Abstract
Morphological changes of testes and the annual cycle of the seminiferous epithelium were observed by optical and transmission electron microscopy to determine the male reproductive cycle of the Korean greater horseshoe bat, Rhinolophus ferrumequinum korai. In this study, R. ferrumequinum korai showed a distinct reproductive cycle, unlike other hibernating bat species. The testicular reproductive cycle of R. ferrumequinum korai consists of three main stages. The first is the spermatogenesis stage (from April to September), including spermatocytogenesis (which appears from April to May) and spermiogenesis (from June to September). The activity of spermatogenesis was highest in August. The lumen of seminiferous tubules was open from mid-April to mid-October. It was closed from November to March of the following year. The second stage is the phagocytosis stage (from mid-October to mid-November), which is a purification process to prepare for new spermatogenesis the following year. This period is called the cleansing period. The third is the dormant stage – that is, a state of holding only spermatogonia and Sertoli cells. It is an adaptation strategy to store energy for long periods of hibernation. Compared to previous studies, spermatogenesis period preceded 1 month earlier. This suggests that temperature increase can impact reproductive development and spermatogenesis.
Introduction
Mammalian species have various breeding methods. Animals in temperate zones are known to limit their reproductive activities to specific periods in order to maximize progeny survival (Nakao et al. Citation2008). In particular, changes in daylight duration are main factors that affect the start of the breeding season. Many other factors such as estrogen levels, neuropeptides, kisspeptin, gonadotropin-releasing hormone/luteinizing hormone (GnRH/LH), thyroid, food, water, housing, space and climate availability also affect the onset of reproduction (Vasantha Citation2016).
The breeding period of hibernating bats is interrupted by hibernation. It only occurs at specific times. Breeding types of hibernating bats differ greatly from those of mammals in general. Hibernation is a physiological adaptation for long-term survival (Wimsatt Citation1960, Citation1969; Krutzsch Citation1979; Lee et al. Citation1993). It has a significant impact on fertilization (Oh et al. Citation1985a). Characteristics of fertilization and early embryogenesis are different among species. Since breeding is done under low-temperature environmental conditions, adaptation strategies such as efficient energy use during breeding are needed (Funakoshi & Uchida Citation1978).
A comprehensive description of male reproduction patterns in hibernating bats is needed because their reproduction events and patterns during hibernation are significantly different from those of other mammals. Their male reproductive cycles can be divided into different patterns based on the timing and duration of major events during the annual reproductive cycle (Courrier Citation1927; Wimsatt Citation1960, Citation1969). Male reproductive patterns of bats have been classified into three types (“Pipistrellus pattern”, “Myotis pattern” and “Miniopterus pattern”) considering the spermatogenesis process, Leydig cell and associated organ changes (Gustafson Citation1979). In general, bats that live in temperate and tropical regions show a seasonal breeding pattern, although some species may not show such a pattern (Wilson Citation1979; Heideman & Bronson Citation1994; Crichton & Krutzsch Citation2000).
Recently, several studies have reported the periodicity of the seminiferous epithelium in bats, including spermatogenesis (Merwe & Rautenbach Citation1987; Morigaki et al. Citation2001; Kurohmaru et al. Citation2002; Kang & Lee Citation2004; Lee & Mōri Citation2004; Sharifi et al. Citation2004; Beguelini et al. Citation2009, Citation2011b, Citation2012a,b, Citation2013, Citation2014; Morais et al. Citation2012, Citation2013, Citation2014). Major events are similar in most species of bats. However, variations in the process between families and other taxa are often observed (Fawcett & Ito Citation1965; Singwi & Lall Citation1983; Breed & Leigh Citation1985; Oh et al. Citation1985b; Lee et al. Citation1992; Saidapur & Patil Citation1992; Phillips et al. Citation1997; Son et al. Citation1997; Kim et al. Citation1999; Beguelini et al. Citation2011a,b). In particular, there has been no report of extinguishment of immature spermatids due to phagocytosis of Sertoli cells during the annual cycle of the seminiferous epithelium except for the male reproductive type of R. ferrumequinum korai (Lee et al. Citation1993). Therefore, the objective of this study was to investigate the relationship between morphological changes in the testes and monthly changes in the differentiation of seminiferous tubules’ spermatogenic cells and review the male reproductive cycle of the Korean greater horseshoe bat (Rhinolophus ferrumequinum korai Kuroda, 1938).
Materials and methods
Experimental animals were collected and examined under the guidelines of the Kyungnam University Institutional Animal Care and Use Committee (KUIAC).
In this study, 40 male Rhinolophus ferrumequinum korai were collected from abandoned mines in Gyeongnam and Jeonnam provinces of South Korea from January 2014 to December 2015 () using inhalant anesthesia. Monthly collected materials were immersed in 5 mL of 3% glutaraldehyde aqueous solution (4°C, pH 7.4, Milloning’s buffer) and 0.01 mL of RNA stabilization solution (). To examine morphological changes of the testes and the stage of differentiation of the seminiferous epithelium according to monthly changes, testis tissues extracted from the epididymis were soaked in 3% glutaraldehyde aqueous solution (4°C, pH 7.4, Milloning’s buffer) for 24 hours. Tunica albuginea was then removed. After that, testicular tissues were cut to 1–1.5 mm3 in size and pre-fixated in 3% glutaraldehyde aqueous solution (4°C, pH 7.4, Milloning’s buffer) for 2 hours. After fixation, tissue slices were washed with the same buffer (4°C, pH 7.4, Milling’s buffer) twice (20 min per wash). They were then post-fixated in 1.33% OsO4 aqueous solution (4°C, pH 7.4, Milloning’s buffer) for 2 hours. These fixed tissue pieces were washed twice with the same buffer (20 min per wash). After washing, tissue pieces were dehydrated with increasing acetone concentration (65, 75, 85, 90, 95, 99 and 100%) followed by embedding with Epon 812 synthetic resin. These embedded tissues were cut to 400 nm thickness using an ultramicrotome (MT-1; Sorvall, Dupont) and stained with 0.5% toluidine blue. The differentiation stage of the seminiferous epithelium was observed with an optical microscope. Subsequently, continuous thin sections of 60–70 nm were obtained. They were double-stained with uranyl acetated solution and lead citrate solution followed by observation with a transmission electron microscope (TEM, H-600, Hitachi) at 75 kV.
Table I. Date examined, locality and number of individuals of the Korean greater horseshoe bat, Rhinolophus ferrumequinum korai, tested in this study (all bats were collected from survey sites of abandoned mines).
Figure 1. Photographs showing the size and morphological changes of the testis and epididymis of Korean Rhinolophus ferrumequinum korai according to month. From the middle of October to the middle of March the following year (the hibernating period), the size of testicles gradually decreased. The size of testes began to gradually increase from April (the awakening period). It showed the largest size in August (the active period). A, January; B, February; C, March; D, April; E, May; F, June; G, July; H, August; I, September; J, October; K, November; L, December. Cp, caput epididymis; Cr, corpus epididymis; Cu, cauda epididymis; T, testis.
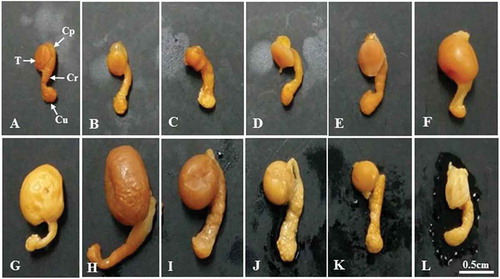
Results
To investigate periodic changes in the male reproductive cycle of Korean R. ferrumequinum korai, morphological changes of the testes and the differentiation pattern of the seminiferous epithelium were observed by optical and electron microscopy. Spermatogonia were observed in all seminiferous tubules from January to December ((–)). The periodic pattern of the male reproductive cycle was divided into active and hibernation periods. The following results were obtained.
Figure 2. (A–L). Optical microscope photographs showing the stage of differentiation of the seminiferous epithelium over a 1-year cycle. Note that seminiferous tubules from January to March are closed with lumen with only Ad-type spermatogonia and Sertoli cells. Ap-type and In-type spermatogonia as well as primary spermatocytes were observed in the first seminiferous tubules in April. From this period, the lumen began to open. In May, a number of primary spermatocytes were observed in the seminiferous tubules. In June, the earliest sperm cells were observed in the seminiferous tubules. In July, numerous spermatocytes and numerous spermatids were observed in the lumen. In August, there were a few primary spermatocytes, a number of early sperm cells and mature sperm cells, and numerous spermatozoa in the lumen. In September, numerous spermatids were observed in the spermatids and lumen, including many early sperm cells. In October, Ad-type spermatogonia, immature spermatids, and sperm cells were observed. For the first time in this period, many immature spermatids were engulfed as part of the phagocytosis process of Sertoli cells. In November, the pattern of seminiferous tubules was the same as that in October. From this time, the lumen was closed. The lumen of the seminiferous tubules in November and December was also closed. Only Ad-type spermatogonia were observed. Ad, dark type spermatogonium; Ap, pale type spermatogonium; B, B type spermatogonium; Bl, basal lamina; D, diplotene spermatocyte; Es, elongating spermatid; In, intermediate spermatogonium; L, lumen; Lf, lipofuscin; M, meta phase Ms, mature spermatid; P, pachytene spermatocyte; PL-L, pre-leptotene/leptotene spermatocyte; Rs, round spermatid; S, sperm; Se, Sertoli cell; St, spermatid; Z, zygotene spermatogonium. A, January; B, February; C, March; D, April; E, May; F, June; G, July; H, August; I, September; J, October; K, November; L, December.
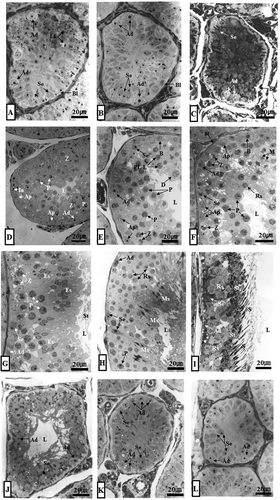
Figure 3. Electron micrographs showing early Golgi phase (June experiment group) and spermiation phase (September experiment group). In the cytoplasm of spherical sperm cells, there were abundant mitochondria and smooth endoplasmic reticulum (sER), including well-developed Golgi complexes (Gc) (A). In addition, many spermatozoa were observed in the lumen, including sperm in the cytoplasm of Sertoli cells (B). L, lumen; N, nucleus; Rb, residual body; S, sperm; Se, Sertoli cell; St, sperm tail.
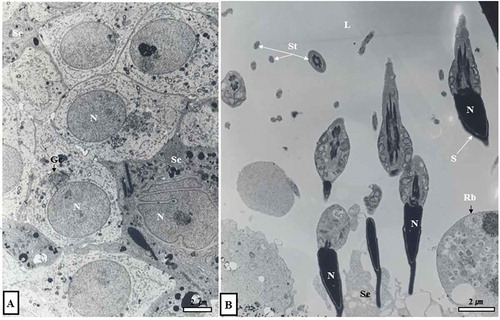
Figure 4. Optical and electron micrographs showing the phagocytosis process of Sertoli cells in seminiferous tubules in October. Note that many immature sperm cells were predated as part of the phagocytosis process of Sertoli cells (A, dotted circle in inset). In the lumen of the seminiferous tubules in October, there were sperm separating from Sertoli cells. Some sperm had already migrated from the lumen to the epididymis (B, inset). In addition, many lipofuscin granules were scattered within the cytoplasm of Sertoli cells (A, B). Ad, dark type spermatogonium; Bl, basal lamina; Irs, immature round spermatid; L, lumen; Lf, lipofuscin; S, sperm; N, nucleus; Se, Sertoli cell.
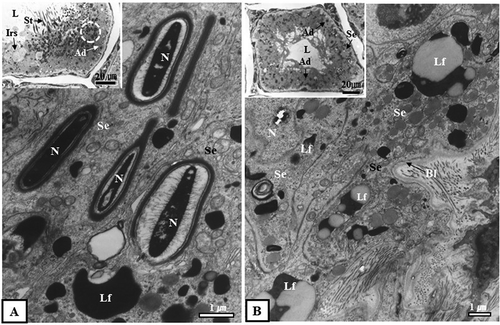
Figure 5. Optical and electron micrographs showing the phagocytosis process of Sertoli cells in the seminiferous tubules in November. The seminiferous tubules in November, like those in October, were phagocytized to a number of immature spermatids as part of the phagocytosis process of Sertoli cells (A, B). From this point on, the lumen was closed (A, inset, B, inset). Lipofuscin was scattered within the cytoplasm of Sertoli cells (A, B). Ad, dark type spermatogonium; Lf, Lipofuscin; N, nucleus; Pg, phagosome; Se, Sertoli cell; Spt, sperm tail.
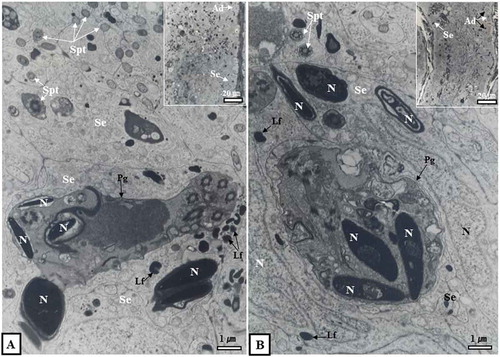
Figure 6. Differentiation stage and periodicity of the seminiferous epithelium according to month. Spermatocytogenesis was in April and May while the process of spermiogenesis was from June to September. The lumen of seminiferous tubules was open from April to October. It was closed from November to March of the following year. Ad, dark-type spermatogonium; Ap, pale-type spermatogonium; B, B-type spermatogonium; D, diplotene spermatocyte; DK, diaknesis; Es, elongating spermatid; In, intermediate spermatogonium; M, metaphase; M/A, metaphase and anaphase of the meiotic cells (meiosis I); Ms, mature spermatid; P, phacytene spermatocyte; PL/L, pre-leptotene/leptotene spermatocyte; Rs, round spermatid; S, sperm; Z, zygotene spermatogonium; □, immature round spermatids.
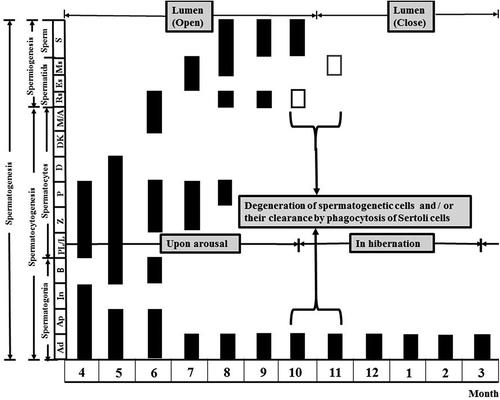
Figure 7. Hibernating Korean Rhinolophus ferrumequinum korai showing three major stages of the male reproductive cycle. The first was the spermatogenesis stage (from April to September), including spermatocytogenesis (from April to May) and the spermiogenesis (from June to early October). The second was the phagocytosis stage (from mid-October to mid-November), which was a purification process to prepare for new spermatogenesis in the following year. The third was a dormant stage. It was a state of holding only spermatogonia and Sertoli cells as an adaptation strategy to make efficient use of energy for long hibernation.
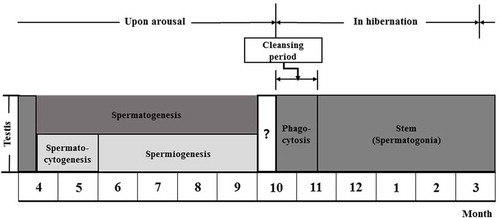
Monthly morphological changes of testes
Morphological traits of the testes of R. ferrumequinum korai were significantly different according to month (–)). The size of testis began to gradually decrease from September () to March of the following year (). It began to gradually increase from April, the awakening phase (). The testis showed the maximum size in August ().
Differentiation pattern of the seminiferous epithelium during the active phase
Spermatocytogenesis occurred throughout April (, , and ) and May (, , and ). Spermiogenesis progressed from June (, , , and ) to September (, , , and ). The activity of spermatogenesis was the highest in mid-August (, , ). The lumen of the seminiferous tubules was open from April (, and ) to mid-October (, (inset), 4B (inset), 6 and ).
Table II. Comparison of differentiation stages of the seminiferous epithelium in hibernating batspecies in Korea according to month.
Figure 8. Comparison of male reproductive patterns of four kinds of domestic bat species. Note that only immature sperm were killed by Sertoli cells in October and November, just before and immediately after hibernation of Rhinolophus ferrumequinum korai ((b), the present study). Immature spermatozoa shown in Lee et al. (Citation1993) occurred in November (a). In particular, spermatogenesis initiation in this study occurred 1 month earlier than that described in Lee et al. (1993).
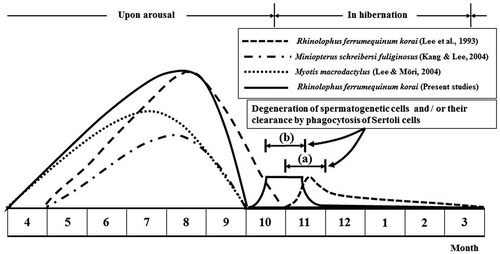
In the seminiferous tubules, a large number of Ad (dark-type) and Ap (pale-type) spermatogonia (), intermediate-type spermatogonia (, ), and primary spermatocytes were observed (, ) in April. In May, seminiferous tubules were wider than those in April. The number of primary spermatocytes in May was greater than that in April (). In June, seminiferous tubules started to show spermatids, including many primary spermatocytes (, , ). In July, many sperm were observed in the lumen of many elongated spermatids containing primary spermatocytes (). In August, a few primary spermatocytes, a number of early spermatids and mature spermatids, and numerous spermatozoa were present in the lumen (). In September, there were few Ad spermatogonia, several early spermatids (, ), abandoned spematids from Sertoli cell cytoplasm, and numerous sperm in the lumen (, ).
Differentiation pattern of seminiferous epithelium during hibernation
Upon hibernal onset starting in October, spermatozoa of seminiferous tubules were transferred to the lumen of the seminiferous tubules (, (inset)). There was no mature sperm (, (inset)). In some seminiferous tubules, spermatogonia of the Ad type, immature sperm cells, and spermatogonia cells were observed ( (inset)). For the first time in this period, numerous immature sperm cells were predated as part of the phagocytosis process of Sertoli cells (). In addition, numerous lipofuscin granules were scattered within the cytoplasm of Sertoli cells () and the lumen of the seminiferous tubules was open (, (inset), 4B (inset)). In November, seminiferous tubules were observed in immature sperm cells (, ) in the same manner as in October. Numerous immature sperm cells were predated as part of the phagocytosis process of Sertoli cells (, ). The lumen of seminiferous tubules was closed for the first time (, (inset), 5B (inset)). These seminiferous tubules were also closed in December (), January (), February (), and March (). Only Ad-type spermatogonia and Sertoli cells were present in these seminiferous tubules (). Many lipofuscin granules were scattered in the cytoplasm of Sertoli cells (, ).
Correlation between differentiation of seminiferous epithelium and monthly temperature changes
According to this study, the size of testes ( (A–L)) and the stage of differentiation of seminiferous epithelium were remarkably different according to month (–L)). These differences were also evident according to annual and monthly temperature changes (). The average annual temperatures in Changwon-si, Tongyeong-si, Namhae-gun in Gyeongnam, and Suncheon-si in Jeonnam in 1991 were 14.6, 14.7, 13.9 and 12.6°C, respectively. The average temperature in these three regions was 14.4°C. In 2015, average temperatures at Changwon-si, Tongyeong-si and Namhae-gun were 14.6, 14.8 and 14.7°C, respectively. The average temperature of these three regions was 14.7°C. It was 13.2°C at Suncheon of Jeollanamdo. When temperatures at Changwon-si, Tongyeong-si, Namhae-gun and Suncheon in April (the spermatogenesis initiation period) were compared between 1991 and 2015, the difference in temperature was about 0.4°C (1991: Changwon, 13.8°C; Tongyeong, 13.1°C; Namhae, 12.8°C; average, 13.2°C; 2015: Changwon, 13.9°C; Tongyeong, 13.2°C; Namhae, 13.7°C; average, 13.6°C). Overall, changes in annual temperature and monthly temperature between 2015 and 1991 were 0.3 to 0.4°C (April for spermatogenesis).
Table III. Comparison of temperature changes from the nearest meteorological stations at the same survey sites between 1991–1992 and 2014–2015 (Korea Meteorological Administration Citation1991, Citation1992, Citation2014, Citation2015).
Discussion
Spermatogenesis of mammals is a series of complicated and elaborate processes involving maturation and differentiation of diploid (2n) spermatogonia into highly differentiated haploid (n) germ cells (França et al. Citation1999; Paula et al. Citation1999; Calvo et al. Citation2000; Pinart et al. Citation2000, Citation2001). Differentiation processes of spermatogenesis involve many sudden changes in the cell. Such changes have been used for biochemistry and gene regulation studies as well as the identification and classification of ultrastructural changes of sperm (Mōri et al. Citation1991; Jeoung et al. Citation2006). In addition, the differentiation process of the seminiferous epithelium affects the reproductive cycle according to abiotic factors such as temperature and photoperiod (Heideman et al. Citation1992; Mello et al. Citation2009).
Although information on the duration of the seminiferous epithelium cycle is well known for a large number of bats (Beguelini et al. Citation2012a), there has been no report on the extinguishment of immature spermatids by phagocytosis of Sertoli cells during the annual cycle of the seminiferous epithelium except for the male reproductive type of R. ferrumequinum korai (Lee et al. Citation1993; ). The timing and duration of the spermatogenesis process in bats are similar to each other (Kurohmaru et al. Citation2002; Kang & Lee Citation2004; Lee & Mōri Citation2004; Beguelini et al. Citation2012a,b, Citation2013, Citation2014; Bueno et al. Citation2014). However, hibernating bat species have unique reproductive patterns. According to the present study, the size of testes began to gradually increase from April, the beginning of spermatogenesis (). In August, the size of testicles was maximized (). It then gradually decreased from September () to March (), the end of hibernation. In addition, diameter changes of seminiferous tubules showed a tendency almost the same as that of testis size (–, ). Diameter change is consistent with spermatogenesis. The maximum activity of spermatogenesis is known to be related to the diameter of the largest seminiferous tubules and the maximum testicle weight (Racey Citation1974; Racey & Tarn Citation1974; Gustafson Citation1976). Sperm production is directly related to the size of testes (Amann Citation1970; França & Russell Citation1998; Bedford Citation2008). Although body temperature does not inhibit sperm maturation in epididymis fluid, it has a significant effect on sperm viability and sperm maturation in storage capacity and cauda epididymis (Bedford Citation2008).
According to some authors, lower temperature of the epididymis makes sperm maturation and storage easier (Foldesy & Bedford Citation1982; Djakiew & Cardullo Citation1986; Jolly & Blackshaw Citation1988).
Considering cell structures of the seminiferous epithelium according to month (–) and the annual cycle of the seminiferous epithelium ( and –) shown in this study, spermatogenesis began in April () and ended in September (). Our results confirm that the male reproductive pattern of Korean R. ferrumequinum korai is the “Pipistrellus pattern”, according to Lee et al. (Citation1993), because spermatogenesis does not occur during the mating period or hibernation period. In particular, spermatocytogenesis occurred throughout April () and May () while spermiogenesis occurred from June () to September (). The activity of spermatogenesis was the highest in mid-August (). In addition, the lumen of seminiferous tubules was open from mid-April () to mid-October (). It was closed from November () to March () of the following year. The mating period was from September to the beginning of October at the latest. This means that most spermatozoa that underwent the spermatogenesis process in August and September might have migrated to the epididymis. After mating, bats soon go into hibernation, and there is no need for sperm production anymore. Therefore, they can have efficient energy use for long hibernation. In particular, from mid-October () to mid-November (), immature spermatids in seminiferous tubules during hibernation were completely destroyed by phagocytosis of Sertoli cells (, , ). In phagocytosis, more than half of differentiating spermatogenic cells are known to undergo cell apoptosis before spermatogenesis in mammals. They are rapidly and selectively cleared by phagocytosis of Sertoli cells (Lee et al. Citation1993; Nakanishi & Shiratsuchi Citation2004). Inhibition of phagocytosis in living animals will result in a decrease in the number of epididymal spermatozoa, suggesting that phosphatidylserine-mediated phagocytosis of apoptotic cells by Sertoli cells is necessary for efficient production of spermatozoa (Nakanishi & Shiratsuchi Citation2004). In hibernating bats, this phagocytosis is a preparation stage for new spermatogenesis in the next year. This is called a cleansing period.
Spermatogenesis initiation and duration of some hibernating bats are shown in and . There were some differences in the spermatogenesis initiation and duration between species (). In the case of R. ferumequinum korai (Lee et al. Citation1993) and Miniopterus schreibersi fuliginosus (Kang & Lee Citation2004), spermatogenesis initiation occurred from May. However, initiation of spermatogenesis in Myotis macrodactylus (Lee & Mōri Citation2004) and R. ferrumequinum korai in this study started in April.
Notably, spermatogenesis of Korean R. ferrumequinum korai occurred in May ( and ) in a previous study (Lee et al. Citation1993). However, it occurred in April in this study (, , and ). The fact that spermatogenesis initiation took place about a month earlier in the present study ( and ) suggests that the rising temperature might have affected the timing of spermatogenesis (). Based on yearly and monthly temperature changes shown in , the average temperature in 2015 was 0.3 to 0.4°C higher than that in 1991. It has been reported that abiotic factors such as temperature and photoperiod can affect the reproductive cycle of bats (Heideman et al. Citation1992; Mello et al. Citation2009). There is a concern that many species might become endangered if they do not develop new seasonal strategies to adapt to the rising temperature (Bradshaw & Holzapfel Citation2006).
Although the reproductive cycle of the bat Sturnira lilium might be directly influenced by factors such as temperature and food availability (Bronson Citation1985; Mello et al. Citation2009), the timing of the male cycle of tropical sperm-storing bats and onyx hibernating bats seems to be dependent on temperature more than on nutrition. Their spermatogenesis is generally stopped or suppressed in the coldest months (Gopalakrishna & Madhavan Citation1971; Myers Citation1977; Krishna & Dominic Citation1981; Krishna Citation1985).
In conclusion, the testicular reproductive cycle of R. ferrumequinum korai consists of three main stages. The first is the spermatogenesis stage (from April to September), including spermatocytogenesis (which appears from April to May) and spermiogenesis (from June to September). The second is the phagocytosis stage (from mid-October to mid-November), which is a purification process to prepare for the new spermatogenesis in the following year. This period is called the cleansing period. The third is the dormant stage that is a state of holding only spermatogonia and Sertoli cells. This is an adaptation strategy to promote efficient use of energy for long hibernation. Compared to previous studies (Lee et al. Citation1993), their spermatogenesis period preceded 1 month earlier. This suggests that temperature increase can affect their reproductive development and spermatogenesis. Even with the apparent morphological features shown in this paper, there is a limitation in that it cannot fully explain the mechanism by which the change of temperature can affect the reproductive cycle of Rhinolophus ferrumquinum korai. Therefore, in addition to electron microscope observation used in this paper, other approaches need to be used in parallel to improve our understanding of the relationship between temperature and reproductive cycle changes. Previous reports have suggested that steroid hormones, receptors and oxidative stresses might influence various aspects of reproduction. Further studies on light and temperature variations by antioxidants using enzymatic assay (Guerriero et al. Citation2003), chromatography (Guerriero et al. Citation2004) and expression analysis of steroid receptors (Guerriero et al. Citation2005, Citation2009, Citation2017a) and dosing free radicals with real-time quantitative polymerase chain reaction (PCR) (Guerriero et al. Citation2017b) are needed in the future.
Geolocation information
Country: Korea, Republic of
Region: Gyeongsangnam-do
City: Changwon-Si
Latitude: 35°11′N (Masan-si)
Longitude: 128°34′E (Masan-si)
Disclosure statement
No potential conflict of interest was reported by the author.
Additional information
Funding
References
- Amann RP. 1970. Sperm production rates. In: Johnson AD, Gomes WR, Vandemark NL, editors. New York: Academic Press. pp. 433–482.
- Bedford JM. 2008. Puzzles of mammalian fertilization and beyond. International Journal of Developmental Biology 52:415–426. DOI:10.1387/ijdb.072551jb.
- Beguelini MR, Goes RM, Taboga SR, Morielle-Versute E. 2014. Two periods of total testicular regression are peculiar events of the annual reproductive cycle of the black Myotis bat, Myotis nigricans (Chiroptera: Vespertilionidae). Reproduction, Fertility and Development 26:834–846. DOI:10.1071/RD13109.
- Beguelini MR, Marchesin SRC, Azeredo-Oliveira MTV, Morielle-Versute E. 2011a. Nucleolar behaviour during meiosis in four species of phyllostomid bats (Chiroptera, Mammalia). Genetics and Molecular Research 10:552–565. DOI:10.4238/vol10-2gmr1060.
- Beguelini MR, Moreira PRL, Faria KC, Marchesin SRC, Morielle-Versute E. 2009. Morphological characterization of the testicular cells and seminiferous epithelium cycle in six species of Neotropical bats. Journal of Morphology 270:943–953. DOI:10.1002/jmor.10731.
- Beguelini MR, Puga CC, Martins FF, Betoli AHS, Taboga SR, Morielle-Versute E. 2012a. Morphological variation of primary reproductive structures in males of five families of Neotropical bats. The Anatomical Record 296:156–167. DOI:10.1002/ar.22613.
- Beguelini MR, Puga CC, Taboga SR, Morielle-Versute E. 2011b. Ultrastructure of spermatogenesis in the white-lined broad-nosed bat, Platyrrhinus lineatus (Chiroptera: Phyllostomidae). Micron 42:586–599. DOI:10.1016/j.micron.2011.02.004.
- Beguelini MR, Puga CC, Taboga SR, Morielle-Versute E. 2013. Annual reproductive cycle of males of the flat-faced fruit-eating bat, Artibeus planirostris (Chiroptera: Phyllostomidae). General and Comparative Endocrinology 185:80–89. DOI:10.1016/j.ygcen.2012.12.009.
- Beguelini MR, Taboga SR, Morielle-Versute E. 2012b. Ultrastructural characteristics of spermatogenesis in Pallas’s Mastiff bat, Molossus molossus (Chiroptera: Molossidae). Microscopy Research and Technique 75:856–868. DOI:10.1002/jemt.22005.
- Bradshaw WE, Holzapfel CM. 2006. Evolutionary response to rapid climate change. Science 312:1477–1478. DOI:10.1126/science.1127000.
- Breed WG, Leigh C. 1985. Sperm head morphology of Australian molossid bats with special reference to the acrosomal structure. Mammalia 49:403–406. DOI:10.1515/mamm.1985.49.3.403.
- Bronson FH. 1985. Mammalian reproduction: An ecological perspective. Biology of Reproduction 32:1–26. DOI:10.1095/biolreprod32.1.1.
- Bueno LM, Beguelini MR, Comelis MT, Taboga SR, Morielle-Versute E. 2014. Ultrastructure of spermatogenesis, spermatozoon and processes of testicular regression and recrudescence in Eptesicus furinalis (Chiroptera: Vespertilionidae). Animal Reproduction Science 148:228–244. DOI:10.1016/j.anireprosci.2014.05.018.
- Calvo A, Pastor LM, Bonet S, Pinart E, Ventura M. 2000. Characterization of the glycoconjugates of boar testis and epididymis. Journal of Reproduction and Fertility 120:325–335. DOI:10.1530/reprod/120.2.325.
- Courrier R. 1927. Etude sur le déterminisme des caractères sexuels secondaires chez quelques mammiféres à activité testiculaire périodique. Archives of Oral Biology 37:173–334.
- Crichton EG, Krutzsch PH. 2000. Reproductive biology of bat. In: Racey PA, Entwistle AC, editors. Life-history and reproductive strategies of bats. London: Academic Press. pp. 364–367.
- Djakiew D, Cardullo R. 1986. Lower temperature of the cauda epididymis facilitates the storage of sperm by enhancing oxygen availability. Gamete Research 15:237–245. DOI:10.1002/mrd.1120150305.
- Fawcett DW, Ito S. 1965. The fine structure of bat spermatozoa. The American Journal of Anatomy 116:567–610. DOI:10.1002/aja.1001160306.
- Foldesy RG, Bedford JM. 1982. Biology of the scrotum. I. Temperature and androgen as determinants of the sperm capacity of the rat cauda epididymis. Biology of Reproduction 26:673–682. DOI:10.1095/biolreprod26.4.673.
- França LR, Becker-Silva SC, Chiarini-Garcia H. 1999. The length of the cycle of seminiferous epithelium in goat (Capra hircus). Tissue and Cell 31:274–280. DOI:10.1054/tice.1999.0044.
- França LR, Russell LD. 1998. The testis of domestic mammals. In: Martinez-Garcia F, Regadera J, editors. Male reproduction: A multidisciplinary overview. Madrid: Churchill Communications. pp. 197–219.
- Funakoshi K, Uchida TA. 1978. Studies on the physiological and ecological adaptation of temperature insectivorous bats III. Hibernation and winter activity in some cave-dwelling bats. Japanese Journal of Ecology 28:237–261.
- Gopalakrishna A, Madhavan A. 1971. Survival of spermatozoa in the female genital tract of the Indian vespertilionid bat Pipistrellus ceylonicus chrysothrix (Wroughton). Proceedings of the Indian Academy of Science 73:43–49.
- Guerriero G, Brundo MV, Labar S, Bianchi AR, Trocchia S, Rabbito D, Palumbo G, Abdel-Gawad FK, De Maio A. 2017a. Frog (Pelophylax bergeri, Günther 1986) endocrine disruption assessment: Characterization and role of skin poly (ADP-ribose) polymerases. Environmental Science and Pollution Research. DOI:10.1007/s11356-017-0395-2.
- Guerriero G, D’Errico G, Di Giaimo R, Rabbito D, Olanrewaju OS, Ciarcia G. 2017b. Reactive oxygen species and glutathione antioxidants in the testis of the soil biosentinel Podarcis sicula (Rafinesque 1810). Environmental Science and Pollution Research. DOI:10.1007/s11356-017-0098-8.
- Guerriero G, Di Finizio A, Ciarcia G. 2003. Oxidative defenses in the sea bass, Dicentrarchus Labrax. In: Dunn JF, Swartz HM, editors. Boston, MA: Springer. pp. 681–688.
- Guerriero G, Ferro R, Russo GL, Ciarcia G. 2004. Vitamin E in early stages of sea bass (Dicentrarchus labrax) development. Comparative Biochemistry and Physiology - Part A: Molecular & Integrative Physiology 138:435–439.
- Guerriero G, Prins GS, Birch L, Ciarcia G. 2005. Neurodistribution of androgen receptor immunoreactivity in the male frog, Rana esculenta. Annals of the New York Academy of Sciences 1040:332–336. DOI:10.1196/annals.1327.054.
- Guerriero G, Roselli CE, Ciarcia G. 2009. The amphibian (Rana esculenta) brain progesterone receptor: Relationship to plasma steroids and vitellogenic cycle during the gonadal recovery phase. Annals of the New York Academy of Sciences 1163:407–409. DOI:10.1111/j.1749-6632.2009.04438.x.
- Gustafson AW. 1976. A study of the annual male reproductive cycle in a hibernating vespertilionid bat (Myotis lucifugus lucifugus) with emphasis on the structure and function of the interstitial cells of Leydig. Dissertation Abstracts 36:4792–4793.
- Gustafson AW. 1979. Male reproductive patterns in hibernating bats. Journal of Reproduction and Fertility 56:317–331. DOI:10.1530/jrf.0.0560317.
- Heideman PD, Bronson FH. 1994. An endogenous circannual rhythm of reproduction in a tropical bat, Anoura geoffroyi, is not entrained by photoperiod. Biology of Reproduction 50:607–614. DOI:10.1095/biolreprod50.3.607.
- Heideman PD, Deoraj P, Bronson FH. 1992. Seasonal reproduction of a tropical bat, Anoura geoffroyi, in relation to photoperiod. Journal of Reproduction and Fertility 96:765–773. DOI:10.1530/jrf.0.0960765.
- Jeoung SJ, Park JC, Kim HJ, Bae CS, Yoon MH, Lim DS, Jeong MJ. 2006. Comparative fine structure of the epididymal spermatozoa from three Korean shrews with considerations on their phylogenetic relationships. Biocell 30:279–286. PubMed: 16972552.
- Jolly SE, Blackshaw AW. 1988. Testicular migration, spermatogenesis, temperature regulation and environment of the sheath-tail bat, Taphozous georgoanus. Journal of Reproduction and Fertility 84:447–455. DOI:10.1530/jrf.0.0840447.
- Kang MS, Lee JH. 2004. Annual cycle of the seminiferous epithelium of Miniopterus schreibersi fuliginosus. The Journal of Biomedical Laboratory Sciences 10:435–445.
- Kim SS, Lee JH, Son SW, Choi BJ. 1999. Morphological comparison of spermatozoa in the Korean greater horseshoe bat (Rhinolophus ferrumequinum korai) and long-fingered bat (Miniopterus schreibersi fuliginosus). Korean Journal of Electron Microscopy 29:1–10.
- Korea Meteorological Administration. 1991. Annual climatological report, 1991. Seoul: Korea Meteorological Administration. pp. 84–193.
- Korea Meteorological Administration. 1992. Annual climatological report, 1992. Seoul: Korea Meteorological Administration. pp. 73–166.
- Korea Meteorological Administration. 2014. Annual climatological report, 2014. Seoul: Korea Meteorological Administration. pp. 84–193.
- Korea Meteorological Administration. 2015. Annual climatological report, 2015. Seoul: Korea Meteorological Administration. pp. 117–267.
- Krishna A. 1985. Reproduction in the Indian pipistrelle bat Pipistrellus mimus. Journal of Zoology 206:41–51. DOI:10.1111/j.1469-7998.1985.tb05635.x.
- Krishna A, Dominic CJ. 1981. Reproduction in the vespertilionid bat Scotophilus heathi Horsefield. Archives of Biology 92:247–258.
- Krutzsch PH. 1979. Male reproductive patterns in nonhibernating bats. Journal of Reproduction and Fertility 56:333–344. DOI:10.1530/jrf.0.0560333.
- Kurohmaru M, Saruwatari T, Kimura J, Mukohyama M, Watanabe G, Taya K, Hayashi Y. 2002. Seasonal changes in spermatogenesis of Japanese Lesser Horseshoe bat, Rhinolophus cornutus from a morphological viewpoint. Okajimas Folia Anatomica Japonica 79:93–100. DOI:10.2535/ofaj.79.93.
- Lee JH, Choi BJ, Son SW. 1992. Spermiogenesis in the Korean greater horseshoe bat, Rhinolophus ferrumequinum korai. Korean Journal of Electron Microscopy 2:97–117.
- Lee JH, Mōri T. 2004. Annual cycle of the seminiferous epithelium of Myotis macrodactylus. Journal of the Faculty of Agriculture Kyushu University 49:355–365.
- Lee JH, Son SW, Mōri T, Shiraishi S. 1993. Studies on the reproductive pattern in the male of Korean greater horseshoe bat, Rhinolophus ferrumequinum korai. I. The cycle of seminiferous epithelium and histological changes of testis. Korean Journal of Zoology 36:36–50.
- Mello MAR, Kalko EKV, Silva WR. 2009. Ambient temperature is more important than food availability in explaining reproductive timing of the bat Sturnira lilium (Mammalia: Chiroptera) in a montane Atlantic forest. Canadian Journal of Zoology 87:239–245. DOI:10.1139/z09-010.
- Merwe M, Rautenbach IL. 1987. Reproduction in Schlieffen’s bat, Nycticeius schlieffenii, in the eastern Transvaal Lowveld, South Africa. Journal of Reproduction and Fertility 81:41–50. DOI:10.1530/jrf.0.0810041.
- Morais DB, Barros MS, Paula TAR, Freitas MBD, Gomes MLM, Matta SLP. 2014. Evaluation of the cell population of the seminiferous epithelium and spermatic Indexes of the Bat Sturnira lilium (Chiroptera: Phyllostomidae). PLoS ONE 9:e101759. DOI:10.1371/journal.pone.0101759.
- Morais DB, Paula TAR, Barros MS, Balarini MK, Freitas MBD, Matta SLP. 2013. Stages and duration of the seminiferous epithelium cycle in the bat. Sturnira lilium. Journal Anatomy 222:372–379. DOI:10.1111/joa.12016.
- Morais DB, Paula TAR, Freitas KM, Matta SLP. 2012. Cycle of the seminiferous epithelium of the bat Molossus molossus, characterized by tubular morphology and acrosomal development. Asian Pacific Journal of Reproduction 1:303–307. DOI:10.1016/s2305-0500(13)60097-4.
- Mōri T, Arai S, Shiraishi S, Uchida TA. 1991. Ultrastructural observations on spermatozoa of the Soricidae, with special attention to subfamily revision of the Japanese water shrew Chimarrogale himalayica. Journal of the Mammalogical of Society of Japan 16:1–12.
- Morigaki T, Kurohmaru M, Kanai Y, Mukohyama M, Hondo E, Yamada J, Agungpriyono S, Hayashi Y. 2001. Cycle of the seminiferous epithelium in the Java fruit bat (Pteropus vampyrus) and the Japanese Lesser Horseshoe bat (Rhinolophus cornutus). Journal of Veterinary Medical Science 63:773–779. DOI:10.1292/jvms.63.773.
- Myers P. 1977. Patterns of reproduction of four species of vespertilionid bats in Paraguay. Berkley, CA: University of California press. DOI:10.1248/bpb.27.13.
- Nakanishi Y, Shiratsuchi A. 2004. Phagocytic removal of apoptotic spermatogenic cells by Sertoli cells: Mechanisms and consequences. Biological and Pharmaceutical Bulletin 27:13–16. DOI:10.1248/bpb.27.13.
- Nakao N, Ono H, Yoshimura T. 2008. Thyroid hormones and seasonal reproductive neuroendocrine interactions. Reproduction 136:1–8. DOI:10.1530/rep-08-0041.
- Oh YK, Mōri T, Uchida TA. 1985a. Prolonged survival of the Graafian follicle and fertilization in the Japanese greater horseshoe bat, Rhinolophus ferrumequinum nippon. Journal of Reproduction and Fertility 73:121–126. DOI:10.1530/jrf.0.0730121.
- Oh YK, Mōri T, Uchida TA. 1985b. Spermiogenesis in the Japanese greater horseshoe bat, Rhinolophus ferrumequinum Nippon. Journal of the Faculty of Agriculture Kyushu University 29:203–209.
- Paula TA, Chiarini-Garcia H, Franca LR. 1999. Seminiferous epithelium cycle and its duration in capybaras (Hydrochoerus hydrochaeris). Tissue and Cell 31:327–334. DOI:10.1054/tice.1999.0039.
- Phillips DM, Rasweiler JJ, Murwali F. 1997. Giant, accordioned sperm acrosomes of the greater bulldog bat, Noctilio leporinus. Molecular Reproduction and Development 48:90–94. DOI:10.1002/(SICI)1098-2795(199709)48:1%3C90::AID-MRD11%3E3.0.CO;2-%23.
- Pinart E, Bonet S, Briz MD, PastorL M, Sancho S, Garcia N, Badia E, Bassols J. 2001. Lectin affinity of the seminiferous epithelium in health and eryptorchid post-puberal boars. International Journal of Andrology 24:153–164. DOI:10.1046/j.1365-2605.2001.00282.x.
- Pinart E, Sancho S, Briz MD, Bonet S, Garcia N, Badia E. 2000. Ultrastructural study of the boar seminiferous epithelium: Changes in cryptorchidism. Journal of Morphology 244:190–202. DOI:10.1002/(sici)1097-4687(200006)244:3<190::aid-jmor4>3.3.co;2-2.
- Racey PA. 1974. The reproductive cycle in male noctule bats, Nydalus nodulo. Journal of Reproduction and Fertility 41:169–182. DOI:10.1530/jrf.0.0410169.
- Racey PA, Tarn WH. 1974. Reproduction in male Pipistrellus pipistrellus (Mammalia: Chiroptera). Journal of Zoology 172:101–122. DOI:10.1111/j.1469-7998.1974.tb04096.x.
- Saidapur SK, Patil SB. 1992. Kinetics of spermatogenesis in mega chiropteran bat, Rousettus leschenaulti (Desmarset): Seminiferous epithelial cycle, frequency of stages, spermatogonial renewal and germ cell degeneration. Indian Journal of Experimental Biology 30:1037–1044.
- Sharifi M, Ghorbani R, Akmali V. 2004. Reproductive cycle in Pipistrellus kuhlii (Chiroptera, Vespertilionidae) in western Iran. Mammalia 68:323–327. DOI:10.1515/mamm.2004.031.
- Singwi MS, Lall SB. 1983. Spermatogenesis in the non-scrotal bat Rhinopoma kinneari Wroughton (Microchiroptera: Mammalia). Acta Anatomica 116:136–145. DOI:10.1159/000145735.
- Son SW, Lee JH, Cheon HM. 1997. Spermiogenesis in the Korean Daubenton’s Bat (Myotis daubentonii ussuriensis). Development and Reproduction 1:9–24.
- Vasantha I. 2016. Physiology of seasonal breeding: A review. Journal of Veterinary Science and Technology 7:331. DOI:10.4172/2157-7579.1000331.
- Wilson DE. 1979. Reproductive patterns. In: Baker RJ, Jones JR, Carter DC, editors. Texas: Special Publication of the Museum Texas Tech University. pp. 317–318.
- Wimsatt WA. 1960. Some problems of reproduction in relation to hibernation in bats. Bulletin of Museum of Comparative Zoology at Harvard College 124:239–270.
- Wimsatt WA. 1969. Some interrelations of reproduction and hibernation in mammals. Symposium of the Society for Experimental Biology 23:511–549. PMID: 5367180.