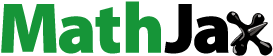
ABSTRACT
A predictive model was developed to determine the combined effect of salinity and pH on sediments enrichment of heavy metals; (EF) of Zn, Pb, Cu, and Ni in Uppanar estuary, India. Data covered four seasons of 2008–2009 and 2009–2010, and corresponded to fivestations scattered along Uppanar estuary. Salinity, pH, EFZn, EFPb, EFCu and EFNi were 17.85 ± 9.46, 7.62 ± 0.35, 9.51 ± 2.01, 3.54 ± 1.96, 10.85 ± 1.97, and 0.70 ± 0.20, respectively. For each metal, we fitted a regression model with EF as response, and the interaction between salinity and pH as predictor. We found that the interaction of salinity and pH predicts EFPb (F3,36 = 9.03, P < 0.001) and EFNi (F3,36 = 3.22, P = 0.034). Specifically, salinity and pH were positively associated with EFPb and negatively associated with EFNi. We found no significant effect of salinity and pH interaction on EFZn (F3,36 = 2.56, P = 0.069) and EFCu (F3,36 = 1.68, P = 0.188). We conclude that a joint effect of salinity and pH on EF varies with heavy metals species, and when significant, takes a different pattern depending on the predominant geochemical phase and source of heavy metals
1. Introduction
Estuaries continue to be in the throes of heavy metals contamination from human activity such as agriculture, industry, fish farming, tourism and coastal settlings (Alloway, Citation2013; Simcock Lead Member et al., Citation2016). They can sink beyond-permissible amount of heavy metals due to the high capacity of sediments to absorb heavy metals (R. Chester, Citation1990). However, this interaction of sediments and heavy metals is not a static relationship (ibid.). It is subject to a number of both disrupting and enhancing factors including physical events (e.g., tidal currents, hydrostatic pressure, and atmospheric deposition), prevailing conditions (e.g., salinity, temperature, pH, redox potential, and microbial activity), and anthropogenic influence (e.g., waste discharge) (Chapman and Wang, Citation2001; R. Chester, Citation1990; C. Zhang et al., Citation2014). In the current paper, we investigate the synergic influence of water column conditions on heavy metals enrichment of sediments in Uppanar estuary, India.
Among estuarine water conditions, salinity varies pointedly and appears as an important drive of geochemical change in estuarine sediments (Chatterjee et al. Citation2013). Typically, estuarine salinity increases seaward and reaches its highest levels in the periods of maximum tides bringing significant volume of marine water into fluvial section of the estuary (Dalrymple and Choi Citation2007; Kjerfve Citation1994). Salinity concentrations rise also during summer season with increased levels of evaporation in the estuary, and become lowest during wetter seasons when rainfall inflow dominate evaporation outflow (US Department of Commerce, National Oceanic and Atmospheric Administration, Citation2017). This fluctuation of salinity concentration impacts chemical reactions in the water column (Paalman et al., Citation1994). More specifically, salt-derived cations compete with positively charged heavy metals species for sorption sites on the solid phase of the sediments, and/or associate with negative electrolytes dissolved in overlying or interstitial water (ibid.). Several studies related the increase of salinity with the rise of bioavailability and mobility of heavy metals such as Cadmium (Cd), Manganese (Mn), Iron (Fe), Copper (Cu) and Lead (Pb) in estuarine sediments (Hatje et al., Citation2003; Du Laing et al., Citation2008; Zhao et al., Citation2013). However, no study explored the extent to which this effect of salinity on heavy metals mobility is moderated by other co-prevailing water conditions such as pH.
pH is an important parameter that influences the solubility of heavy metals in estuarine water (Gäbler, Citation1997; Y. Zhang et al., Citation2018). Estuarine pH fluctuates with the type and amount of dissolved minerals, dissolved carbon dioxide, atmospheric deposition, waste, turbulence, water flow, and microorganisms’physiology such as respiration and photosynthesis (R. Chester, Citation1990; Roy Chester, Citation2009; Feely et al., Citation2010; Venkatramanan et al. Citation2015). Estuarine pH levels range from 7.0 to 7.5 in upstream sections, to between 8.0 and 8.6 in downstream areas (Hossain & Marshall, Citation2014; Ohrel & Register, Citation2006). Alkalinity of estuarine water is largely due to the elevated amount of carbonate and bicarbonate dissolved in water (Schroeder, Citation2003). A number of studies looked at the effect of pH on heavy metals release, and toxicity (Hong et al., Citation2011; Riba et al., Citation2004; Y. Zhang et al., Citation2018). An increase or decrease in pH has been related to the retention or release of heavy metals, hence their bioavailability and distribution in overlying water (Hatje et al., Citation2003; Hong et al., Citation2011; Van Assche, Citation2006) . High pH values are associated with adsorption and precipitation of heavy metals (Boekhold et al., Citation1993; Evans, Citation1989). In contrast, low pH is proven to promote the release of heavy metals by sediments (C. Zhang et al., Citation2014). However, it remains unclear whether the effect of pH on the affinity of sediments to heavy metals is independent or dependent from the similar effect of salinity.
To our knowledge, the current study is the first to investigate the combined influence of salinity and pH on enrichment factor (EF) of Zn, Pb, Cu, and Ni in estuarine sediments of Uppanar, India. EF approach is electively used to sense both the levels and origin of heavy metals in sediments (Gałuszka & Migaszewski, Citation2011; Reimann & de Caritat, Citation2005). In fact, Uppanar estuary harbors intensive fish farming and receives constant water runoff from surrounding intensive agriculture, ever growing industry, and human settlings (Gopal et al., Citation2018; Sheeba, Citation2016; Sulieman & El Amin, Citation2019). Therefore, there is a local need of measuring and understanding the fate of anthropogenic heavy metals for an improved awareness and a better management of heavy metals.
2. Methods
2.1. Study Data
Data were obtained from Sheeba (Citation2016). They cover a period of two years (2008–2009 and 2009–2010) and four seasons (Monsoon, Post Monsoon, Summer, and Premonsoon) of each year. They include salinity, pH, and EF. Briefly, salinity and pH of sediments were respectively obtained from refractometer and pH meter measurements. For salinity measurement; a sediment sample was saturated with distilled water, shaken, filtered, and applied on refractometer. Whereas, for pH measurement, a sediment sample was added with distilled water and shaken for 1 h before applying pH meter. Heavy metals were sequentially extracted from sediments following the method of Tessier et al. (Citation1979).
EF values were obtained using the following equation:
Where and
are the analyzed heavy metal concentrations in the sediment sample and the background reference, respectively;
and
are Fe concentrations in the sample and background reference material, respectively.
The enrichment factor of heavy metals can be differentiated, whether it is from natural or anthropogenic origin. EF value below one reflects a natural origin of the heavy metal while EF value above one indicates enrichment due to anthropogenic input (Gałuszka & Migaszewski, Citation2011).
2.2. Data Analysis
For data description, we expressed pH, salinity, EFZn, EFPb, EFCu, and EFNi values in mean ± standard deviation. We further indicated whether they differed across the year, stations, and seasons by applying Mann-Whitney U-test and Kruskal-Wallis H-test based on their rationale and assumptions (Banks et al., Citation2005; Madeline Levitan, Citation2014; Siegel & Castellan, Citation1988).
For inferential analysis, we used multiple regression models to investigate the combined effect of pH and salinity on enrichment factor of Zn, Pb, Cu, and Ni. Multiple regression model estimates a linear relationship between two or more variables by using the general equation:
Whereby Yi denotes the response variable,
Xi and Wi are predictor variables,
is an intercept, and
is a residual (error).
For each heavy metal, we used R function “lm” to fit a corresponding model with enrichment factor (EF) as response variable, and the interaction between pH and salinity as predictor variable. Therefore, the model fitted data to the following linear equation:
Whereby Yi is the response variable, Xi Wi is an interaction term of predictor variables, and is an intercept, and
is a residual.
We then checked the assumptions of normally distributed and homogeneous residuals of each model by visually inspecting scatter plot of the residuals plotted against fitted values. We found no obvious deviation from these assumptions. We further checked the stability of each model by calculating and comparing maximum dffits and Cook’s distance against the respective thresholds of 2 and 1 (Field, Citation2005; Quinn & Keough, Citation2002). We found maximum dffits of 0.985, 1.041, 0.949, and 0.956 for Zn, Pb, Cu and Ni model respectively. The respective maximum Cook’s distance was 0.22, 0.232, 0.206, and 0.209. To test the significant effect of the predictor on the response, we compared the fit of the full model with that of the null model comprising only the intercept (Forstmeier & Schielzeth, Citation2011). We computed this comparison by using R function “ANOVA” based on F-test approach (Bishwal, Citation2017). In fact, this function calculates residual sum of squares of both full and null models by respectively using the following formulas:
Whereby Yi is the observed response and i is the fitted response for the full model.
Whereby Yi is the observed response and is the fitted response for the null model.
Then, the function calculates F-statistic as follows:
Where Df (N) and Df (F) denote the respective degrees of freedom associated with null and full models.
When F-statistic was large or its associated p-value was small (i.e., below 0.05), we rejected the null hypothesis that the null model is correct.
All calculations were performed in R version 3.6.3.
3. Results
3.1. Descriptive Statistics
Salinity, pH, EFZn, EFPb, EFCu and EFNi were 17.85 ± 9.46, 7.62 ± 0.35, 9.51 ± 2.01, 3.54 ± 1.96, 10.85 ± 1.97, and 0.70 ± 0.20, respectively. Salinity and pH did not change significantly in 2009–2010 as compared to 2008–2009, remained stable across seasons, but varied significantly between stations (, ). EFPb, EFCu and EFNi did not change in 2009–2010 as compared to 2008–2009 but showed significant variation across seasons and stations (, &). EFZn showed a significant seasonal trend but did not vary between 2008–2009 and 2009–2010, and stayed similar amongst stations ().
Figure 2. Variation of enrichment factor of Zinc (A), Lead (B), Copper (C) and Nickel (D) across seasons (MN: Monsoon; POM: Postmonsoon; PRM: Premonsoon; SUM: Summer).
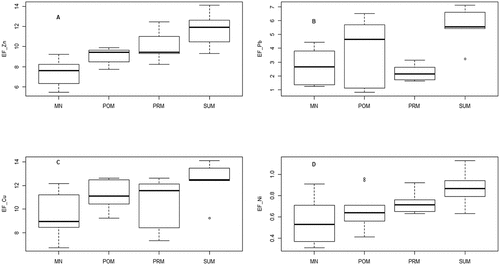
Table 1. Data characteristics across the study period, seasons and stations.
3.2. Combined Effect of Salinity and pH on Enrichment Factor of Heavy Metals
The comparison of full model against null model revealed a significant effect of salinity and pH interaction on enrichment factor of lead and nickel (). More specifically, an increase of salinity and pH was associated with high values of lead enrichment factor (). In contrast, high levels of nickel enrichment factor were related to the low levels of salinity and pH (). There was no significant effect of salinity and pH on zinc and copper enrichment factors.
Table 2. Output of full versus null model comparison.
4. Discussion
The multiple regression analysis conducted here estimated the combined effect of salinity and pH on enrichment of Zn, Pb, Cu, and Ni in estuarine sediments. It revealed a significant effect of salinity and pH on enrichment of lead () and Nickel (
). However, it showed no significant effect of salinity and pH on enrichment of zinc (
) and Copper
.
The pattern of increasing with salinity and pH may be explained as follows. First, the high pH levels are favorable to the formation and stability of links between heavy metals and sediments (C. Zhang et al., Citation2014) . These links are metallic, organic and/or substitution bonds, which lead to physical occurrence of adsorption, complexation, and sedimentation (Appel and Ma, Citation2002). In contrast, the pH change toward acidity breaks these bonds and leads to the release, dissolution and redistribution of heavy metals(Gäbler, Citation1997). Second, an increase of
along with salinity suggests an important anthropogenic portion of Pb that gets fixed in sediments as salinity and pH conditions become favorable (Zhao et al., Citation2013). Third, high salinity acts in synergy with high pH by presumably inhibiting enzymes that would digest biogenic compounds of sediments to release heavy metals including Pb (Cortés-Lorenzo et al., Citation2012). In fact, salinity creates osmotic pressure and induces metabolic change that cause plasmolysis of bacteria that produce enzymes (e.g., aminopeptidases and glucosidases) involved in biogeochemical cycles (Fabiano & Danovaro, Citation1998; Poremba, Citation2006). Fourth, a gradual increase of salinity and pH occur seaward along estuary mixing zone whereby denser seawater mixes upward with light freshwater and generates forces that flocculate iron oxides, humic substances, and other colloidal particles such as clays which are deposited with absorbed heavy metals, for instance, Lead (R. Chester, Citation1990). Fifth, an increase of salinity with
increases can indicate that there might be a high volume of Pb ejected in Uppanar estuary so that the cations created in high salinity condition can’t fully compete the adsorption of Pb on sediments. Uppanar estuary is adjacent to highly industrialized and urbanized region of Cuddalore, Tamil Nadu. Moreover, the estuary receives water runoff from various regions of Tamil Nadu, one of the biggest reserves of Lead in India. Lead resources in Tamil Nadu and the whole country were estimated respectively to 790 and 13,003.7 million tonin 2015 (Indian Bureau of Mines, Citation2020). Yet, in the last 10 years (2009–2019), the country imported about 19,111.2 ton per year to suffice its industrial demand (ibid.). These figures point out how important was (and is) the potential risk of Pb enrichment in the study period and areas.
The analysis of under joint effect of salinity and pH value showed a reverse pattern as compared to
. This was likely due to the difference in the predominant source and geochemical phase of Ni and Pb in the study area. In fact,
(as opposed to
) remained below one, indicating a very low degree of sediments contamination by Ni. Therefore, the major source of Ni was natural rather than anthropogenic (Venkatramanan et al., Citation2018). Furthermore, we deduce that a great amount of Ni was in residual phase, linked to the sediment matrix. Residual phase is obtained after digestion in a strong acid to leach out recalcitrant metals at the very last stage of 5-step Tessier et al. (Citation1979) sequential extraction method. It gives an estimation of long-term potential risk of the heavy metal when conditions change. Like here, we found variation of
with salinity and pH, pointing out dynamism of Ni mobility from weakly bound phases; exchangeable (soluble species, cation exchange sites, and linked to carbonates), reducible (linked to manganese oxides, and Iron oxides as Pentlandite), and oxidisable (linked to sulphides as Millerite, and organic compound) (Cao et al., Citation2015; C. Zhang et al., Citation2014). Exchangeable phase represents the portion of Nickel bound to the sediments that can be easily replaced by neutral salts (Rauret, Citation1998). The portion bound to carbonates is released as pH decreases depending on the size and amount of carbonate compounds (Gleyzes et al., Citation2002). Manganese and Iron oxides phases are considered as heavy metals sink. Mn and Fe oxides form chemical bonds with heavy metals by gaining their electrons. Oxidisable phase occurs when heavy metals get associated with organic matter and release them during oxidizing conditions and degradation of the latter (Bastami et al., Citation2017). All these weakly bound phases originate from anthropogenic input which may be attributed to permanent and casual runoff from adjacent industries manufacturing mainly stainless metal objects. A decrease of pH values breaks heavy metals association with estuarine sediments and result in the increase of heavy metals mobility. When pH drop the sediment would increase in the heavy metals concentration (Simpson et al., Citation2004). Both, low values of salinity and pH surge the release of heavy metals from sediments to water particularly Zn (Riba et al., Citation2003). Along the salinity gradient, the enrichment of Ni increased seaward, exhibiting a clear value maximum in the bay. Pearson’s correlation and principal components analysis(PCA) revealed that
is positively associated with the salinity gradient in the sediments of the bay zone (S. Koukina & Lobus, Citation2019; S.E. Koukina & Lobus, Citation2020). The pattern of Nickel enrichment (
) at low salinity and pH values suggest lithogenic origin. In the course of study period, the enrichment factor (EF) value for Ni was
less than one indicating that the poorly enriched in the estuarine sediments and substantiates that Ni is not influenced by anthropogenic inputs (Sheeba, Citation2016).
Lastly, we found no significant effect of salinity and pH interaction on enrichment of Zinc () and Copper
. It appears that the dissolved proportion of Zinc and Copper were too high and could not be significantly displaced and mobilized in increasing pH and salinity conditions. While the great portion of dissolved Copper is likely to originate from metal mining and /or industrial areas, a large portion of dissolved Zinc comes from air deposition (Ahumada et al., Citation2007; Bakary et al., Citation2015; Campos et al., Citation1998; Moore & Ramamoorthy, Citation1984a; Neff, Citation2002). In oxidizing conditions, both Zinc and Copper have high affinity to organic matter, with Copper exhibiting higher selectivity and stability for organic ligand complexation than Zinc (Ferrer et al., Citation2003; Moore & Ramamoorthy, Citation1984; Ruhong et al., Citation2010). In reducing conditions, Zinc and Copper are adsorbed to amorphous iron and manganese oxides. It’s more likely that Zinc and Copper are rapidly occurring in each of above-mentioned phases due to wide sources and high level of availability of both metals. The non-significant effect of salinity and pH on enrichment of zinc (
) and copper
suggested that Zn and Cu are more conservative due to their highest amount in residual fraction. It is well known that heavy metals in the residual fraction are bound to crystal lattices and cannot easily release (Devi & Bhattacharyya, Citation2018; Wijaya et al., Citation2019).
Conclusion
In conclusion, the effect of salinity and pH interaction on Pb enrichment is primarily explained by ionic exchange induced by varying salinity and pH and also by a stable fixation of anthropogenic Pb on solid phase of sediments. In contrast, the bonds between Ni and sediments are weak and easily disrupted by high salinity and pH gradient. Lastly, we could not detect a significant change on EF of Cu and Zn due to potential large amount or crystal bounds of the latter in the sediments. Future studies should model heavy metals remobilization from estuarine sediments under other coexisting environmental factors.
Acknowledgments
This work would not have been possible without genuine collaboration with the research group led by T. Ramkumar, Professor and Director i/c Centre for International Relations at Annamalai University, India. Rumuri is deeply indebted to his family for their warm-hearted support throughout the whole research work.
Disclosure statement
The authors declare that they have no known competing financial interests or personal relationships which have, or could be perceived to have, influenced the work reported in this article.
References
- Ahumada, R., González, E., Medina, V., & Rudolph, A. (2007). Analysis of mayor zinc sources in marine sediments of the estuarine zone in Aysén Fjord, Chile. Investigaciones Marinas, 35(2), 5–14. https://doi.org/10.4067/S0717-71782007000200001
- Alloway, B. J. (2013). Sources of heavy metals and metalloids in soils. In: B. J. Alloway (Ed.), Heavy Metals in Soils (pp. 11–50). Springer. https://doi.org/10.1007/978-94-007-4470-7_2
- Appel, C., & Ma, L. (2002). Concentration, pH, and Surface Charge Effects on Cadmium and Lead Sorption in Three Tropical Soils. Journal of Environment Quality, 31(2), 581–589. https://doi.org/10.2134/jeq2002.0581
- Bakary, I., Yao, K. M., Etchian, O. A., Soro, M. B., Trokourey, A., & Bokra, Y. (2015). Zinc, copper, cadmium, and lead concentrations in water, sediment, and Anadara senilis in a tropical estuary. Environmental Monitoring and Assessment, 187(12), 1–11. https://doi.org/10.1007/s10661-015-4976-6
- Banks, D., Morland, G., & Frengstad, B. (2005). Use of non-parametric statistics as a tool for the hydraulic and hydrogeochemical characterization of hard rock aquifers. Scottish Journal of Geology, 41(1), 69–79. https://doi.org/10.1144/sjg41010069
- Bastami, K. D., Neyestani, M. R., Esmaeilzadeh, M., Haghparast, S., Alavi, C., Fathi, S., Nourbakhsh, S., Shirzadi, E. A., & Parhizgar, R. (2017). Geochemical speciation, bioavailability and source identification of selected metals in surface sediments of the Southern Caspian Sea. Marine Pollution Bulletin, 114(2), 1014–1023. https://doi.org/10.1016/j.marpolbul.2016.11.025
- Bishwal, R. M., 2017. Potential use of R-statistical programming in the field of geoscience, In: 2017 2nd International Conference for Convergence in Technology, I2CT 2017, (pp. 979–982). Mumbai, India: Institute of Electrical and Electronics Engineers Inc., https://doi.org/10.1109/I2CT.2017.8226275
- Boekhold, A. E., Temminghoff, E. J. M., & Van Der Zee, S. E. A. T. M. (1993). Influence of electrolyte composition and pH on cadmium sorption by an acid sandy soil. Journal of Soil Science, 44(1), 85–96. https://doi.org/10.1111/j.1365-2389.1993.tb00436.x
- Campos, E., Barahona, E., Lachica, M., & Mingorance, M. D. (1998). A study of the analytical parameters important for the sequential extraction procedure using microwave heating for Pb, Zn and Cu in calcareous soils. Analytica Chimica Acta, 369(3), 235-243. http://doi.org/10.1016/S0003-2670(98)00238-4
- Cao, L., Tian, H., Yang, J., Shi, P., Lou, Q., Waxi, L., Ni, Z., & Peng, X. (2015). Multivariate Analyses and Evaluation of Heavy Metals by Chemometric BCR Sequential Extraction Method in Surface Sediments from Lingdingyang Bay, South China. Sustainability, 7(5), 4938–4951. https://doi.org/10.3390/su7054938
- Chapman, P. M., & Wang, F. (2001). Assessing sediment contamination in estuaries. Environmental Toxicology and Chemistry,20(1), 3–22. http://www.ncbi.nlm.nih.gov/pubmed/11351413
- Chatterjee, M., Shankar, D., Sen, G. K., Sanyal, P., Sundar, D., Michael, G. S., Chatterjee, A., Amol, P., Mukherjee, D., Suprit, K., Mukherjee, A., Vijith, V., Chatterjee, S., Basu, A., Das, M., Chakraborti, S., Kalla, A., Misra, S. K., Mukhopadhyay, S.… Sarkar, K. (2013). Tidal variations in the sundarbans estuarine system, India. Journal of Earth System Science,122(4), 899–933. https://doi.org/10.1007/s12040-013-0314–y
- Chester, R. (1990). The transport of material to the oceans: The river pathway. In: R. Chester (Ed.), Marine Geochemistry (pp. 14–82). Netherlands: Springer. https://doi.org/10.1007/978-94-010-9488-7_3
- Chester, R. (2009). Marine Geochemistry. John Wiley & Sons.
- Cortés-Lorenzo, C., Rodríguez-Díaz, M., López-Lopez, C., Sánchez-Peinado, M., Rodelas, B., & González-López, J. (2012). Effect of salinity on enzymatic activities in a submerged fixed bed biofilm reactor for municipal sewage treatment. Bioresource Technology, 121(2012), 312–319. https://doi.org/10.1016/j.biortech.2012.06.083
- Dalrymple, R. W., & Choi, K. (2007). Morphologic and facies trends through the fluvial–marine transition in tide-dominated depositional systems: A schematic framework for environmental and sequence-stratigraphic interpretation. Earth-Science Reviews, 81(3–4), 135–174. https://doi.org/10.1016/j.earscirev.2006.10.002
- Devi, U., & Bhattacharyya, K. G. (2018). Mobility and bioavailability of Cd, Co, Cr, Cu, Mn and Zn in surface runoff sediments in the urban catchment area of Guwahati, India. Applied Water Science, 8(1), 18. https://doi.org/10.1007/s13201-018-0651-8
- Du Laing, G., De Vos, R., Vandecasteele, B., Lesage, E., Tack, F. M. G., & Verloo, M. G. (2008). Effect of salinity on heavy metal mobility and availability in intertidal sediments of the Scheldt estuary. Estuarine, Coastal and Shelf Science, 77(4), 589–602. https://doi.org/10.1016/j.ecss.2007.10.017
- Evans, L. J. (1989). Chemistry of metal retention by soils. Environmental Science & Technology, 23(9), 1046–1056. https://doi.org/10.1021/es00067a001
- Fabiano, M., & Danovaro, R. (1998). Enzymatic activity, bacterial distribution, and organic matter composition in sediments of the Ross Sea (Antarctica). Applied and Environmental Microbiology, 64(10), 3838–3845. https://doi.org/10.1128/AEM.64.10.3838-3845.1998
- Feely, R. A., Alin, S. R., Newton, J., Sabine, C. L., Warner, M., Devol, A., Krembs, C., & Maloy, C. (2010). The Combined Effects of Ocean Acidification, Mixing, and Respiration on PH and Carbonate Saturation in an Urbanized Estuary. Estuarine, Coastal and Shelf Science, 88(4), 442–449. https://doi.org/10.1016/j.ecss.2010.05.004
- Ferrer, L. D., Andrade, J. S., Contardi, E. T., Asteasuain, R. O., & Marcovecchio, J. E. (2003). Copper and zinc concentrations in Bahía Blanca Estuary (Argentina), and their acute lethal effects on larvae of the crab Chasmagnathus granulata. Chemical Speciation & Bioavailability, 15(1), 7–14. https://doi.org/10.3184/095422903782775271
- Field, A. (2005). Discovering statistics using SPSS. SAGE Publications.
- Forstmeier, W., & Schielzeth, H. (2011). Cryptic multiple hypotheses testing in linear models: Overestimated effect sizes and the winner’s curse. Behavioral Ecology and Sociobiology, 65(1), 47–55. https://doi.org/10.1007/s00265-010-1038-5
- Gäbler, H. E. (1997). Mobility of heavy metals as a function of pH of samples from an overbank sediment profile contaminated by mining activities. Journal of Geochemical Exploration, 58(2–3), 185–194. https://doi.org/10.1016/S0375-6742(96)00061-1
- Gałuszka, A., & Migaszewski, Z. (2011). Geochemical background - an environmental perspective, in: Mineralogia. Sciendo, 7–17. https://doi.org/10.2478/v10002-011-0002-y
- Gleyzes, C., Tellier, S., & Astruc, M. (2002). Fractionation studies of trace elements in contaminated soils and sediments: A review of sequential extraction procedures. TrAC Trends in Analytical Chemistry, 21(6–7), 451–467. https://doi.org/10.1016/S0165-9936(02)00603-9
- Gopal, V., Nithya, B., Magesh, N. S., & Jayaprakash, M. (2018). Seasonal Variations and Environmental Risk Assessment of Trace Elements in the Sediments of Uppanar River Estuary, Southern India. Marine Pollution Bulletin, 129(1), 347–356. https://doi.org/10.1016/j.marpolbul.2018.03.003
- Hatje, V., Payne, T. E., Hill, D. M., McOrist, G., Birch, G. F., & Szymczak, R. (2003). Kinetics of trace element uptake and release by particles in estuarine waters: Effects of pH, salinity, and particle loading. Environment International, 29(5), 619–629. https://doi.org/10.1016/S0160-4120(03)00049-7
- Hong, Y. S., Kinney, K. A., & Reible, D. D. (2011). Effects of cyclic changes in pH and salinity on metals release from sediments. Environmental Toxicology and Chemistry, 30(8), 1775–1784. https://doi.org/10.1002/etc.584
- Hossain, M. B., & Marshall, D. J. (2014). Benthic infaunal community structuring in an acidified tropical estuarine system. . Aquatic Biosystems, 10(1), 11. https://doi.org/10.1186/2046-9063-10-11
- Indian Bureau of Mines. (2020). Indian Minerals Yearbook 2019 (Part-II:Metals and Alloys). (58th.).
- Kjerfve, B. (1994). Coastal Lagoons. In: Kjerfve (Ed.), Elsevier Oceanography Series (pp. 1–8). Elsevier. https://doi.org/10.1016/S0422–9894(08)70006–0
- Koukina, S., & Lobus, N. (2019). ajor and Trace Element Distribution in Suspended Particulate Matter and Sediments of the Tropical River Estuary (South Vietnam). In: H. Chaminé , M. Barbieri, O. Kisi, M. Chen, B. Merkel (Eds), Advances in Sustainable and Environmental Hydrology, Hydrogeology, Hydrochemistry and Water Resources (pp. 65-67). Springer. https://doi.org/10.1007/978-3-030-01572-5_16
- Koukina, S. E., & Lobus, N. V. (2020). Relationship between enrichment, toxicity, and chemical bioavailability of heavy metals in sediments of the Cai River estuary. Environmental Monitoring and Assessment, 192(5), 1–19. https://doi.org/10.1007/s10661-020-08282-6
- Madeline Levitan, D. (2014). Statistical Analysis of the Environmental Geochemistry of an Unmined Uranium Ore Deposit. [Doctoral dissertation, Virginia Polytechnic Institute and State University]. VTechWorks.
- Moore, J. W., & Ramamoorthy, S. (1984). Copper. In: J.W. Moore & S. Ramamoorthy (Eds). Heavy Metals in Natural Waters (pp. 77-99). Springer. https://doi.org/10.1007/978-1-4612-5210-8_5
- Moore J.W. & Ramamoorthy S. (1984). Zinc. In: J.W. Moore & S. Ramamoorthy (Eds). Heavy Metals in Natural Waters (pp. 182-204). Springer. https://doi.org/10.1007/978-1-4612-5210-8_9
- Neff, J. M. (2002). Zinc in the Ocean. In J.M. Neff (Ed.), Bioaccumulation in Marine Organisms (pp. 175–189). Elsevier.https://doi.org/10.1016/b978-008043716-3/50011-7
- Ohrel, R. L., & Register, K. M. (2006). Voluntary estuary monitoring manual. Environ. Prot. Agency Wash. DC USA, 2009–2015.
- Paalman, M. A. A., Van Der Weijden, C. H., & Loch, J. P. G. (1994). Sorption of cadmium on suspended matter under estuarine conditions; competition and complexation with major sea-water ions. Water, Air, and Soil Pollution, 73(1), 49–60. https://doi.org/10.1007/BF00477975
- Poremba, K. (2006). Hydrolytic enzymatic activity in deep-sea sediments. FEMS Microbiology Ecology, 16(3), 213–222. https://doi.org/10.1111/j.1574-6941.1995.tb00285.x
- Quinn, G. P., & Keough, M. J. (2002). Experimental Design and Data Analysis for Biologists. Cambridge University Press. https://doi.org/10.1017/CBO9780511806384
- Rauret, G. (1998). Extraction procedures for the determination of heavy metals in contaminated soil and sediment. Talanta, 46(3) , 449–455.https://doi.org/10.1016/S0039-9140(97)00406-2
- Reimann, C., & de Caritat, P. (2005). Distinguishing between natural and anthropogenic sources for elements in the environment: Regional geochemical surveys versus enrichment factors. Science of the Total Environment, 337(1–3), 91–107. https://doi.org/10.1016/j.scitotenv.2004.06.011
- Riba, I., Del Valls, T. Á., Forja, J. M., & Gómez-Parra, A. (2004). The influence of pH and salinity on the toxicity of heavy metals in sediment to the estuarine clam Ruditapes philippinarum. Environmental Toxicology and Chemistry, 23(5), 1100–1107.https://doi.org/10.1897/023-601
- Riba, I., Garcia-Luque, E., Blasco, J., & DelValls, T. A. (2003). Bioavailability of heavy metals bound to estuarine sediments as a function of pH and salinity values. Chemical Speciation & Bioavailability, 15(4), 101–114. https://doi.org/10.3184/095422903782775163
- Ruhong, L., Kun, S., Yueying, L., & Yong, S. (2010). Assessment of heavy metal pollution in estuarine surface sediments of Tangxi River in Chaohu Lake Basin. Chinese Geographical Science, 20(1), 009–017. https://doi.org/10.1007/s11769-010-0009-0
- Schroeder, E. D. (2003). Water resources. In R.A. Meyers (Ed.), Encyclopedia of physical science and technology (pp. 721–751). Academic Press. https://doi.org/10.1016/B0-12-227410-5/00821-8
- Sheeba, S. J., 2016. A study on sediment texture and environmental geochemical study of selected trace metals in the sediments of the Uppanar River Estuary, Cuddalore,Tamil Nadu, India. [Doctoral dissertation, Annamalai University]. INFLIBNET Centre. http://hdl.handle.net/10603/282963
- Siegel, S., & Castellan, N. J. (1988). Nonparametric Statistics for the Behavioural Sciences (2nd ed). McGraw-Hill Book Company.
- Simcock Lead Member, A., Halpern, B., Kirubagaran, R., Maruf Hossain, M. M., Polette, M., Smith, E., Wang, J., Bera, A., Costello, M., Duce, R., Ebinghaus, R., Kelley, J., Malone, T., Rasoanaina, J., Clark, M. R., Vecchione, M., Angel Perez, J. A., Levin, L. A., Priede, I. G., Sutton, T., … Rogers, A. (2016). Coastal, riverine and atmospheric inputs from land. In: Inniss L, Simcock A., (Eds.), coordinators, first global integrated marine assessment (pp. 1–93). United Nations.
- Simpson, S. L., Angel, B. M., & Jolley, D. F. (2004). Metal equilibration in laboratory-contaminated (spiked) sediments used for the development of whole-sediment toxicity tests. Chemosphere, 54(5), 597–609. https://doi.org/10.1016/j.chemosphere.2003.08.007
- Sulieman, H. M. A., & El Amin, M. S. (2019). Appraisal of Heavy Metal Levels in Some Marine Organisms Gathered from the Vellar and Uppanar Estuaries Southeast Coast of Indian Ocean. Journal of Taibah University for Science, 13(1), 338–343. https://doi.org/10.1080/16583655.2019.1576276
- Tessier, A., Campbell, P. G. C., & Bisson, M. (1979). Sequential extraction procedure for the speciation of particulate trace metals. Analytical Chemistry, 51(7), 844–851. https://doi.org/10.1021/ac50043a017
- US Department of Commerce, National Oceanic and Atmospheric Administration. 2017. “Estuaries, References, NOS Education Offering.” https://oceanservice.noaa.gov/education/tutorial_estuaries/est12_references.html.
- Van Assche, F. (2006). European Union (EU) risk assessment for zinc and zinc compounds. Integrated Environmental Assessment and Management, 2(2):103. https://doi.org/10.1897/1551-3793(2006)2[103a:ltte]2.0.co;2
- Venkatramanan, S., Chung, S., Ramkumar, T., Gnanachandrasamy, G., & Kim, T. H. (2015). Evaluation of geochemical behavior and heavy metal distribution of sediments: The case study of the Tirumalairajan river estuary, southeast coast of India. International Journal of Sediment Research,30(1), 28–38. https://doi.org/10.1016/S1001-6279(15)60003-8
- Venkatramanan, S., Chung, S. Y., Ramkumar, T., & Selvam, S. (2018). Ecological risk assessment of selected heavy metals in the surface sediments of three estuaries in the southeastern coast of India. Environmental Earth Sciences, 77(4), 116. https://doi.org/10.1007/s12665-018-7294-9
- Wijaya, A. R., Ohde, S., Shinjo, R., Ganmanee, M., & Cohen, M. D. (2019). Geochemical fractions and modeling adsorption of heavy metals into contaminated river sediments in Japan and Thailand determined by sequential leaching technique using ICP-MS. Arabian Journal of Chemistry, 12(6), 780–799. https://doi.org/10.1016/j.arabjc.2016.10.015
- Zhang, C., Yu, Z. G., Zeng, G. M., Jiang, M., Yang, Z. Z., Cui, F., Zhu, M. Y., Shen, L. Q., & Hu, L. (2014). Effects of sediment geochemical properties on heavy metal bioavailability. Environment International, 73(2014), 270–281. https://doi.org/10.1016/j.envint.2014.08.010
- Zhang, Y., Zhang, H., Zhang, Z., Liu, C., Sun, C., Zhang, W., & Marhaba, T. (2018). PH efect on heavy metal release from a polluted sediment. Journal of Chemistry, 2018(1), 1-7. https://doi.org/10.1155/2018/7597640
- Zhao, S., Feng, C., Wang, D., Liu, Y., & Shen, Z. (2013). Salinity increases the mobility of Cd, Cu, Mn, and Pb in the sediments of Yangtze Estuary: Relative role of sediments’ properties and metal speciation. Chemosphere, 91(7), 977-984. https://doi.org/10.1016/j.chemosphere.2013.02.001