective pilot study. Oral Oncol. 2018;78(12):31–36. doi: 10.1016/j.oraloncology.2018.01.005.
- Yang WF, Zhang CY, Choi WS, et al. A novel ‘surgeon-dominated’ approach to the design of 3D-printed patient-specific surgical plates in mandibular reconstruction: a proof-of-concept study. Int J Oral Maxillofac Surg. 2020;49(1):13–21. doi: 10.1016/j.ijom.2019.05.005.
- Du R, Su YX, Yan Y, et al. A systematic approach for making 3D-printed patient-specific implants for craniomaxillofacial reconstruction. Engineering. 2020;6(11):1291–1301. doi: 10.1016/j.eng.2020.02.019.
- Wang CF, Yu Y, Bai W, et al. Mechanical properties of three-dimensionally printed titanium plates used in jaw reconstruction: preliminary study. Int J Oral Maxillofac Surg. 2022;51(6):754–761. doi: 10.1016/j.ijom.2021.09.008.
- Boyd JB, Gullane PJ, Rotstein LE, et al. Classification of mandibular defects. Plast Reconstr Surg. 1993;92(7):1266–1275.
- Antony AK, Chen WF, Kolokythas A, et al. Use of virtual surgery and stereolithography-guided osteotomy for mandibular reconstruction with the free fibula. Plast Reconstr Surg. 2011;128(5):1080–1084. doi: 10.1097/PRS.0b013e31822b6723.
- Zhong S, Shi Q, Sun Y, et al. Biomechanical comparison of locking and non-locking patient-specific mandibular reconstruction plate using finite element analysis. J Mech Behav Biomed Mater. 2021;124(9):104849. doi: 10.1016/j.jmbbm.2021.104849.
- Park SM, Lee JW, Noh G. Which plate results in better stability after segmental mandibular resection and fibula free flap reconstruction? Biomechanical analysis. Oral Surg Oral Med Oral Pathol Oral Radiol. 2018;126(5):380–389. doi: 10.1016/j.oooo.2018.05.048.
- Savoldelli C, Bouchard PO, Loudad R, et al. Stress distribution in the temporo-mandibular joint discs during jaw closing: a high-resolution three-dimensional finite-element model analysis. Surg Radiol Anat. 2012;34(5):405–413. doi: 10.1007/s00276-011-0917-4.
- Tie Y, Wang DM, Ji T, et al. Three-dimensional finite-element analysis investigating the biomechanical effects of human mandibular reconstruction with autogenous bone grafts. J Craniomaxillofac Surg. 2006;34(5):290–298. doi: 10.1016/j.jcms.2006.03.004.
- Nagasao T, Kobayashi M, Tsuchiya Y, et al. Finite element analysis of the stresses around fixtures in various reconstructed mandibular models – Part II (effect of horizontal load). J Craniomaxillofac Surg. 2003;31(3):168–175. doi: 10.1016/S1010-5182(03)00029-5.
- Van Eijden TMGJ. Biomechanics of the mandible. Crit Rev Oral Biol Med. 2000;11(1):123–136. doi: 10.1177/10454411000110010101.
- Bujtár P, Simonovics J, Váradi K, et al. The biomechanical aspects of reconstruction for segmental defects of the mandible: a finite element study to assess the optimisation of plate and screw factors. J Craniomaxillofac Surg. 2014;42(6):855–862. doi: 10.1016/j.jcms.2013.12.005.
- Knoll WD, Gaida A, Maurer P. Analysis of mechanical stress in reconstruction plates for bridging mandibular angle defects. J Craniomaxillofac Surg. 2006;34(4):201–209. doi: 10.1016/j.jcms.2006.01.004.
- Zhong S, Shi Q, Van Dessel J, et al. Biomechanical validation of structural optimized patient-specific mandibular reconstruction plate orienting additive manufacturing. Comput Methods Programs Biomed. 2022;224:107023. doi: 10.1016/j.cmpb.2022.107023.
- Cheng K J, Liu Y F, Wang JH, et al. Biomechanical behavior of mandibles reconstructed with fibular grafts at different vertical positions using finite element method. J Plast Reconstr Aesthet Surg. 2019;72(2):281–289. doi: 10.1016/j.bjps.2018.10.002.
- Shi Q, Sun Y, Yang S, et al. Preclinical study of additive manufactured plates with shortened lengths for complete mandible reconstruction: design, biomechanics simulation, and fixation stability assessment. Comput Biol Med. 2021;139:105008. doi: 10.1016/j.compbiomed.2021.105008.
- Geetha M, Singh AK, Asokamani R, et al. Ti based biomaterials, the ultimate choice for orthopaedic implants – A review. Prog Mater Sci. 2009;54(3):397–425. doi: 10.1016/j.pmatsci.2008.06.004.
- Mitsuo N. Mechanical properties of biomedical titanium alloys. Mater Sci Eng A. 1998;243(1–2):231–236.
- Shi Q, Sun Y, Yang S, et al. Failure analysis of an in-vivo fractured patient-specific Ti6Al4V mandible reconstruction plate fabricated by selective laser melting. Eng Fail Anal. 2021;124(3):105353. doi: 10.1016/j.engfailanal.2021.105353.
- Liu SP, Cai ZG, Zhang J, et al. Plate related complication after mandibular reconstruction. Chin J Stomatol. 2013;48(10):586–590.
- Li P, Shen L, Li J, et al. Optimal design of an individual endoprosthesis for the reconstruction of extensive mandibular defects with finite element analysis. J Craniomaxillofac Surg. 2014;42(1):73–78. doi: 10.1016/j.jcms.2013.02.005.
- Gutwald R, Jaeger R, Lambers FM. Customized mandibular reconstruction plates improve mechanical performance in a mandibular reconstruction model. Comput Methods Biomech Biomed Engin. 2017;20(4):426–435. doi: 10.1080/10255842.2016.1240788.
- Hoefert S, Taier R. Mechanical stress in plates for bridging reconstruction mandibular defects and purposes of double plate reinforcement. J Craniomaxillofac Surg. 2018;46(5):785–794. doi: 10.1016/j.jcms.2018.01.016.