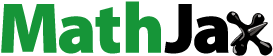
Abstract
This study assessed the effectiveness of a novel conservation strategy for the native brown trout populations in Sardinia (Italy). The conservation measures included: (i) installation of an electric fish barrier; (ii) removal of all the resident introgressed brown trout upstream from the barrier; (iii) restocking of the upstream region with native Sardinian trout genetically characterised; and (iv) estimation of native trout population size with prediction of future growth. Introgressed brown trout were removed using multiple-pass electrofishing methods in a 2-km region upstream from the barrier. In two eradication campaigns, conducted in 2010 and 2012, a total of 1801 trout were captured, comprising a total biomass of 55,501 g. The capture efficiency analysis showed total capture rates of over 99% were achieved for parr (0+ age cohort), and 100% for adult trout. After the eradication campaigns we restocked this area with native brown trout (Adriatic lineage, AD, according to the mitochondrial DNA control region nomenclature). The monitoring campaigns showed good survival (S = 60%) and reproduction of 3.1 recruits/adult trout. Successful breeding was recorded starting from the summer of 2015 and a self-sustaining population resulted after only two restocking events. The results of this restoration project allow recommendations to be made for future conservation eradication and repopulation projects for restoration of typical Mediterranean streams.
Introduction
Freshwater species worldwide are significantly affected by human intervention (Saunders et al. Citation2002). Harmful interventions such as habitat pollution and destruction, overexploitation and introduction of invasive species are significant factors driving native populations towards decline and range reduction (Almodóvar & Nicola Citation1998; Dudgeon et al. Citation2006).
Over the last two decades, native salmonid species have been the focus of important conservation projects, owing to population reduction and extinction in many countries (Crivelli et al. Citation2000; Araguas et al. Citation2008, Citation2009; Carmona-Catot et al. Citation2010; Caudron et al. Citation2011; Caputo Barucchi et al. Citation2015). In particular, the introduction of non-native Atlantic trout has included foreign genes into Mediterranean brown trout populations, and has altered the traits of the native gene pool (Berrebi et al. Citation2000, Citation2017; Nonnis Marzano et al. Citation2003; Caputo et al. Citation2004; Querci et al. Citation2013; Gratton et al. Citation2014; Splendiani et al. Citation2016). In this context, the nuclear locus LDH-C1 and the mitochondrial DNA control region (mtDNA CR) are well-known tools used to estimate introgression from the Atlantic hatchery stocks. The LDH-C1 locus is characterised by two co-dominant alleles, the LDH C1*100 fixed in the Mediterranean native populations, and the LDH C1*90 fixed in the North Atlantic lineage (McMeel et al. Citation2001).
The mtDNA gives a testable method of classification, dividing Salmo trutta into five main evolutionary lineages: Atlantic (AT), Mediterranean (ME), Marble (MA), Adriatic (AD) and Danubian (DA) (Bernatchez et al. Citation1992; Bernatchez Citation2001). These five main European groups were increased to seven in later studies: the Duero lineage (DU, Vera et al. Citation2010) and the Tigris lineage (TI, Susnik et al. Citation2005). The AT lineage is native to both Iberian Peninsula (Cortey et al. Citation2009) and Sicilian Island (Schöffmann et al. Citation2007; Fruciano et al. Citation2014) populations, while DU lineage is native solely in the Iberian Peninsula (Vera et al. Citation2010). Introgression detection with mtDNA is required because the four AT haplotypes present in hatchery stocks are not naturally present in wild populations (Cortey & Garcia-Marin Citation2002). The Southern European basins host native AD, AT, DA, DU and MA, and the introgression is described by the presence of the four AT hatchery haplotypes.
Sardinian rivers in Italy are home to two introduced, non-native salmonid species, the brown trout (Salmo trutta Linneaus, 1758 – North Atlantic origin) and the rainbow trout ((Oncorhyncus mykiss (Walbaum, 1792) – North American origin)), as well as the native Mediterranean brown trout ((Salmo cettii Rafinesque, 1810 – formerly Salmo (trutta) macrostigma (Duméril, 1858)) (Sabatini et al. Citation2006, Citation2011; Kottelat & Freyhof Citation2007; Massidda et al. Citation2008; Zaccara et al. Citation2015). Non-native trout in Sardinia have replaced native populations over much of their range (Massidda Citation1995). Atlantic brown trout have interbred with local forms, altering their gene pool. Consequently, in many areas of Sardinia, entire populations of native trout have disappeared and have been replaced by hybrid or non-native trout (Sabatini et al. Citation2006, Citation2011).
As a result, Mediterranean brown trout are listed as Critically Endangered by the International Union for Conservation of Nature (IUCN; Rondinini et al. Citation2013), holding special conservation status at the regional level (“Decree of the Assessor of the Defense of the Environment” 10.05.1995 n. 412).
The decline of native trout populations has therefore attracted the attention of biologists and public institutions, seeking to implement conservation strategies. Wildlife refuges and sanctuaries only offer a partial solution to restore the native gene pool; to date, few such areas have been created specifically for salmonid species (Poteaux et al. Citation1998; Reid et al. Citation2001; Vincenzi et al. Citation2007, Citation2008; Araguas et al. Citation2009).
The headwater of the Ermolinus stream in central-eastern Sardinia was chosen for preliminary trials of the first experimental conservation measure for the Mediterranean native trout population. Historic literature was used, as well as information from the Forest Guard, local individuals and fishermen, to assess the current presence and distribution of native trout in the study area (Pomini Citation1940; Cottiglia Citation1968; Massidda et al. Citation1996; Orrù et al. Citation2010). A previous study, conducted between 2005 and 2007, analysed the nuclear locus LDH-C1 and mtDNA CR of salmonids and showed that the stream was inhabited by non-native trout and hybrids (Sabatini et al. Citation2006, Citation2011). The hybridisation with domestic Atlantic trout was a consequence of past restocking practices in the Ermolinus stream and other rivers within the Flumendosa basin.
One management option to minimise the threat posed by invasive non-native trout is to isolate and remove the non-native trout species, and then reintroduce a native trout strain. Generally, a fundamental objective of any reintroduction effort is the establishment of a self-sustaining population (Araguas et al. Citation2008; Servanty et al. Citation2014).
The use of barriers to fish movement represents an important tool in the conservation of native fish species. Barriers are increasingly being used in many countries to protect threatened native trout populations from damaging interactions with non-native fish (Applegate et al. Citation1952; Kruse et al. Citation2001; Novinger & Rahel Citation2003; Clarkson Citation2004; Avenetti et al. Citation2006; Dawson et al. Citation2006).
Towards this end, the following measures were taken: (i) installation of an electric barrier to isolate the upper part of the stream; (ii) electrofishing upstream of the barrier to remove all the fish present; (iii) restoration of the Sardinia trout population by a series of stocking experiments using genetically characterised native trout; and (iv) estimation of native trout population size and prediction of future population growth.
The approach described in this paper presents a model for restoration of the original biodiversity in a typical Mediterranean stream and could be applied in many similar reintroduction management settings. This strategy might provide an efficient method for evaluating and guiding management of threatened natural trout populations. For a species under conservation concern, eradication of non-native species and subsequent reintroduction of genetically purebred native species represents the main step for establishment of protected areas know as Special Areas of Conservation (SACs), as recommended by the EU Habitat Directive (92/43/EC).
Material and methods
Study site
The restoration experiment was conducted in the Ermolinus Stream (Sardinian Italy), an upper tributary of the Flumendosa River (39°52ʹN, 9°23ʹE). The stream is located in the Montarbu Forest (2700 ha), managed by the Sardinian Forest Agency (Fo.Re.S.TA.S.). The entire forest was designated a wildlife protection oasis in 1980 (Regional Law 157/92). The oasis is set in the Gennargentu Mountains system, a Site of Community Importance (ITB021103; European Commission Habitats Directive 92/43/EEC). Bi-seasonal climatic features typify this region. It has a hot-arid, drought-prone summer and a wet autumn–winter. The lowest temperatures are recorded in January, which is also the coldest month on average, while the warmest month is August. The source of the Ermolinus Stream is 1200 m above sea level, in the Gennargentu Mountains in central eastern Sardinia, Italy. The stream is 5 km long and merges with the Anus Stream to form the River San Girolamo, which runs into the Flumendosa River ().
Figure 1. Map of the Montarbu Forest (Central Eastern Sardinia, Italy) showing the borders of the wildlife protection oasis, the main rivers, and the area isolated by an electric barrier.
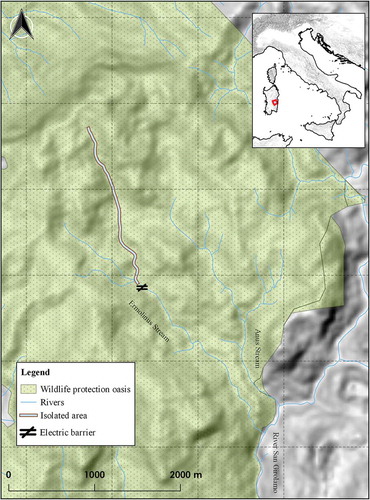
Land cover is characterised by Mediterranean maquis with a more or less thin soil cover. Holm oak (Quercus ilex L.), hop hornbeam (Ostrya carpinifolia Scop.) and yew (Taxus baccata L.) are the most abundant riparian trees. The stream is characterised by clear, well-oxygenated water, a moderately fast current, and the presence of waterfalls, jumps, ripples, turbulence and pools. The stream varies between 1 and 5 m in width, and is 0.10 to 1.80 m deep, and the streambed consists of 20% boulders, 50% gravel and 30% rubble. Surface runoff is almost perennial in the stream where it flows on more or less impermeable rocks, but disappears as soon as the stream crosses the carbonate rocks. The carbonate part of the stream is characterised by the presence of travertine deposition (calcium carbonate) that occurs in a variety of forms, from cementing of substrate particles to small dams that create pools. The stream profile (starting at transect 001, located upstream the electric barrier), shows that in the carbonate parts (sections 010–018 and 033–040) the slope is irregular and higher among the measured transects, whereas the impermeable part is characterised by an almost horizontal profile (between sections 001–009 and 019–032). Surface runoff is activated after important rain events and can persist for several days, also in the steeper part of the stream.
The electric fish barrier
In the spring of 2010, several stream sections were investigated within the Ermolinus stream for the potential placement of a fish barrier. Based on physical site characteristics and other considerations, an electric barrier was installed in an appropriate area in the stream. The barrier was built near the Forest Guard station about 2 km from the stream source. At the electrical barrier site, the Ermolinus stream is 5 m wide and up to 0.2 m deep, allowing the barrier to work effectively at any water flow level likely to be encountered. The site also benefits from a wooden bridge that closes it off to strangers and animals and which made construction and servicing of the barrier easier. The efficiency of the barrier was further enhanced by the presence of a waterfall just below the bridge, which forms a natural barrier to the upstream passage of fish. The electric fish barrier was designed by Scubla Aquaculture & Co. Inc. and consisted of elements discharging an electrical current through the water. The barrier had two panels: a power supply located near the Forest Guard station and a control panel next to the stream. Power was supplied by a battery package, recharged directly from solar panels, or from a power cable in case of emergency. A command board allowed regulation of the voltage and pulse frequency. The electric fish barrier produced up to 1000 V and a continuous pulse frequency of 5 pulses per second. The electrical circuit was made up of two metal electrodes submersed in the water with the voltage applied between them (). Then the isolated upstream section (about 2 km in total) was divided into 40 50-m study sections using a global position system unit. All sections were located between 839 and 981 m above sea level (masl). The gradient (%) of each section was estimated by the ratio between the change in altitude along the section and the horizontal length of the section.
Eradication of brown trout population in the Ermolinus stream headwater
Following the installation of the electric barrier in June 2010, two consecutive eradication operations were conducted, in June 2010 and July 2014. Considering the genetic analysis reported in previous studies (Sabatini et al. Citation2011) we decided to remove all the trout present in the headwater of Ermolinus stream. The fish were removed using two motor-powered, backpack-mounted electrofisher units (5.2–2.8 Å, 230–400 V, 1300 W). Fish were caught by two teams, each composed of three operators. One person operated the electrofisher unit, while two others caught the stunned fish with landing-nets. Before each removal session, the upper and lower limits of the section were blocked with nets to prevent trout moving in and out of the section (Kulp & Moore Citation2000). All trout captured were measured and weighed on site: total length (TL, to the nearest 0.1 cm) and total weight (TW, nearest 0.1 g). After processing, the fish were placed in large containers and released downstream of the barrier. Capture of the existing fish commenced in June 2010, after the barrier had been installed, and when the stream was at base flow. Four years later, in July 2014, a second eradication was conducted because of an outage of the electric barrier. The barrier outage was apparently caused by a lightning strike that damaged several components. Depletion estimates were obtained by 10 removal passes using the maximum likelihood procedure (Carle & Strub Citation1978; Lockwood & Schneider Citation2000). This method provided a statistical estimate of the actual proportion of fish present that were captured. Fish removal was considered complete when two consecutive electrofishing passes caught no further fish. In both eradication activities brown trout densities (N fish/m2) and biomass (g/m2) were estimated for each section. The non-parametric Kolmogorov–Smirnov (K-S) test was also used, to determine whether length–frequency distributions changed over the two eradication campaigns.
Restocking of native trout and population growth model
In 2010, immediately after the depopulation had been completed, the upstream area was stocked with 0+ parr trouts (N = 100, TL 6.6–9.0 cm) in order to study the performance of the barrier associated with the efficiency of the eradication. The fish used in the experiment were hatchery-reared genetically characterised native brown trout (Sadali fish farm, Sardinia, Italy) (Sabatini et al. Citation2011). After the second eradication, a second phase of stocking activities was conducted in 2014 and 2015 using parr and adults (N = 135, from age 0+ to 3+) obtained from the same fish farm. The native trout were stocked in the sections of the stream following the distribution of the eradicated population. In 2014 and 2015, before the stocking activities, in order to identify individual trout, all sub-adults and adults were tagged with Therachip OS080N nano-microchip (1.25 × 7 mm) passive integrated transponder (PIT) tags. These tags were implanted into the peritoneal cavity as reported by Gheorghiu et al. (Citation2010), using a Bioforlife Therapet Syringe. Brown trout were starved for at least 3 days prior to tagging to minimise complications that might arise due to the presence of partially digested food in the digestive system of the fish (Gheorghiu et al. Citation2010). Prior to tag implantation, the fish were anaesthetised with tricaine methanesulphonate (MS-222) and their TL determined. Both tagging and stocking operations were conducted under veterinary control and before stocking all fish were subjected to veterinary examination. If fish were determined suitable for stocking, they received a veterinary certificate of health in accordance with Directive 91/67/ECC (Annex E) that lays out good practice in order to reduce the risk of spreading diseases of fish.
After the restocking phases, we conducted an electro-fishing survey to evaluate the effectiveness of the barrier at preventing upstream invasion of introgressed trout, and the condition of the stocked population. Fish monitoring in the Ermolinus stream above the electrical barrier was initiated in 2012 immediately after the restocking. In 2012, for each specimen captured a fin clip was collected for genomic DNA extraction. Genetic analyses were performed using mitochondrial DNA control region (mtDNA CR) and nuclear markers (locus LDH-C1*) as reported in Sabatini et al. (Citation2006, Citation2011). Site were sampled in late spring and summer when the stream was at base flow. Sampling followed a random selection of eight sections, among the 40 sections identified upstream of the barrier. This sampling activity allowed us to monitor the population and to follow the age cohorts annually.
Based on the data obtained from both phases of restocking and monitoring sampling we produced a population growth model in order to assess the effect of management action. All the spawners restocked were tagged, allowing for accurate assignment of the trout to age cohorts. Conversely, the age of new generations (age cohorts 2015 and 2016) were determined by scalimetry combined with length frequency distribution (Burnet Citation1969; Pauly et al. Citation1995). Age determination was performed on scales of 32 fish samples in 2015 (age cohorts 3+, 4+) and 37 fish in 2016 (age cohorts 1+, 3+ and 4+).
In order to create the model, the annual reproductive rates (R) and annual survival rates (S) were calculated. The model set-up was performed using both tagged and untagged fish data. The annual reproductive rate was computed as follows:
where (Nr) is the number of recruits for the tRtal number of adult trout (Na).
The annual survival rates (S) were calculated by dividing the number of adults that survived after 1 year from restocked specimens (Nat+1), by the number of adults introduced (Nat):
On the basis of this result, and using the mean population parameters of R and S for different years, the demographic model was established. The number of recruits for each year (Nr) was computed considering the spawners from age cohorts 2+ to 6+, as follows:
With the specimen number for each cohort (Nct), the number of survivors after 1 year (Nct+1) was obtained using the mean S, as follows:
The model used in the present work includes some assumptions that should be taken into account when considering the results:
It was assumed that the only factor changing the population structures of reintroduced trout was the rate of harvesting by humans. In the absence of human fishing, the fish population would not change;
It was assumed that population-limiting factors such as disease, predation or climate would not change over time and did not influence the model set-up.
Considering that the reproduction rate and survival rate can be affected by many abiotic and biotic factors (Vincenzi et al. Citation2007, Citation2008), the model results should be interpreted with caution.
Results
Eradication of brown trout population in the Ermolinus stream headwater
Fishing efforts encompassed over 2000 m of stream, totalling 216 hours (5.5 h/50 m section) in 2010, and 56 hours (1.4 h/50 m section) in 2014. During the two eradication campaigns, a total of 1355 and 446 trout were captured for a total biomass of 23,830 and 31,671 g in 2010 and 2014, respectively.
In 2014, fish density was highest in the initial reaches of the stream, from 0.72 to 1.18 fish/m2 (sections 004 and 006), as well as in intermediate reaches (section 017, 0.62 fish/m2). In 2010, the density calculated in the sections ranged from 0.01 to 1.27 fish/m2 (sections 013 and 018, respectively). In both campaigns, the largest fish were usually caught in the intermediate reaches of the stream, between sections 019 and 030, and section 027 had particularly large fish. This part of the stream is mainly characterised by the presence of impermeable rocks that create pools. The smallest fish were caught in sections 016 and 007, which had a substrate typical of a nursery habitat. Low water speeds (< 0.2 m/s), water depths of less than 15 cm, and the presence of fine gravel characterised these areas.
The eradicated trout length–frequency distributions were significantly different between 2010 and 2014 (K-S test: D = 0.4, P = 0.01). Length–frequency distributions for brown trout for each year are provided in . Fish that were caught ranged from 1.5 to 29.0 and 4 to 29.7 cm TL in 2010 and 2014, respectively, with an increase of 3.4 cm of TL and 53 g of TW. A high proportion of the fish caught (63%) had total lengths under 9 cm TL (0+ age class) while the remaining 37% comprised the sub-adult and adult specimens. In 2014, 47% of the caught brown trout were age 0+ and 53% were sub-adults and adults. During the eradication campaigns brown trout were caught in 27 out of the total 40 sections that were electrofished in 2010, and from 23 sections in 2014 (). The fish density and total biomass captured in each section averaged (mean ± standard deviation, SD) from 0.34 ± 0.36 to 0.19 ± 0.17 fish/m2 and from 5.96 ± 6.26 to 13 ± 9.90 g/m2 in 2010 and 2014, respectively.
Figure 3. Length frequencies in the catches of non-native brown trout in the two eradication campaigns (2010 and 2014).
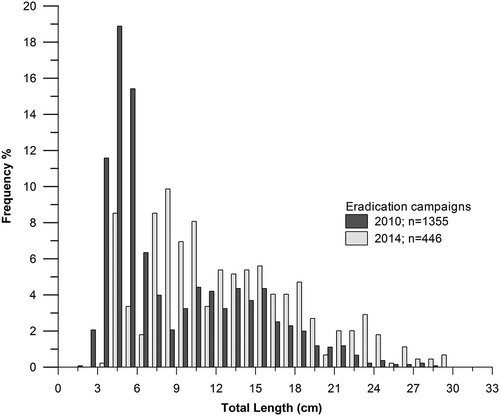
Figure 4. Density and biomass of Ermolinustrout of the 40 sections along the longitudinal profile of the Ermolinus stream during the eradication campaigns of 2010 and 2014.
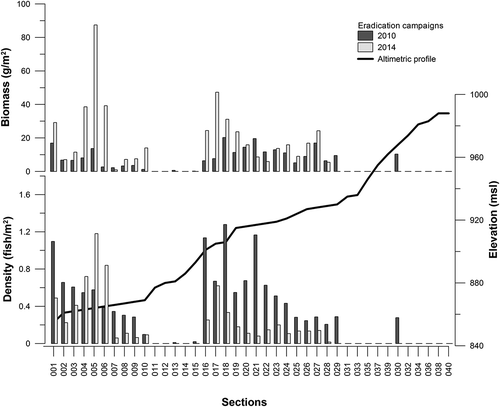
Several electrofishing passages were made in each section to ensure capture of all the brown trout in the Ermolinus population. In 2010, during the first pass 64.0% of the fish removed were adults, and 28.2% were of age 0+. The same trend was observed in the eradication campaign of 2014, with 30.2% (age 0+) and 57.2% (adults) of brown trout removed during the first pass. In subsequent passes, age 0+ fish were more numerous than adults, a bias that decreased progressively and that had almost vanished between the seventh and eighth passes (2010 and 2014, respectively; ).
Table I. Eradication campaigns, 2010 and 2014. Removal in absolute numbers and percentage of catches (in parentheses) for age classes (0+ and adult), and total in 10 passages of electrofishing; estimated population (± standard deviation) and capture efficiency (CE) by multi-depletion model.
This methodology was validated using the multiple depletion method comparing the actual catch and the estimated population, which showed a total capture efficiency of over 0.996 in 2010 and 0.998 in 2014 ().
Restocking of native trout and population growth model
After the depopulation had been completed the eradicated part of the stream was stocked with native trout bred from genetically characterised breeders. In 2010, in order to study the efficiency of the eradication, and the barrier performance, only 100 parr native trouts were stocked (sections 001–010; R1, ).
Table II. Activity (R: repopulation, M: monitoring, P: estimated population) and sample data for Sardinian trout growth modelling.
Two years after stocking (2012), due to the low contribution of rainwater in the summer, eight sections were randomly selected and monitored. In total, 12 trout were collected with genetic characteristics of native trout (AD *100/*100) that matched an estimated population of 60 specimens. The stocked trout showed a mean survival rate (S) of 0.77 per year. Native trout ranged in TL from 18.2 to 20.5 cm with a mean length of 19.3 cm and an overall mean weight of 77.5 g (corresponding to age classes 2+).
Due to the barrier outage, a second phase of stocking activities were conducted in 2014 and 2015 (R2 and R3, ) using parr and adult trout (from age 0+ to 3+) obtained from the same fish farm. In October 2014, 50 fish from age 2+ to 3+ (TL 18.5–35.5 cm) were released in pools in every section, while the parr stage (0+) and sub-adults (1+) were released in pools characterised by low water speed (< 0.2 m/s) and water depths less than 15 cm.
In 2015, a further 85 fish were stocked in batches of three in every section. Subsequent distributions of the stocked fish (July 2015), determined by electrofishing surveys and by reading tags, showed a survival rate (S) of 0.64 with a density of 0.022 ind/m2 (monitoring campaign M2). In this monitoring campaign parr trout (age 0+) were observed consisting of 16 ± 10 individuals with an average reproductive rate (R) of 0.5 (parr trout 0+/adults). During the third monitoring campaign (M3), conducted in July 2016, a total density of 0.103 ind/m2 was calculated and a survival rate (S) of 0.58 was observed. In this year, the reproductive rate increased to 3.2 ± 1 (parr trout 0+/adults) and a total estimated population of 223 fish. Using these parameters (S = 0.6 and R = 3.2), a population of 323 trout was estimated for the year 2017 (P1). This first result was overestimated in M4, conducted in June 2017. Indeed, during M4 a total density of 0.138 ind/m2 was estimated with an increase of 34% between 2016 and 2017. The same reproductive rate (R = 3.1) was registered but with a decrease in the survival rate of 12% (S = 0.51).
Considering that the river was estimated to withstand a maximum sustainable population of ~1355 trout, an exponential growth model was established using a reproductive rate (R) of 3.2 parr trout 0+/adults and a mean survival rate (S) of 51%. The model predicts, from 2018, a mean growth rate of 12% that will increase until 2024 (P8) with 638 trout, approaching 50% of the carrying capacity.
Discussion
The Ermolinus stream was chosen to perform and assess the first isolation management as a conservation strategy for brown native trout in Mediterranean headwater streams. Artificial barriers are important management tools to prevent upstream movement of non-native aquatic organisms into streams with native fish populations. Reducing the connectivity between invaded and non-invaded water bodies by constructing physical barriers is an obvious way to limit the distribution of aquatic invasive species (AIS; Buley et al. Citation2017). The obstructions used to isolate headwater sections of streams can be either natural or artificial. Natural barriers (waterfalls and cascades) can sometimes be used to block the upstream movement of non-native fish (Gresswell & Hendricks Citation2007). Where natural barriers are absent or ineffective, gabion, culvert barriers (Thompson & Rahel Citation1998), and electric or bubble barriers (Clarkson Citation2004; Dawson et al. Citation2006) can be set in place to isolate the two populations. Placement of such structures minimises the risk of fish moving along the river, mixing populations (Novinger & Rahel Citation2003). The electric barrier to prevent upstream movements of non-native fish was first successfully used in North America in the 1950s (Applegate et al. Citation1952).
Our study on Ermolinus stream suggests that introgressed trout present downstream cannot move through the electric barrier. Upstream recolonisation by introgressed brown trouts was detected in November 2013 when several components of the barrier were damaged by a lightning strike. In general, the sources of electrical barrier outage included the major categories of mechanical failure (damage from lightning strikes and manufacturing flaws) and human error (failure to adhere to component maintenance and replacement schedules) (Verrill & Berry Citation1995; Swink Citation1999; Clarkson Citation2004).
The genetic analysis of the Ermolinus stream trout population before the restoration project showed a high degree of introgression. Nucleotide identity-test of mitochondrial DNA (mtDNA CR) and analysis of nuclear DNA (locus LDH C1*) showed the presence of non-native individuals of domestic origin (29%) and a high level of genetic hybridisation (65%), reflecting a reduction in the genetic integrity of the native trout population (Sabatini et al. Citation2011). Therefore, considering the high degree of introgression, all the trout upstream of the barrier were removed. In some studies, chemical or mechanical means have been used to remove non-native trout. Chemical methods include the use of the piscicides antimycin, rotenone or ozone, and have mainly been used to eradicate exotic fish populations, or fish which may spread disease, in order to protect threatened or endangered species (Gresswell Citation1991; Demong Citation2001; Allen et al. Citation2006; Buley et al. Citation2017). Mechanical methods include the use of gill netting (Knapp & Matthews Citation1998; Tiberti et al. Citation2017), and repeated electrofishing (Kulp & Moore Citation2000; Carmona-Catot et al. Citation2010; Caudron et al. Citation2011). The use of chemical methods for eradication is often controversial due to harmful effects on non-target species, and lethal effects on aquatic invertebrates and the larval forms of amphibians (Mangum & Madrigal Citation1999; Ling Citation2003; Buley et al. Citation2017). Chemicals were not employed in this study. This study was conducted in a protected area of Sardinia, home to many extremely important species such as the endemic mountain newt Euproctus platycephalus (Amphibia: Urodela), an endangered species listed in Appendix II of the Bern Convention, on Annex IV of the EU Habitats Directive and on regional legislation (Regional Law n. 23/1998 (art. 5, c. 3) (Vignoli et al. Citation2016). Mechanical removal methods, such as gill netting or repeated electrofishing, allow selective species removal and are a viable alternative to chemical methods. Netting can reduce populations of invasive trout, but can never eliminate them completely (Knapp & Matthews Citation1998) while, as Growns et al. (Citation1996) have shown, electrofishing collects 30 times more fish per unit of fishing time than gill netting.
For the above reasons, we chose electrofishing as the mitigation tool to remove the introgressed brown trout population from the stream above the barrier. The eradication campaigns required two different fishing efforts (216 and 56 hours for 2010 and 2014, respectively). The higher fishing effort registered in the first campaign was a consequence of (a) a different eradication period and (b) a low population number, primarily composed of adult specimens in 2014. Capture data by age class showed that the small fish (0+ age class) were more difficult to catch, as demonstrated by Caudron and Champigneulle (Citation2011). The capture efficiency analysis conducted during the two campaigns confirmed this observation and showed achieved total capture rates of over 99% for 0+ and 100% for adult trout. These results were in accordance with Knapp and Matthews (Citation1998), and Tiberti et al. (Citation2017), hypothesising that the eradications can be considered concluded after 1 year without fish capture. Overall, the electrofishing method of fish capture and removal was shown to be generally applicable to headstreams in Mediterranean climatic zones prone to summer droughts, only if matched to the use of the electric barrier.
The capture data gathered during fish removal campaigns showed the distribution of the species in this area. Two areas of the stream held particularly abundant trout, and in two other areas fish were rare or absent. The fish preferred parts of the stream where the gradient and the likelihood of summer drought were low. In accordance with (Armstrong et al. Citation2003) we observed that adult fish are present in pools and riffles, while parr trout were observed in the smallest pools characterised by low water speed (< 0.2 m/s) and water depths of less than 15 cm.
The stocked native trout caught were dominated by stock-size fish (mean length), which correspond to age class 2+ as expected. The lack of parr trout confirms the efficiency of the electrofishing method to eradicate the trout populations. Subsequent restocking phases were conducted in 2014 and 2015 using tagged trouts (0+ to 3+). The restocking practice has already been conducted for a number of endangered salmonids (Crivelli et al. Citation2000; Caudron et al. Citation2009). We preferred to restock with sexually mature adults instead of parr trout in order to reduce the recovery time of the population. Furthermore, the use of sexually mature adults reduces the risk of extinction of the species (Todd et al. Citation2004; Hilderbrand Citation2011).
The restocked populations have been monitored every year starting from July 2015. The monitoring campaigns in 2015 and 2016 showed good survival (S = 0.6) and reproduction (R = 3.1). The high percentage of survival rate is due to the absence of a density-dependence mechanism that did not negatively affect the survival rate.
Successful breeding was recorded starting from the summer of 2015. In 2017, a decrease in the survival rate of about 12% was observed as a consequence of downstream migration of a few adult trout. Therefore, S = 0.51 and R = 3.1 were used to set up a predictive growth model in order to predict a mean growth rate of 12% that will increase until 2024, approaching 50% of the carrying capacity of the stream. From 2024, the population will grow exponentially and the basic model parameters may be subject to change. This will need to be re-established when the population approaches the carrying capacity and the growth rate decreases. Even with an estimation of the attainment of carrying capacity of 1352 trout in the year 2030, a self-sustaining population has already been registered after only two restocking events. This predictive growth model includes a large amount of natural variation; therefore, we suggest it is an operative tool for the restoration of native brown trout population in Mediterranean type stream. However, its application to other fish restoration programmes in other streams and populations should be tested for those particular situations.
Results from the present study allow us to make recommendations for future conservation eradication and repopulation projects to restore the rivers in the Mediterranean area. The management and conservation of wild native trout require that (1) the reintroduction of native species should be conducted within their historical range; (2) good ecological and trophic conditions are available to ensure the survival of reintroduced fish; (3) the population must be adversely affected by genetic introgression to justify eradication and reintroduction of native species; (4) the eradication campaign should be conducted in low flow conditions when the population can be properly removed using electrofishing; (5) the morphology of the river should be adequate such that structures can be built to isolate the portion of river chosen for restocking; and may also require (6) complementary measures for continued maintenance of the barrier in order to prevent repopulation by introgressed brown trout. However, to ensure a successful management programme in Ermolinus stream, the status of the restored native trout population should be continually monitored.
Seven years from the beginning of the restoration project, the stream is managed and controlled by the local forest guard. The project will continue to focus these efforts on the translocation of wild native trout in order to reduce the time for the establishment of self-sustaining populations.
Acknowledgements
This project was conducted by the Department of Life and Environment Sciences at the University of Cagliari, with the collaboration of Forest Management Agency of Sardinia (Fo.Re.S.T.A.S). Funding was provided by the “Assessorato Della Difesa dell’Ambiente” and was coordinated at regional level “Servizio Tutela Della Natura” (REP. RAS 22001-1 D.A. 4/10/2013). The native trout were provided by the hatchery located in Sadali (Sardinia) and managed by the “Comunità Montana Sarcidano Barbagia di Seulo”. We are especially grateful to Laura Cappai, Gianluca Orrù and Laura Angius from “Servizio Tutela della Natura” for their support throughout this project. We also acknowledge Mauro Marongiu, Bastiano Moi and all the other Montarbu forest workers for their help during the activities.
Disclosure statement
No potential conflict of interest was reported by the authors.
Additional information
Funding
References
- Allen Y, Kirby S, Copp GH, Brazier M. 2006. Toxicity of rotenone to topmouth gudgeon Pseudorasbora parva for eradication of this non-native species from a tarn in Cumbria, England. Fisheries Management and Ecology 13:337–340. DOI:10.1111/j.1365-2400.2006.00499.x.
- Almodóvar A, Nicola GG. 1998. Assessment of a brown trout Salmo trutta population in the River Gallo (central Spain): Angling effects and management implications (Salmonidae). Italian Journal of Zoology 65:539–543. DOI:10.1080/11250009809386881.
- Applegate VC, Smith BR, Nielson WL. 1952. Use of electricity in the control of sea lampreys: Electromechanical weirs and traps and electrical barriers. U.S. Fish and Wildlife Service Special Scientific Report: Fisheries 92:1–58.
- Araguas RM, Sanz N, Fernàndez R, Utter FM, Pla C, Garcìa-Marìn JL. 2008. Genetic refuges for a self-sustained fishery: Experience in wild brown trout populations in the eastern Pyrenees. Ecology of Freshwater Fish 17(Suppl. 4):610–616. DOI:10.1111/j.1600-0633.2008.00312.x.
- Araguas RM, Sanz N, Fernàndez R, Utter FM, Pla C, Garcìa-Marìn JL. 2009. Role of genetic refuges in the restoration of native gene pools of brown trout. Conservation Biology 23:871–878. DOI:10.1111/j.1523-1739.2008.01144.x.
- Armstrong JD, Kemp PS, Kennedy CJ, Ladle M, Milner NJ. 2003. Habitat requirements of Atlantic salmon and brown trout in rivers and streams. Fisheries Research 62:143–170. DOI:10.1016/S0165-7836(02)00160-1.
- Avenetti LD, Robinson AT, Cantrell CJ. 2006. Short-term effectiveness of constructed barriers at protecting Apache trout. North American Journal of Fisheries Management 26:213–216. DOI:10.1577/M04-092.1.
- Bernatchez L. 2001. The evolutionary history of brown trout (Salmo trutta L.) inferred from phylogeographic, nested clade, and mismatch analyses of mitochondrial Dna variation. Evolution 55(2):351–379. DOI:10.1111/j.0014-3820.2001.tb01300.x.
- Bernatchez L, Guyomard R, Bonhomme F. 1992. DNA sequence variation of the mitochondrial control region among geographically and morphologically remote European brown trout Salmo trutta populations. Molecular Ecology 1:161–173. DOI:10.1111/j.1365-294X.1992.tb00172.x.
- Berrebi P, Jesenšek D, Crivelli AJ. 2017. Natural and domestic introgressions in the marble trout population of Soča River (Slovenia). Hydrobiologia 785:277–291. DOI:10.1007/s10750-016-2932-2.
- Berrebi P, Povz M, Jesensek D, Cattaneo-Berrebi G, Crivelli AJ. 2000. The genetic diversity of native, stocked and hybrid populations of marble trout in the Soca river, Slovenia. Heredity 85:277–287. DOI:10.1046/j.1365-2540.2000.00753.x.
- Buley RP, Hasler CT, Tix JA, Suski CD, Hubert TD. 2017. Can ozone be used to control the spread of freshwater Aquatic Invasive Species? Management of Biological Invasions 8:13–24. DOI:10.3391/mbi.2017.8.1.02.
- Burnet AMR. 1969. An examination of the use of scales and fin rays for age determination of brown trout (Salmo trutta L.). New Zealand Journal of Marine and Freshwater Research 3:147–151. DOI:10.1080/00288330.1969.9515282.
- Caputo Barucchi V, Carosi A, Giovannotti M, La Porta G, Splendiani A, Lorenzoni M 2015. Life+ Trout Project (LIFE12 NAT/IT/0000940) for the recovery and conservation of Mediterranean trout (Salmo trutta complex) in the central Apennines (Italy). XV European Congress of Ichthyology 7-11 September. Porto (Portugal). DOI:10.3389/conf.fmars.2015.03.00101.
- Caputo V, Giovannotti M, Nisi Cerioni P, Caniglia ML, Splendiani A. 2004. Genetic diversity of brown trout in central Italy. Journal of Fish Biology 65:403–418. DOI:10.1111/j.0022-1112.2004.00458.x.
- Carle FL, Strub MR. 1978. A new method for estimating population size from removal data. Biometrix 34:621–630. DOI:10.2307/2530381.
- Carmona-Catot GP, Moyle B, Aparicio E, Crain PK, Thompson LC, García-Berthou E. 2010. Brook trout removal as a conservation tool to restore eagle lake rainbow trout. North American Journal of Fisheries Management 30:1315–1323. DOI:10.1577/M10-077.1.
- Caudron A, Champigneulle A. 2011. Multiple electrofishing as a mitigate tool for removing nonnative Atlantic brown trout (Salmo trutta L.) threatening a native Mediterranean brown trout population. European Journal of Wildlife Research 57:575–583. DOI:10.1007/s10344-010-0468-8.
- Caudron A, Champigneulle A, Guyomard R, Largiadèr CR. 2011. Assessment of three strategies practiced by fishery managers for restoring native brown trout (Salmo trutta) populations in Northern French Alpine Streams. Ecology of Freshwater Fish 20:478–491. DOI:10.1111/j.1600-0633.2010.00458.x.
- Caudron A, Champigneulle A, Largiadèr CR, Launey S, Guyomard R. 2009. Stocking of native Mediterranean brown trout (Salmo trutta) into French tributaries of Lake Geneva does not contribute to lake-migratory spawners. Ecology of Freshwater Fish 18:585–593. DOI:10.1111/j.1600-0633.2009.00374.x.
- Clarkson RW. 2004. Effectiveness of electrical fish barriers associated with the central Arizona Project. North American Journal of Fisheries Management 24:94–105. DOI:10.1577/M02-146.
- Cortey M, Garcia-Marin J-L. 2002. Evidence for phylogeographically informative sequence variation in the mitochondrial control region of Atlantic brown trout. Journal of Fish Biology 60:1058–1063. DOI:10.1111/jfb.2002.60.issue-4.
- Cortey M, Vera M, Pla C, Garcia-Marin JL. 2009. Northern and Southern expansions of Atlantic brown trout (Salmo trutta) populations during the Pleistocene. Biological Journal of the Linnean Society 97:904–917. DOI:10.1111/j.1095-8312.2009.01220.x.
- Cottiglia M. 1968. La distribuzione della ittiofauna dulciacquicola in Sardegna. Rivista di Idrobiologia 7:64–116.
- Crivelli AJ, Poizat G, Berrebi P, Jesensek D, Rubin JF. 2000. Conservation biology applied to fish: The example of a project for rehabilitating the Marble trout (Salmo marmoratus) in Slovenia. Cybium 24:211–230.
- Dawson HD, Reinhardt UG, Savino JF. 2006. Use of electric or bubble barriers to limit the movement of Eurasian ruffe (Gymnocephalus cernuus). Journal of Great Lakes Research 32:40–49. DOI:10.3394/0380-1330(2006)32[40:UOEOBB]2.0.CO;2.
- Demong L. 2001. The use of rotenone to restore native brook trout in the Adirondack Mountains of New York–An overview. In: Caiteux RL, Demong L, Finlayson B, Horton W, McClay W, Schnick RA, editors. Rotenone in fisheries: are the rewards worth the risk?. Bethesda, MD: American Fisheries Society, Trends In Fisheries Science And Management 1. pp. 29–36.
- Dudgeon D, Arthington AH, Gessner MO, Kawabata ZI, Knowler DJ, Lévêque C, Naiman RJ, Prieur-Richard AH, Soto D, Stiassny MLJ, Sullivan CA. 2006. Freshwater biodiversity: Importance, threats, status and conservation challenges. Biological Reviews of the Cambridge Philosophical Society 81:163–182. DOI:10.1017/S1464793105006950.
- Fruciano C, Pappalardo AM, Tigano C, Ferrito V. 2014. Phylogeographical relationships of Sicilian brown trout and the effects of genetic introgression on morphospace occupation. Biological Journal of the Linnean Society 112:387–398. DOI:10.1111/bij.12279.
- Gheorghiu C, Hanna J, Smith JW, Smith DS, Wilkie MP. 2010. Encapsulation and migration of PIT tags implanted in brown trout (Salmo trutta L.). Aquaculture 298:350–353. DOI:10.1016/j.aquaculture.2009.10.004.
- Gratton P, Allegrucci G, Sbordoni V, Gandolfi A. 2014. The evolutionary jigsaw puzzle of the surviving trout (Salmo trutta L. complex) diversity in the italian regionion a multilocus bayesian approach. Molecular Phylogenetics and Evolution 79:292–304. DOI:10.1016/j.ympev.2014.06.022.
- Gresswell R. 1991. Use of antimycin for removal of brook trout from a tributary of Yellowstone Lake. North American Journal of Fisheries Management 11:83–90. DOI:10.1577/1548-8675(1991)011<0083:UOAFRO>2.3.CO;2.
- Gresswell RE, Hendricks SR. 2007. Population-scale movement of coastal cutthroat trout in a naturally isolated stream network. Transactions of the American Fisheries Society 136:238–253. DOI:10.1577/T05-196.1.
- Growns IO, Pollard DA, Harris JH. 1996. A comparison of electric fishing and gillnetting to examine the effects of anthropogenic disturbance on riverine fish communities. Fisheries Management and Ecology 3:13–24. DOI:10.1111/j.1365-2400.1996.tb00126.x.
- Hilderbrand RH. 2011. Simulating supplementation strategies for restoring and maintaining stream resident cutthroat trout populations. North American Journal of Fisheries Management 22:879–887. DOI:10.1577/1548-8675(2002)022<0879.
- Knapp RA, Matthews KR. 1998. Eradication of nonnative fish by gill-netting from a small mountain lake in California. Restoration Ecology 6:207–213. DOI:10.1111/j.1526-100X.1998.06212.x.
- Kottelat M, Freyhof J. 2007. Handbook of European freshwater fishes. Berlin: Kottelat M and J. Freyhof editors.
- Kruse CG, Hubert WA, Rahel FJ. 2001. An assessment of headwater isolation as a conservation strategy for cutthroat trout in the Absaroka Mountains of Wyoming. Northwest Science 75:1–11.
- Kulp MA, Moore SE. 2000. Multiple electrofishing removals for eliminating rainbow trout in a small Southern Appalachian stream. North American Journal of Fisheries Management 20:259–266. DOI:10.1577/1548-8675(2000)020<0259:MERFER>2.0.CO;2.
- Ling N. 2003. Rotenone - A review of its toxicity and use for fisheries management. Science for Conservation 211:1–40.
- Lockwood RN, Schneider JC. 2000. Stream fish population estimates by mark- and-recapture and depletion methods. In: Schneider JC, editor. Manual of fisheries survey methods II: with periodic updates. Ann Arbor, MI: Michigan, Department for Natural Resources, Fisheries Special Report 25. pp. 1–14.
- Mangum FA, Madrigal JL. 1999. Rotenone effects on aquatic macroinvertebrates of the Strawberry River, Utah: A five-year summary. Journal of Freshwater Ecology 14:125–135. DOI:10.1080/02705060.1999.9663661.
- Massidda P. 1995. Salmo (trutta) macrostigma in Sardegna. Biologia Ambientale 5:40–43.
- Massidda P, Cau A, Conti G, Loddo G. 2008. Pesci d’acqua dolce della Sardegna I. Cagliari: Aìsara. pp. 96.
- Massidda P, Sabatini A, Davini M, Conti G, Loddo G, Cau A. 1996. Nuovi dati sulla distribuzione dell’ittiofauna d’acqua dolce in Sardegna. In: Atti del VI convegno nazionale associazione ittiologi acque dolci. Varese Ligure.
- McMeel OM, Hoey EM, Ferguson A. 2001. Partial nucleotide sequences, and routine typing by polymerase chain reaction-restriction fragment length polymorphism, of the brown trout (Salmo trutta) lactate dehydrogenase, LDH-C1*90 and*100 alleles. Molecular Ecology 10:29–34. DOI:10.1046/j.1365-294X.2001.01166.x.
- Nonnis Marzano F, Corradi N, Papa R, Tagliavini J, Gandolfi G. 2003. Molecular evidence for introgression and loss of genetic variability in Salmo (trutta) macrostigma as a result of massive restocking of Apennine populations (Northern and Central Italy). Environmental Biology of Fishes 68:349–356. DOI:10.1023/B:EBFI.0000005762.81631.fa.
- Novinger DC, Rahel F. 2003. Isolation managment with artifical barriers as a conservation strategy for cutthroat trout in headwater streams. Conservation Biology 17:772–781. DOI:10.1046/j.1523-1739.2003.00472.x.
- Orrù F, Deiana AM, Cau A. 2010. Introduction and distribution of alien freshwater fishes on the island of Sardinia (Italy): An assessment on the basis of existing data sources. Journal of Applied Ichthyology 26:46–52. DOI:10.1111/j.1439-0426.2010.01501.x.
- Pauly D, Moreau J, Abad N. 1995. Comparison of age-structured and length-converted catch curves of brown trout Salmo trutta in two French rivers. Fisheries Research 22:197–204. DOI:10.1016/0165-7836(94)00323-O.
- Pomini PF. 1940. Ricerche sul Salmo macrostigma. Bollettino di Pesca, di Piscicoltura e di Idrobiologia:1–63.
- Poteaux C, Bonhomme F, Berrebi P. 1998. Differences between nuclear and mitochondrial introgressions of brown trout populations from a restocked main river and its unrestocked tributary. Biological Journal of the Linnean Society 63:379–392. DOI:10.1111/j.1095-8312.1998.tb01524.x.
- Querci G, Pecchioli E, Leonzio C, Frati F, Nardi F. 2013. Molecular characterization and hybridization in Salmo (trutta) macrostigma morphotypes from Central Italy. Hydrobiologia 702:191–200. DOI:10.1007/s10750-012-1320-9.
- Reid DM, Anderson DM, Henderson BA. 2001. Restoration of lake trout in parry sound, lake huron. North American Journal of Fisheries Management 21:156–169. DOI:10.1577/1548-8675(2001)021<0156:ROLTIP>2.0.CO;2.
- Rondinini C, Battistoni A, Peronace V, Teofili C. 2013. Lista Rossa IUCN dei Vertebrati Italiani. Comitati Italiano IUCN e Ministero dell’Ambiente e della Tutela del Territorio e del Mare. Roma.
- Sabatini A, Cannas R, Follesa MC, Palmas F, Manunza A, Matta G, Pendugiu AA, Serra P, Cau A. 2011. Genetic characterization and artificial reproduction attempt of endemic Sardinian trout Salmo trutta L., 1758 (Osteichthyes, Salmonidae): Experiences in captivity. Italian Journal of Zoology 78:20–26. DOI:10.1080/11250003.2010.497171.
- Sabatini A, Orrù F, Cannas R, Serra P, Cau A. 2006. Conservation and management of Salmo (trutta) macrostigma, in Sardinia freshwaters: First result of genetic characterization. Journal of Freshwater Biology, Quaderni ETP/34/2006:335–340.
- Saunders DL, Meeuwig JJ, Vincent ACJ. 2002. Freshwater protected areas: Strategies for conservation. Conservation Biology 16:30–41. DOI:10.1046/j.1523-1739.2002.99562.x.
- Schöffmann J, Susnik S, Snoj A. 2007. Phylogenetic origin of Salmo trutta L 1758 from Sicily, based on mitochondrial and nuclear DNA analyses. Hydrobiologia 575:51–55. DOI:10.1007/s10750-006-0281-2.
- Servanty S, Converse SJ, Bailey LL. 2014. Demography of a reintroduced population: Moving toward management models for an endangered species, the Whooping Crane. Ecological Applications 24:927–937. DOI:10.1890/13-0559.1.
- Splendiani A, Ruggeri P, Giovannotti M, Pesaresi S, Occhipinti G, Fioravanti T, Lorenzoni M, Nisi Cerioni P, Caputo Barucchi V. 2016. Alien brown trout invasion of the Italian peninsula: The role of geological, climate and anthropogenic factors. Biological Invasions 18:2029–2044. DOI:10.1007/s10530-016-1149-7.
- Susnik S, Schöffmann J, Weiss S. 2005. Genetic verification of native brown trout from the Persian Gulf (Catak Cay River, Tigris basin). Journal of Fish Biology 67:879–884. DOI:10.1111/j.1095-8649.2005.00780.x.
- Swink W. 1999. Effectiveness of an electrical barrier in blocking a sea lamprey spawning migration on the Jordan River, Michigan. North American Journal of Fisheries Management 19:397–405. DOI:10.1577/1548-8675(1999)019<0397:EOAEBI>2.0.CO;2.
- Thompson P, Rahel F. 1998. Evaluation of artificial barriers in small Rocky Mountain streams for preventing the upstream movement of brook trout. North America Journal of Fisheries Society 18:206–210. DOI:10.1577/1548-8675(1998)018<0206:EOABIS>2.0.CO;2.
- Tiberti R, Nelli L, Brighenti S, Iacobuzio R, Rolla M. 2017. Spatial distribution of introduced brook trout Salvelinus fontinalis (Salmonidae) within alpine lakes: Evidences from a fish eradication campaign. The European Zoological Journal 84:73–88. DOI:10.1080/11250003.2016.1274436.
- Todd CR, Nicol SJ, Koehn JD. 2004. Density-dependence uncertainty in population models for the conservation management of trout cod, Maccullochella macquariensis. Ecological Modelling 171:359–380. DOI:10.1016/j.ecolmodel.2003.06.002.
- Vera M, Cortey M, Sanz N, Garcia-Marin JL. 2010. Maintenance of an endemic lineage of brown trout (Salmo trutta) within the Duero river basin. Journal of Zoological Systematics and Evolutionary Research 48:181–187. DOI:10.1111/j.1439-0469.2009.00547.x.
- Verrill D, Berry JC. 1995. Effectiveness of an electrical barrier and lake drawdown for reducing common carp and bigmouth buffalo abundances. North American Journal of Fisheries Management 15:137–141. DOI:10.1577/1548-8675(1995)015<0137:EOAEBA>2.3.CO;2.
- Vignoli L, Macale D, Luiselli L, Lecis R, Casula P. 2016. Are conservation assessments of threatened species reliable? Updated distribution of the Endangered Sardinian newt Euproctus platycephalus and implications for Red List assessments of Italian amphibians. Oryx:1–7. DOI:10.1017/S0030605315001416.
- Vincenzi S, Crivellì AJ, Jesensek D, Rubin JF, De Leo GA. 2007. Early survival of marble trout Salmo marmoratus: Evidence for density dependence?. Ecology of Freshwater Fish 16:116–123. DOI:10.1111/j.1600-0633.2007.00203.x.
- Vincenzi S, Crivelli AJ, Jesensek D, Rubin JF, Poizat G, De Leo GA. 2008. Potential factors controlling the population viability of newly introduced endangered marble trout populations. Biological Conservation 141:198–210. DOI:10.1016/j.biocon.2007.09.013.
- Zaccara S, Trasforini S, Antognazza CM, Puzzi C, Britton JR, Crosa G. 2015. Morphological and genetic characterization of Sardinian trout Salmo cettii Rafinesque, 1810 and their conservation implications. Hydrobiologia 760:205–223. DOI:10.1007/s10750-015-2322-1.