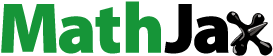
ABSTRACT
Geophagia is widespread in rural Ghana and particularly among pregnant and breastfeeding women. The perceptions of the practitioners to ingest the geophagic materials generally are not based on science and data but are hinged often on cultural and traditional beliefs and sometimes thought as substitute to treat some ailments without the use of medicine. The geophagic materials are earth products and could contain essential and harmful elements as well as materials that may have detrimental impact to human health. To assess the health risks accompanying the consumption of geophagic material, twenty geophagic materials were analyzed geochemically using Ultra Trace Aqua Regia ICP-MS analytical technique and with Qualitative X-Ray Diffraction analysis (XRD Qual) for the mineralogical phases. The results revealed quartz as the abundant mineral (average, 54.30%). The chemical analysis also revealed depletions of the analyzed elements. The health risk assessment showed the practitioners risk consuming the geophagic materials because the health risk indices for Pb and Cr were >1. Again, the substantial amount of quartz in the geophagic materials may damage the dental enamel during mastication and as well erode the gastro-intestinal lining and perforate the sigmoid colon of which the outcome will be stomach disorders.
I. Introduction
Deliberately eating earthy materials such as clay are known globally as geophagy (Abrahams, Citation2003, Citation2013; Al-Rmalli et al., Citation2010; Woywodt & Kiss, Citation2002; Ziegler, Citation1997). The practice is a centuries-old practice that is common worldwide but very much common in the developing world. There are people who frowned upon the practice but have on their part failed to justify their reasons scientifically. Despite the ill feelings from that school of thought, the geophagists have seen the results of the practice to play a role of providing some nutritional inputs in human development, addressing some psychological and cultural goals, while to others it is for medical guarantees (Danford et al., Citation1982). Meanwhile, others also crave to ingest the geophagic materials just to satisfy spiritual, religious, ritual, or social resolutions, whilst others eat it for the taste of it (Geissler et al., Citation1998; Hunter, Citation1993; Vermeer & Ferrell, Citation1985). There is an interesting belief that the ingested geophagic materials act as detoxifiers and this has been demonstrated by the practitioners in Ghana by smearing their newborn babies with it (Mensah et al., Citation2010).
Geophagia is prevalent in Ghana because most pregnant women eat clay as an appetite suppressant and it is also observed in anorexia nervosa (Woywodt & Kiss, Citation2002). In Ghana, because the practice is geospatially distributed across the country, different names have been given to the processed geophagic materials ready for ingestion. It is known as Ayilo (Ga), Agatawe (Ewes), Hyire (Akan) and several other names in other dialects. Though the geophagic materials have different names, the source materials are the weathered products from the underlying bedrocks. The weathered rocks used as geophagic materials may contain essential and harmful elements that may impact on health if the dose ratio for good health is exceeded (Arhin & Zango, Citation2017). The source materials may contain harmful elements such as aluminium, arsenic, mercury, lead, thallium, boron, and nickel etc. which can be potentially harmful to humans (Arhin & Zango, Citation2017; Kariuki et al., Citation2016; Sarpong, Citation2015). In addition, many scientists have warned against the consumption of some geophagic materials by virtue of their state as a pregnant woman or lactating mother or as ordinary persons, since there are diverse health implications associated with its consumption (Steiner-Asiedu et al., Citation2016).
There is no doubt that geophagia is beneficial but it may also be harmful as the source of the geophagic material has a link to the underlying weathered rocks. Whatever the possible benefits are, there must be a way to balance the benefit with the bane because there exists the risk of ingesting the eggs of parasitic worms as well as exposure to toxic metal ions in the process. As noted by Arhin et al. (Citation2016), the essential elements and the toxic elements coexist in the geophagic materials. The oral ingestions of these materials do not separate the essential elements from the non-essential elements. However, the positive or negative effects of geophagia depend on the physicochemical, chemical, biological and mineralogical properties of the materials ingested (Hooda, Citation2003; Mahaney et al., Citation1993; Wilson, Citation2003). Therefore, to make geophagy more attractive and safe, studies that identify elemental distributions and concentrations in the geophagic materials are relevant. More so, the knowledge of the mineralogy of the geophagic materials may give a hint on the possible health consequence if the material was wrongly sourced. On the account of these, this study seeks to examine the mineralogical makeup and geochemical contents of geophagic materials at Mfensi-Adankwame community in the Ashanti Region of Ghana.
II. Location, geology and physiographic setting of study area
The study was carried out at Mfensi-Adankwame, a town in the Atwima Nwabiagya Municipality in Ashanti Region of Ghana. It is about 22 km from Kumasi, the capital of the Ashanti Region ().
The area is characterized by undulating topography with an average topographic height of about 77 m above sea level. The vegetation is semi-equatorial, marked by double maxima of rainfall that range between 1700 mm and 1850 mm every year. The major season rainfall starts from mid-March to July, with the minor season occurring in September to mid-November. The annual temperature is fairly uniform, it averages 27°C during the rainy seasons and peaks at 31°C in March during the dry season. The mean relative humidity is between 87 and 91% with the lowest relative humidity occurring usually in February to April. During this period, the humidity ranges between 83–87% in the morning and 48–67% in the afternoon. Geologically the area is underlain by metasedimentary rocks comprising phyllites, greywackes, schists, with intrusions of basin granitoids and gneisses ().
Figure 2. Generalized Geological map of Ghana (Petersson et al., Citation2018).
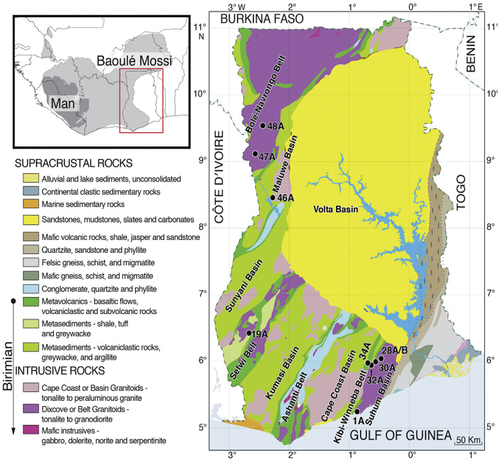
III. Materials and methods
The materials employed in the study include samples collected in the field, those collected at the field camps and those purchased at the market from the geophagic materials vendors. In all, twenty (20) geophagic materials were collected in the field, at the field camps and in the markets. Four (4) in situ samples were collected from dugout pits in the weathered clay zones in the regolith profile limit. The geophagic materials were randomly scooped within the saprolithic clay zone until a weight of 1 kg was obtained. The scooping of the samples was done at different points in the clay zones in the pits. Information such as type of the underlying geology and characteristics of the overlying saprolite and the plasticity of the geophagic materials sampled were recorded. Samples were taken from four active pits where the locals were working in. Three (3) samples each that add up to a total of 12 samples were collected from the bank materials excavated from the pits. Four (4) additional samples were also taken from already baked and ready to ingest molded clays from vendors in Kumasi Central Market. Four quality assurance and quality control (QAQC) samples were added to the field samples and the samples bought from the market. The quality assurance samples were duplicate pair of samples taken from the four already baked and ready to ingest molded clays from vendors in Kumasi Central Market. The intent of these duplicate samples was to assess the precision of the analytical quality of data.
The 20 total samples excluding the QA/QC samples were processed first by sun drying for about 24 hours after which they were reduced by sieving to <125 μm size fraction. The sieved samples were then labeled and made ready for chemical and mineralogical analysis at ALS Geochemical laboratory in Kumasi. The sample weight 100 g portion of all the samples were sent to the laboratory for ICP-MS, XRF and XRD qualitative analyses.
A. Method descriptions of the analytical techniques
Mineral identification studies using X-ray diffraction analysis (Qualitative analysis for complete mineralogy), and elemental composition of the geophagic materials using Ultra Trace Aqua Regia ICP-MS analytical procedure were used. X-ray fluorescence (XRF) analytical technique was used to determine the major oxides in the geophagic materials. The mineralogy of the geophagic materials were identified using the X-ray diffraction technique that employs Qualitative Analysis for complete mineralogy (coded XRDQual by ALS). The samples were prepared using a back loading preparation method. Using this preparation method, the analysis was performed with a Malvern Panalytical AERIS diffractometer with PIXcel detector and fixed silts with Fe filtered Co-Kα radiation. The mineral phases were identified using X’Pert Highscore Plus software. The relative phase amounts (in weight per cent) were also estimated using the Rietveld method.
The inductively coupled plasma (ICP) technique employed ALS-Chemex sample analysis protocol ME-MS41 that uses both atomic emission spectrometry (ICP-AES) and mass spectrometry (ICP-MS) techniques. The combined methods used in this analytical protocol consist of near-total and partial extraction methods. The near-total technique uses ICP-AES method and characterizes the base metals such as Ag, Cd, Co, Cu, Mo, Ni, Pb, Sc and Zn whereas elements most appropriate for aqua Regia leach like As, Bi, Hg, Sb, Se, and Te were characterized in the samples by the ICP-MS method. (Unpublished ALS-Group analytical protocols: Galway-Ireland; www.alsglobal.com). The data reported from aqua Regia leach represent only the leachable portion of the particular analyte. This implies that recovery percentages for many analytes from more resistive major elements can be very low. In this method, samples were digested with aqua Regia in a graphite heating block and left to cool. The resulting solution was diluted with deionized water and subsequently subjected to ICP-AES analysis. Results of the initial analysis were reviewed for high concentrations of bismuth (Bi), mercury (Hg), molybdenum (Mo), silver (Ag) and tungsten (W). ICP-MS analysis then commences for the remaining suite of elements.
B. pH and electrical conductivity (EC) measurements on samples
10 grams each of 8 in situ geophagic materials were dissolved in 50 millilitres (mls) of distilled water in labelled plastic test tubes. The test tube was covered with a lid and the solution was placed on a sample vortex for rigorous mixing of the solution at a speed of 2000 revolution per minute (rpm) for 60 seconds. The mixture was allowed to settle for the milky part of the solution to be suspended at the top. JENWAY 350 pH meter was used for the pH measurement. Prior to performing sample measurements, a 1 or 2-point calibration using mercury free buffer solution(s) with pH of (4 ± 0.01) for acidic, pH (7 ± 0.01) for neutral and pH (10 ± 0.01) for alkaline, all at room temperature were performed. The solution was then filtered using Whatman filter paper into another test tube. The pH meter probe was gently immersed in the filtered solution and left for about 5 minutes for the readings to stabilize before a reading was taken from the LCD screen of the pH meter. To avoid cross contamination, the probe was immersed in water between each successive test.
The electrical conductivity of the samples was measured using the Hanna Instrument. Before taking measurements, the meter was calibrated by immersing the probe in a calibration solution of known conductivity. The filtered solutions for each of the samples were poured in labelled plastic beakers. Plastic beakers were used to minimize electromagnetic interferences. The EC measurements on the samples were taken by submerging the probe in the filtered solutions. The measurement was taken when the stability symbol on the top left of the LCD disappears. The EC value in microsiemens per centimeter (µS/cm) automatically compensated for temperature was shown on the primary LCD while the secondary LCD showed the temperature of the sample (in °C).
C. Data analysis
The elements obtained from the geochemical analysis were categorized into Macro elements and Micro elements. The micro elements constitute both the essential trace elements and toxic trace elements. This classification of the elements was based on the WHO Classification of Elements in 1973 and Frieden’s Categorical Classification of Elements in 1974.
The concentrations of these elements were compared to the United State Geological Survey (USGS) average concentrations of elements in soils, and average concentrations of elements in the Upper Continental Crust (UCC) to determine enrichment or depletion levels of the elements in the analyzed samples. The micro elements in the samples were compared with some known established toxic elements also to assess the health risk of practitioners (United State Environmental Protection Agency [USEPA], Citation2002). The approach helped in completing the Health Risk Index (HRI) on ingestion of geophagic materials. The health risk index that depended on the Probable Daily Intake (PDI) of the geophagic materials by the geophagic practitioners is the product of the maximum elemental concentrations () of the analyzed trace element and the mean daily consumption (MDC) of the geophagic materials, expressed mathematically as:
Meanwhile, from Arhin and Zango (Citation2017), the mean daily consumption of geophagic materials by the geophagic practitioners in Ghana is 70 g; and to simplify the computation, the body weight (BW) of the geophagic practitioners was assumed to be 60 kg. Again, the Provisional Maximum Tolerable Daily Intake (PMTDI), a requirement for the computation of the Health Risk Index (HRI) was obtained from reports generated by Expert Committees of World Health Organization (WHO) and Food and Agriculture Organization (FAO). The Health Risk Index (HRI) was then calculated from the ratio of the Probable Daily Intake (PDI) to the Provisional Maximum Tolerable Daily Intake (PMTDI) of trace elements as:
The interpretation of Health Risk Assessment according to USEPA (Citation2002) is given as HRI < 1 as safe for human health whereas HRI > 1 as unsafe for human health.
IV. Results and discussions
Shown in are the minerals identified in the geophagic samples analyzed using the XRD Qualitative Analytical technique. The minerals identified are Quartz, Muscovite and Kaolinite. As presented in , quartz appeared to be the most dominant mineral in all the in situ samples and has an inverse relationship with kaolinite in terms of mineral concentrations in all analyzed samples ().
Figure 5. Analytical quality of results using Cu levels in the duplicate pairs of samples for precision studies.
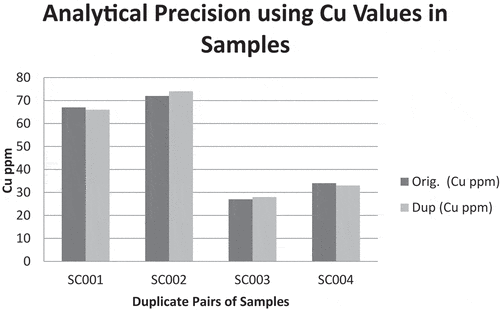
shows the analytical quality of the data received from the laboratory using Cu concentration levels in the duplicate pairs of samples. As seen in , the differences in Cu concentrations between the duplicate pairs are marginal suggesting analytical result may be reproducible and will have good precision. With this precision in analysis, the results obtained from the laboratory was considered acceptable. Hence, the selected results of the chemical analysis performed on the field in situ samples, the bank samples at the field camp and samples purchased at the market are presented in . The measured elements concentration levels differed from sample to sample with many of the samples registering measured results below detection limits of the analytical instrument. In this study, discussions were made on elements that had Public Health consequence if not monitored. Some of these elements have been established as essential for good health and others toxic if exposure exceeds certain tolerable limits. The elements are As, Cd, Cr, Cu, Hg, Mn, Mo, P, Pb, Th, Tl, Zn, Fe, K, Mg and Ca (). Apart from the toxic elements known to have carcinogenic effects, there are some essential elements that collectively perform five general physiological roles such as water and electrolyte balancing, metabolic catalysis, oxygen binding and transport as well as hormonal effects in the human body. But the achievements of the physiological roles only happen when there is an existence of right dose of the elements in the human system. The elements in are classified into macro elements (i.e., Na, Mg, P, K, Ca), micro elements (Co, Cr, Cu, Fe, Mn, Mo, Se, Zn) and toxic trace elements (Cd, Hg, As, Pb). shows the summary statistics and crustal averages with enrichment/depletion status. From , the mean concentration of Na is 0.0335 wt% which is below the background value of 1.2 wt%. The average concentration of Mg in the samples is also 0.0330 wt% which is below the USGS value of 0.9 wt%. Conversely, all the samples analyzed had P concentrations above limit of detection but their mean concentration lower than the background concentration. The mean concentration of P is 59 ppm whilst the background concentration is 430 ppm. K and Ca concentrations in the samples were both below the background concentrations, recording 0.135 wt% and 0.0225 wt% respectively as compared to USGS average concentrations of elements in soils of 1.50 wt% for K and Ca, 2.40 wt%. All the macro elements had concentrations lower than their respective background concentrations () with concentration values ranging from (0.01–0.03) wt% for Ca; (0.01–0.05) wt% for Mg; (0.01–0.04) wt% for Na; (0.04–0.23) wt% for K; and (20–90) ppm for P. Despite that, the standard deviations of the elements were very low except for P. This signifies that the elemental compositions in the individual samples are not far away from the mean concentrations (). The departure of the micro elements from their background values were as follows: mean Co concentration in the samples was 1.00 ppm compared to the background concentration of 9.10 ppm; the mean concentration of Cr, Cu and Fe were 21.5 ppm, 11.7 ppm and 0.391 ppm compared to their background concentrations of 54.0 ppm, 25.0 ppm and 2.60 ppm, respectively; Mn and Se mean concentrations in the samples were lower than their respective background concentrations although Se was detected in only seven out of the twenty samples analyzed (). The mean concentration of Mn in the samples was 34.4 ppm and that of Se was 0.229 ppm. Mo mean concentration though absent in six of the samples was 0.0529 ppm and the background concentration 0.970 ppm. Zn showed depletion in concentration in all the samples. The mean and background concentration of Zn was 6.55 ppm and 60 ppm, respectively (). Similarly, the mean concentrations of the micro elements in all the samples collected compared to the crustal averages also showed depletion in all elements (). The implications are that the practitioners will lose out because all the essential elements are below detection and showed depletion if the consumption of the geophagic materials is for medicinal purposes.
Table 1. Elements and their concentrations in the geophagic samples.
Table 2. Summary statistics of Results showing Enrichment/Depletion status of the elements as compared to the Crustal Averages of the elements in soils.
The concentrations of some known toxic trace elements in the samples were observed to have As values to range from 0.2 to 1.4 ppm with an average concentration of 0.870 ppm. Cd concentrations in all the samples were below limit of detection except eight of them which recorded 0.01 ppm. Hg concentrations in majority of the samples were below detection except for only four of the samples (). Pb was detected in all the samples, and recorded a mean concentration of 5.04 ppm. The analysis showed the elements As, Cd, Hg and Pb were depleted in the samples when compared to their background concentrations (). This has a positive outcome as the human bodies do not need these elements for their physiological roles.
From the pH measurements, all the samples recorded values ranging from 7.43 to 8.36 depicting alkaline environment (). According to Andrews-Jones (1968), in a neutral to alkaline environment, the elements Cr, Th, Zn, Cu, Co, Hg are very low to immobile; P, K, Pb, Tl, Fe and Mn have low mobility; As and Cd have medium mobility; Ca, Na, Mg have high mobility; whilst S, Mo, Se and have very high mobility. The depletion of macro elements (K and P) in the geophagic materials could be due to their low mobility in the alkaline environment. The mobility of the micro elements Fe and Mn (low mobility), Co, Cr, Cu, Zn (very low to immobile) in the neutral to alkaline environment depicts their low concentration levels in the geophagic materials. Similarly, the toxic trace elements Pb and Hg have low and very low to immobile mobilities, respectively which accounts for their low concentrations in the geophagic materials. Though the macro elements (Ca, Na, Mg), micro elements (Mo, Se) and toxic trace elements (As, Cd) have high, very high and medium mobilities, respectively, but showed depleted concentrations in the geophagic materials. This could be attributed to the high chemical index of alteration (CIA) values of the geophagic materials (). This is because high CIA values could be indicative of low concentrations of essential chemical constituents in the geophagic materials (Depetris, Citation1998).
Table 3. Daily trace element intake from 70 g geophagic materials collected and calculated health risk index in the study area.
Table 4. pH and Electrical Conductivity (EC) measurements on the geophagic materials.
Table 5. Standard Global Electrical Conductivity (EC) values of elements.
Table 6. Major oxides concentrations and chemical index of Alteration (CIA) values of the geophagic materials.
Soil electrical conductivity (EC) is a measure of the amount of salts in soil (salinity of soil). It is important indicator of soil health. According to Smith and Doran (Citation1997), soils having EC of (0–2.0) dS/m are non-saline, (2.1–4.0) dS/m are slightly saline, (4.1–8.0) dS/m are moderately saline, (8.1–16.0) dS/m are strongly saline and soils having EC greater than 16.0 dS/m are very saline. From , EC values of the geophagic samples falls below 2.0 dS/m. This shows that the geophagic materials are non-saline. Also, soils dominated by clay minerals that have a low cation-exchange capacity (CEC), such as kaolinite (), have lower EC (as shown in ) (Unpublished United States Department of Agriculture (USDA) Soil Electrical Conductivity–Soil Quality Kit Guide). In this study, the electrical conductivity values of all the samples are far lower than the standard global conductivity values () in similar materials. With the macro elements, Ca has the highest EC value, followed by Mg, to Na, K, and P the least. The micro elements have the following order of decreasing EC values as Cu > Mo > (Co, Zn) > Fe > Cr > Mn, whilst Se has no EC value. Cd has the highest EC value for the toxic trace elements followed by Pb, to As, and then Hg. Low EC values recorded for all the samples means that there is not enough water for the elements to be released from the parent material and this accounted for the low concentration of all the analyzed elements in the geophagic material.
A. The health risk due to elements in the studied geophagic materials
The geophagic samples contain significant amounts of kaolinite and quartz. Recounting from Kikouama et al. (Citation2009) and Alexander (Citation1977), clays with high kaolinite may provide medicinal benefit. This means the ingestion of clay balls from the study area will have positive impact on the health of geophagic individuals.
On the contrary, the high amounts of quartz in the samples may erode the benefits provided by kaolinite. The reason is the main component of the human dental enamel is made up of hydroxyapatite [Ca5(PO4)3OH] which has a hardness of 5 on the Mohs scale of hardness (Ekosse & Ngole, Citation2012). The ingested geophagic material containing predominantly quartz has a higher degree of hardness (7). Ingesting the geophagic material may accidentally damage dental enamel during mastication (Diko & Ekosse, Citation2014). In addition, the quartz particles in the geophagic materials can also erode the gastro-intestinal lining of the geophagic practitioners which could possibly perforate the sigmoid colon (Ekosse et al., Citation2017). The consequence will be stomach disorders. The cultural and traditional systems seek to build strong and healthy societies and will not want the inhabitants to ingest geophagic materials to make them sick neither will religious leaders intend to superintend unhealthy community and therefore will not want their constituents to practice something that will make them unwell. The perceptions and motivations of geophagists are not backed by science and data and therefore require breakeven point where there is a balance between benefits and banes.
Additional information from the Health Risk Assessment based on the health risk index on the samples revealed that ingesting 70 g daily of these geophagic materials is unsafe. This is because the probable daily intake of Cr and Pb are at levels higher than the permissible maximum tolerable daily intake. PDI of 2170 µg/kg/day compared to the tolerable daily intake of 2100 µg/kg/day was calculated for Cr. For Pb, PDI of 532 µg/kg/day was calculated as greater than the permitted maximum tolerable daily intake of 180 µg/kg/day (). Chromium and lead in the samples exceeded the limit with which they can be declared safe for consumption, i.e., HRI for Cr and Pb = > 1. Though, the measured Cr is total Cr and it could be either trivalent or hexavalent Cr. These two forms of Cr are the dominant oxidation forms as noted by Bartlett and James (Citation1988). Whereas Cr3+ is considered as essential microelement, Cr6+ is considered to be highly toxic to humans (Megharaj et al., Citation2003). Cr6+ toxicity in humans is accompanied with damaging of blood cells, livers, nervous systems, and kidneys, causing stunted growth in babies (Mackenzie et al., Citation1958; Waldron, Citation1980). Pb is considered as potentially harmful element which is generally toxic to humans. Pb has negative effects on kidneys, nervous system and heart, and leads to reduced fertility. The department of Health and Human Services (DHHS), Environmental Protection Agency (EPA) and International Agency for Research on Cancer (IARC) have determined that Pb is probably cancer-causing in humans (U.S. Department of Health and Human Services, Citation2007). Hence the presence of Cr and Pb in the geophagic materials should make the practice be considered as detrimental to human health and should not be encouraged just because the practitioners crave for it.
V. Conclusion
The geophagic materials from Mfensi-Adankwame contain macro elements such as Na, Mg, P, K and Ca; micro elements including Co, Cr, Cu, Fe, Mn, Ni, Se, V and Zn; and known toxic elements such as As, Cd and Pb. The pH values measured for all the samples revealed that the soils in the oxidized environment at the study area is alkaline and ranges from 7.43 to 8.36. This means that macro elements such as K and P that have low mobility in an alkaline environment will be patchily distributed in the source materials. This suggests that not all geophagic materials dug and processed for ingestion will contain these macro elements K and P useful for some physiological roles in the geophagic practitioners. Conversely, Ca, Na and Mg that have high mobility under all environmental conditions (be it acidic, alkaline, neutral, oxidizing or reducing environments) if available in the underlying rocks will be released and be mobilized in the soils. All the micro elements analyzed in the geophagic materials in this study behaved like the macro elements K and P, of which all of them have low mobility because of the low alkaline environment. The low concentrations of the micro elements recorded in the samples will have limited spread and their uptake by plants may be localized. The electrical conductivity values of all the samples were far lower than the standard global conductivity values of the elements in similar materials. This deprived the release of the elements from the source material which led to the low concentration values recorded for all the analyzed elements in the geophagic materials. More so, the concentrations of the essential elements (macro elements and micro elements at certain concentration level) in the samples were low, which could be attributed to high chemical index of alteration (CIA) values by virtue of the area lying in the rainforest region. The known toxic elements were deficient in the samples analyzed. Despite that, some health risks were identified to be associated with Pb and Cr as the calculated Health Risk Index values were greater than one (> 1) signifying a health threat to the geophagic practitioners.
Additionally, the study found the mineral constituents of the geophagic materials to contain quartz, kaolinite and muscovite, with quartz as the dominant mineral. Excessive amount of quartz and toxic trace elements in the geophagic materials could pose detrimental health threats to the practitioners as against kaolinite that provides medicinal benefit to the geophagic individuals. The hardness of quartz can damage dental enamel during mastication and could erode the gastro-intestinal lining of the geophagic practitioners leading to a perforated sigmoid colon of which the outcome will lead to stomach disorders. The authors conclude that for geophagy to be attractive, the knowledge of the mineralogy and geochemistry of geophagic materials must be known in order to strike the balance between the essential and harmful elements as well as adopting a method to reduce the amount of quartz content in order to make it safe for ingestion. More so, because of the variability of geochemistry of geologic materials across the landscape, the authors recommend the need to identify the provenance of the geologic makeup of the geophagic materials, which consequently may relate to different rock types. This would be useful tool to suggest the likely elements contained in the geophagic materials consumed by the practitioners. Knowledge of this information could provide a guide to where geophagic materials could be sourced to avoid spreading preventable public health diseases from harmful elements exposures.
Acknowledgments
The authors would like to acknowledge with much appreciation to African Union Commission through Pan African University Institute for Life and Earth Sciences (including Health and Agriculture) (PAULESI) for funding this research.
Disclosure statement
No potential conflict of interest was reported by the author(s).
References
- Abrahams, P. W. (2003). Human geophagy: A review of its distribution, causes and implications. Oxford University Press, London.
- Abrahams, P. W. (2013). Geophagy and the involuntary ingestion of soil. In Dordrecht (Eds.), Essentials of medical geology (pp. 433–454). Springer.
- Alexander, M. (1977). Introduction to soil microbiology (2nd ed.). John Wiley & Sons.
- Al-Rmalli, S. W., Jenkins, R. O., Watts, M. J., & Haris, P. I. (2010). Risk of human exposure to arsenic and other toxic elements from geophagy: Trace element analysis of baked clay using inductively coupled plasma mass spectrometry. Environmental Health, 9(1), 79. https://doi.org/10.1186/1476-069X-9-79
- Arhin, E., Boansi, A. O., & Zango, M. S. (2016). Trace elements distributions at Datoko-Shega artisanal mining site, northern Ghana. Environmental Geochemistry and Health, 38(1), 203–218. https://doi.org/10.1007/s10653-015-9705-0
- Arhin, E., & Zango, M. S. (2017). Determination of trace elements and their concentrations in clay balls: Problem of geophagia in Ghana. Journal of Environmental Geochemistry and Health., 39(1), 1–14 10.1007/s10653, 016–9801–9. https://doi.org/10.1007/s10653-016-9801-9
- Bartlett, R. J., & James, B. R. (1988). Mobility and bioavailability of chromium in soils. Advances in Environmental Science and Technology, 20, 267–304.
- Danford, D. E., Smith, J. J. C., & Huber, A. M. (1982). Pica and mineral status in the mentally retarded. The American Journal of Clinical Nutrition, 35(5), 958–967. https://doi.org/10.1093/ajcn/35.5.958
- Depetris, P. J. (1998). Variability of the chemical index of alteration (CIA) in the Paraná River suspended load. Mineralogical Magazine, 62(1–3), 366–367. https://doi.org/10.1180/minmag.1998.62A.1.193
- Diko, M. L., & Ekosse, G. E. (2014). Soil ingestion and associated health implications: A physicochemical and mineralogical appraisal of geophagic soils from Moko, Cameroon. Studies on Ethno-medicine, 8(1), 83–88. https://doi.org/10.1080/09735070.2014.11886476
- Ekosse, G. E., & Ngole, V. M. (2012). Mineralogy, geochemistry and provenance of geophagic soils from Swaziland. Applied Clay Science, 57, 25–31. https://doi.org/10.1016/j.clay.2011.12.003
- Ekosse, G. I. E., Ngole-Jeme, V. M., & Diko, M. L. (2017). Environmental geochemistry of geophagic materials from Free State Province in South Africa. Open Geosciences, 9(1), 114–125. https://doi.org/10.1515/geo-2017-0009
- Geissler, P. W., Shulman, C. E., Prince, R. J., Mutemi, W., Mnazi, C., Friis, H., & Lowe, B. (1998). Geophagy, iron status and anaemia among pregnant women on the coast of Kenya. Transactions of the Royal Society of Tropical Medicine and Hygiene, 92(5), 549–553. https://doi.org/10.1016/S0035-9203(98)90910-5
- Hooda, P. S. (2003). Soil ingestion affects the potential bioavailability of Cu, Mn, and Zn. In Proceedings of the 7th International Conference on the Biogeochemistry of Trace Elements (pp. 8–11). Uppsala, Sweden.
- Hunter, J. M. (1993). Macroterme geophagy and pregnancy clays in southern Africa. Journal of Cultural Geography, 14(1), 69–92. https://doi.org/10.1080/08873639309478381
- Kariuki, L., Lambert, C., Purwestri, R., & Biesalski, H. (2016). Trends and consequences of consumption of food and non‐food items (pica) by pregnant women in Western Kenya. NFS Journal, 5, 1–4. https://doi.org/10.1016/j.nfs.2016.09.001
- Kikouama, J. O., Konan, K. L., Katty, A., Bonnet, J. P., Baldé, L., & Yagoubi, N. (2009). Physicochemical characterization of edible clays and release of trace elements. Applied Clay Science, 43(1), 135–141. https://doi.org/10.1016/j.clay.2008.07.031
- Mackenzie, R. D., Byerrum, R. U., Decker, C. F., Hoppert, C. A., Langham, R. F., & Mich, E. L. (1958). Chronic toxicity studies. II. Hexavalent and Trivalent Chromium Administered in Drinking Water to Rats. Archives of Industrial Health, 18, 232–234.
- Mahaney, W. C., Hancock, R. G., & Inoue, M. (1993). Geochemistry and clay mineralogy of soils eaten by Japanese macaques. Primates, 34(1), 85–91. https://doi.org/10.1007/BF02381284
- Megharaj, M., Avudainayagam, S., & Naidu, R. (2003). Toxicity of hexavalent chromium and its reduction by bacteria isolated from soil contaminated with tannery waste. Current Microbiology, 47(1), 0051–0054. https://doi.org/10.1007/s00284-002-3889-0
- Mensah, F. O., Twumasi, P., Amenawonyo, X. K., Larbie, C., & Jnr, A. K. B. (2010). Pica practice among pregnant women in the Kumasi metropolis of Ghana. International Health, 2(4), 282–286. https://doi.org/10.1016/j.inhe.2010.09.004
- Petersson, A., Scherstén, A., Kristinsdóttir, B., Kemp, A., & Whitehouse, M. (2018). Birimian crustal growth in the West African Craton: U-Pb, O and Lu-Hf isotope constraints from detrital zircon in major rivers. Chemical Geology, 479, 259–271. https://doi.org/10.1016/j.chemgeo.2018.01.021
- Sarpong, G. (2015). Eating ayilo can be harmful. Graphic Online Publication.
- Smith, J. L., & Doran, J. W. (1997). Measurement and use of pH and electrical conductivity for soil quality analysis. Methods for assessing soil quality,49, 169–185.
- Steiner-Asiedu, M., Harrison, O. A., Vuvor, F., & Tano-Debrah, K. (2016). Quality evaluation of processed clay soil samples. Pan African Medical Journal, 24, 1. https://doi.org/10.11604/pamj.2016.24.118.8406
- U.S. Department of Health and Human Services. (2007). Toxicological profile for Lead (update) https://www.atsdr.cdc.gov/toxprofiles/tp13.pdf.PublicHealthServiceAgencyforToxicSubstancesandDiseaseRegistry
- United State Environmental Protection Agency. (2002). Region 9, preliminary remediation goals. http://www.epa.Gov/region09/waste/sfund/prg
- Vermeer, D. E., & Ferrell, R. E. (1985). Nigerian geophagical clay: A traditional antidiarrheal pharmaceutical. Science, 227(4687), 634–636. https://doi.org/10.1126/science.3969552
- Waldron, H. A. (1980). Metal in the environment. Academic Press Inc.
- Wilson, M. J. (2003). Clay mineralogical and related characteristics of geophagic materials. Journal of Chemical Ecology, 29(7), 1525–1547. https://doi.org/10.1023/A:1024262411676
- Woywodt, A., & Kiss, A. (2002). Geophagia: The history of earth-eating. Journal of the Royal Society of Medicine, 95(3), 143–146. https://doi.org/10.1177/014107680209500313
- Ziegler, J. L. (1997). Geophagy: A vestige of palaeonutrition? Tropical Medicine & International Health, 2(7), 609–611. https://doi.org/10.1046/j.1365-3156.1997.d01–359.x