Abstract
The deleterious effects of anthropogenic noise on animal communication are nowadays recognised, not only in urban environments but also in terrestrial habitats and along coasts and in open waters. Yet, the assessment of short- and long-term exposure consequences of anthropogenic noise in marine organisms remains challenging, especially in fish and invertebrates. Males of the Mediterranean damselfish Chromis chromis vocalise and perform visual displays (multimodal communication) to attract mates. The frequency-range of courtship vocalisations overlaps with low-frequency noise generated by maritime activities, resulting in a reduced detection distance among conspecifics. We quantified the number of courtship-related visual displays performed by males living in areas with different levels of maritime traffic. We also tried to manipulate ambient noise in the field to test male short-term response to increased noise levels. Males living in busier areas (near to a harbour) performed significantly more visual displays than those living in less congested areas. When exposed to artificially-increased ambient noise level (playback of boat noise), males did not adjust the number of visual displays accordingly. Yet, we note how assessing the actual effect of maritime traffic in marine populations in their natural environments is particularly difficult, as the effects of boat noise cannot be easily disentangled from a variety of other intrinsic or environmental factors, discussed in the paper. We thus present suggestions to obtain more robust analyses of variations of courtship behaviours in territorial fishes. We hope this will facilitate a further understanding of the potential long-term effects of anthropogenic noise, whose analyses should be prioritised in the context of environmental impact assessment, resource management and biodiversity conservation.
Introduction
Coastal and offshore human activities often lead to local increments of ambient noise levels, polluting the marine soundscape (Slabbekoorn et al. Citation2010; Radford et al. Citation2014; Whitfield & Becker Citation2014; Dooling et al. Citation2015; Hawkins & Popper Citation2018; Putland et al. Citation2018). To better understand the short and long-term effects of marine acoustic pollution, not only at the individual but also at the population and ecosystem level (Kunc et al. Citation2016; Nabe-Nielsen et al. Citation2018), more underwater acoustic ecological studies should be performed (Slabbekoorn et al. Citation2010; Brumm Citation2014; Radford et al. Citation2014; Slabbekoorn Citation2016). Indeed, even though these topics are gaining interest and conservation relevance (Williams et al. Citation2015; Aguilar de Soto et al. Citation2016; Greggor et al. Citation2016; Gordon et al. Citation2018), and the focus is broadening from an initial concern targeted mainly to marine mammals (Weilgart Citation2007; Southall et al. Citation2008; Ellison et al. Citation2011; Miller et al. Citation2015), investigations on acoustic behaviours of aquatic organisms in general and the detrimental effects of man-made noise in particular remain challenging in marine and freshwater ecosystems (Lobel et al. Citation2010; Bolgan et al. Citation2016; Mickle & Higgs Citation2017; Hasan et al. Citation2018; Linke et al. Citation2018). Studies on the effects of anthropogenic noise on invertebrates are scant (Wale et al. Citation2013; Williams et al. Citation2015; Aguilar de Soto Citation2016; André et al. Citation2016; Tidau & Briffa Citation2016) and more research is also needed on fish (see the recent review by Popper & Hawkins Citation2019), especially on wild populations in natural habitat, which is extremely difficult (Slabbekoorn Citation2016). Finally, as in many behavioural responses (Siegenthaler et al. Citation2018), a certain level of plasticity to address anthropogenic noise is expected not only at the interspecific and interpopulation level, but also at the intrapopulation level based on inter and intra-individual variability and time of exposure (Radford et al. Citation2015; Harding et al. Citation2018).
In light of the results obtained from studies performed in terrestrial environments (e.g., Barber et al. Citation2009; Francis & Barber Citation2013), researchers suggest that similar effects should be expected in aquatic environments, especially with regards to fishes (Dooling et al. Citation2015). However, caution should be taken, given the differences existing between terrestrial and aquatic media in terms of physical properties (McGregor et al. Citation2013) and the different adaptations to sound detection and acoustic communication in different taxa (Wong Citation2014). Even when sound pollution affects fish communication, the main question is “how much does it matter” (Brumm Citation2014)? In other words: can fish respond in a phenotypically plastic way to noise stressors? How and how well can fish adjust their communication in response to anthropogenic impacts? Can short-term effects, at the individual level, impact population dynamics in the long term?
In the water, anthropogenic noise can be generated intentionally, for example to prevent harmful interactions between marine mammals and fishery activities (Waples et al. Citation2013) or during seismic studies (Popper et al. Citation2005) and sonar applications (Hildebrand Citation2009). More often, noise is the by-product of various activities, including wind farm plants (Wahlberg & Westerberg Citation2005), underwater explosions, pile-driving operations (Haelters et al. Citation2013) or, most commonly, maritime traffic (Popper & Hastings Citation2009). Noise generated by ships and boats mainly falls in the lower frequency range of the acoustic spectrum (1–1000 Hz) which is also the range at which all fish studied to date are able to hear (<50 to 1000–1500 Hz; Popper Citation2003; Popper & Fay Citation2011; Radford et al. Citation2014; Popper & Hawkins Citation2019) even though some fish reach 4000–8000 Hz (Lobel et al. Citation2010; Ladich & Fay Citation2013).
Fish use sounds (production and/or detection) in multiple ways (Bass & Ladich Citation2008; Ladich Citation2013; Popper & Hawkins Citation2019): specific sounds can be used during migrations and as directional orientation clues for larvae to settle (Tolimieri et al. Citation2004) but mostly sounds are received and produced to acquire and transmit information. Many fish species actively produce sounds to communicate the presence of predators or food to their conspecifics (Bessey & Heithaus Citation2013), during aggressive behaviours (Millot et al. Citation2011), to defend their territories (Myrberg Citation1997; Pereira et al. Citation2014) and to attract mates: indeed, a very important context during which teleosts vocalise is courtship (e.g., Amorim & Neves Citation2007; Parmentier et al. Citation2010; Amorim et al. Citation2012; Maruska et al. Citation2012; Casaretto et al. Citation2015).
Given its vocal behaviours, the family Pomacentridae (damselfishes) has been an interesting study system for acoustic studies (Myrberg & Spires Citation1972; Mann & Lobel Citation1997; Amorim Citation2006; Kasumyan Citation2008; Ladich Citation2013; Weimann et al. Citation2018), in coral reefs (Lobel et al. Citation2010; Parmentier et al. Citation2010; Fakan & McCormick Citation2019) and in the Mediterranean (Picciulin et al. Citation2002, Citation2010; Codarin et al. Citation2009; Bracciali et al. Citation2012). Damselfishes are an abundant component of coral and rocky reefs, where they play a key role in the trophic functioning of the ecosystem (Bracciali et al. Citation2012; Pinnegar Citation2018). Chromis chromis Linnaeus, 1758, is the only native pomacentrid in the Mediterranean Sea (Allen Citation1991); it is a small damselfish commonly found in shoals near rocky reefs or above seagrass meadows at depths between 3 and 30 m (Quignard & Pras Citation1986). Mediterranean damselfish are characterised by localised spawning areas and habitat use. Their life history traits include the production of benthic eggs and short-lived pelagic larvae (18–19 days; Raventós & Macpherson Citation2001). These features and the marked territoriality of adults are expected to favour population isolation and geographic genetic structure (Domingues et al. Citation2005). During the summer, males colonise nests and guard them until the end of the reproductive season. They court females by vocalising and performing specific behavioural patterns, i.e., signal jumps (“the male swims rapidly upwards for 1–3 m before rotating downwards and returning to the nest”; Laglbauer et al. Citation2017) as visual displays, in order to receive egg deposition (Picciulin et al. Citation2002, Citation2010 and references therein). The frequency range of their vocalisations overlaps with low frequency noise generated by boats and ships, resulting in a marked reduction (up to 100 times) of the detection distance among conspecifics (Mann & Lobel Citation1997; Codarin et al. Citation2009; Wysocki et al. Citation2009).
This study aimed to investigate variations in the courtship strategies of the Mediterranean damselfish in three different areas in Sicily, Italy. Literature suggests that the high levels of ambient noise (sensu Hildebrand Citation2009: “the sound field against which signals must be detected”) caused by navigation make it more difficult for females to hear male vocalisations (Codarin et al. Citation2009). Thus, we predicted that males living in a more congested (thus possibly noisier) environment would on average perform more visual displays (VD; signal jumps) to overcome the loss of efficiency of vocal cues, adjusting from a multimodal acoustic and visual communication to a mainly visual display (Partan Citation2017). To explore the role of short-term behavioural plasticity in accounting for the patterns observed, we also tested whether a relative change in courtship strategy (visual displays vs vocal cues) would occur after a few hours of increased ambient noise level in populations not normally exposed to high levels of boat/ship noise. This observational study performed on wild individuals in their natural habitat provides initial evidence on the evolutionary adaptation and behavioural flexibility of fish communication in different areas. A more robust analysis should be performed with acoustic recordings of the vocalizations and hydrophones able to record sound pressure levels combined with particle velocity levels as well as sound exposure levels (Spiga et al. Citation2012). We thus highlight the challenges for pure behavioural ecologists and evolutionary biologists to master fast-evolving technologies and suggest a stronger collaboration from the planning stage of projects with acousticians for a more effective characterization of the soundscape and recording of animal vocalizations (see also Popper & Hawkins Citation2019).
Materials and methods
Study areas
Behavioural observations were performed along the Sicilian coast, Italy, in summer 2011 and 2012. Three areas characterised by different maritime traffic (used as proxy of possible different ambient noise levels) were identified as study sites (). The first area (A) was located just outside the main commercial harbour of Palermo, the fifth largest city in Italy. The intense maritime traffic was due to commercial ships, cruise ships, as well as smaller boats used for various purposes around the dock areas (see http://www.marinetraffic.com/it/ais/details/ports/635/Italy_port:PALERMO). Two smaller docks were also present in proximity of the main harbour, one hosting several sailing and motor boats (also affecting ambient noise levels; Haviland-Howell et al. Citation2007) and the other used as a shelter for small fishing boats. The second area, Zingaro (B) was located between two small harbours (the distance between the sampling area and each of the two harbours was approximately 10 km) mostly accessed by recreational boats. The third area (C) was located in a zone where boat traffic is prohibited (Vendicari Nature Reserve). Even though long-term records were not registered, due to logistics, the traffic data should represent the average situation for each area (but we are aware of changes on short term – e.g., weekends vs week days, days vs night). “Marine roads” (i.e., shipping routes; Pirotta et al. Citation2019) and maritime traffic can be used as a “quick and dirty” metric to identify more acoustically impacted areas. Having classified the three areas on the basis of traffic data (), background/ambient noise was measured during each behavioural recording session (see details below) using an uncalibrated hydrophone (H2a-XLR, operational frequency range 10 Hz −100 KHz, sensitivity −180dB re: 1V/µPa, Aquarian Audio Products, Washington, USA) suspended from a boat (3 m deep), connected to a digital recorder (DR-100mkII, recording at 48 KHz 24-bit, Tascam Teac Professional, USA). Gain settings at all locations were kept constant. The methodology used solely relies on sound pressure levels, without including analyses of particle motion and velocity component (Radford et al. Citation2012). Also, the methodology used cannot discriminate the overall background noise in more specific components, such as biological noise (produced by organisms), influences of currents and sediment type, and traffic noise (Robinson et al. Citation2014; Nedelec et al. Citation2015). Nevertheless, the measurements obtained, combined with traffic data, can be used as a proxy of in-situ noise.
Figure 1. Global shipping density map of Sicily, Italy as recorded by Maritime Traffic (https://www.marinetraffic.com/). Traffic density is colour coded, moving from low to high traffic (from blue to red). The sampling sites are identified in the map. Imagery©2015 TerraMetrics; Map data ©2015 Google.
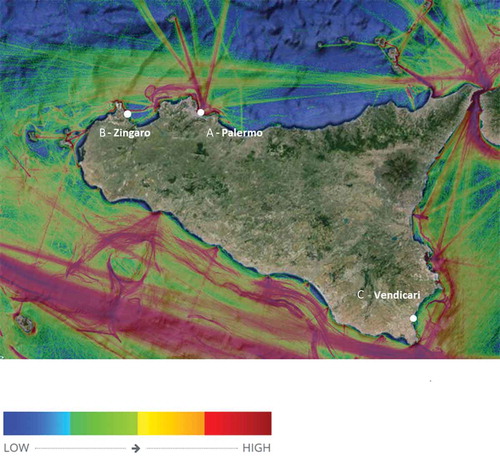
Experimental set-up
Two types of experiments (one observational and one manipulative) were performed to assess the difference in courtship strategies performed by nesting males in different areas. In the first phase, data were collected in the three selected sites (A, B, C). Subsequently, a manipulative field experiment was conducted to assess whether nesting males from the natural reserve of Vendicari (C) were able to adjust their courtship strategy when the ambient noise level was artificially increased with playback of boat noise (D).
Behavioural observations - The three study sites (5–12 m from the coast) were reached by boat and the nesting areas (7–12 m deep) were identified by scuba diving. Video cameras (XTC-200, Midland, China) were installed in front of nests, at a distance of 80–120 cm. Cameras recorded the interactions occurring around the nests between 9:00 am and 11:30 am, in sunny conditions. During that time the hydrophone was activated, to match visual behaviours with background noise. Courtship behaviours were monitored in this way for a total of 47 focal males (20 in A, 15 in B and 12 in C).
Manipulative observation - In order to alter the background noise in the natural reserve (C), a sound track was prepared recording the noise generated by several boats and ships transiting in the commercial harbour of Palermo with the same hydrophone and digital recorder used throughout the behavioural observations. These recordings were pooled to create a 5-min noise track using the software Audacity 2.0.0 (http://audacity.sourceforge.net/). This track was reproduced underwater in Vendicari Nature Reserve using a sound generating system composed by an underwater speaker (LL916C, Lubell Labs, Ohio, USA), an amplifier (HPB-210, Monacor, UK) and a music player, all powered by two 12 Volts 12000 mAh batteries. The underwater speaker was hung 3 m deep from the boat and the 5-min noise track was played continuously for 1 h to let the damselfish colony acclimate to the new disturbance. After this period, behavioural videos and ambient noise were recorded as described in the previous section, while the noise-generating system was playing. The courtship behaviour of the focal males from the observational study was used as the control to this experiment. Thus, videos were monitored for 19 focal males (12 in C and 7 in D).
A total of 54 videos (approximately 81 h of observation in total) were recorded among the three study sites (A, B, C) and the manipulative treatment (D, artificial noise given by playback of boat noise).
Behavioural analyses
An ethogram was prepared using the detailed description of C. chromis behaviour by Verginella et al. (Citation1999), grouping all described behaviours in three main categories: courtship related visual displays (VD; ); mating (MT: time spent by a pair, male and female, in the nest while spawning); non courtship related behaviours (NC: all other behaviours, ranging from cleaning the nest to feeding). The time spent by the focal male outside of the camera view (OV) was also taken into account. From the original recording session (approximately 90 min long) the initial and final parts were removed to minimise the possible disturbance created by the diver accessing the cameras and the scoring was focused on the central 75 min of the recordings. Each video was scored in full length to determine the number of VD performed and the amount of time spent in MT, NC and OV. Then, each focal male was assigned to either the “parental care” (number of VD and MT equal to zero) or “courtship” (number of VD and/or MT greater than zero) groups. The latter group was the focus of the analyses. Because of the slightly asynchronous reproductive cycles among individuals within the same colony, the majority of the males were in parental care, so the available data on courtship behaviours was reduced. Videos were scored using the software JWatcher 1.0 (Blumstein & Daniel Citation2007). For standardisation purposes, the number of visual displays per hour (VD * h−1) was quantified considering only the time spent by focal males in sight of the camera view (i.e., VD * (total time in min – out of site in min)−1 * 60).
Damselfish abundance analyses
Damselfish abundance (number of individuals within the colony) varied across populations. Chromis chromis are well known to be very abundant in the Mediterranean Sea (Pinnegar Citation2018). The variability in adult density across study sites might affect the reproductive behaviour of the fish (Soucy & Travis Citation2003). At the end of randomly chosen sampling sessions, video recordings (n = 6 for A, n = 3 for B, n = 2 for C) were used to estimate relative fish abundance. The diver, while floating at the centre of sampling area, collected a 360° panoramic video-recording of approximately 30 s (Colin et al. Citation2003). Three random snapshots from each recording were used to count the number of damselfish using the software ImageJ 1.46r (Schneider et al. Citation2012). The three abundance values from each observation were averaged and this value was associated to the behavioural observations obtained during the same sampling session. For the behavioural observations during which abundance values were not collected we used the average value of the corresponding study area.
Ambient noise analyses
Ambient noise levels were estimated for each of the three areas (A, B, C) and for the manipulative treatment (D). For each one, ten 1-min subsample tracks were randomly selected from the recordings collected in the field and equivalent continuous sound level (Leq) were estimated for the one-third octave band centre frequencies (1/3 OBCF) 100, 125, 160, 200, 250, 315, 400, 500 and 630 Hz, the range at which the Mediterranean damselfish hear and vocalise (Wysocki et al. Citation2009; Picciulin et al. Citation2010). Subsample tracks were generated with Audacity, and Leq values were estimated using MATLAB (Brandt et al. Citation2011). Variability in ambient noise level between A, B and C and between C and D was compared by examining mean Leq for each narrowband and 95% C.I. All sound tracks were also visualised using the R packages seewave (Sueur et al. Citation2008) and tuneR, following Villanueva-Rivera et al. (Citation2011).
Statistical analyses
Average Leq and 95% confidence intervals (C.I.) were calculated for each frequency narrowband and for each treatment. The difference in the number of VD * h−1 performed by focal males between A (n = 12), B (n = 6) and C (n = 4) were investigated. Also, visual displays were compared between the manipulative treatment D (n = 6) and C (n = 4). For this purpose, two generalised linear models (GLM) were fitted to the data (software R 2.13; R Core Team Citation2018). Due to data overdispersion, a GLM with a negative-binomial distribution and log link function was employed (O’Hara & Kotze Citation2010). The rate of visual displays (VD * h−1) was the response variable and area/treatment the independent variable. Due to marked differences in terms of number of individuals in each colony, relative abundance estimates were included as a covariate in the GLM and visualised and interpreted using the effect display (“effects” package in R; Fox & Hong Citation2009).
Due to the use of three areas, we do not have enough independent replicates to statistically infer differences due to nautical traffic (Hurlbert Citation1984; Jordan Citation2018; Lazic et al. Citation2018). Nevertheless, we offer a biological interpretation (Davies & Gray Citation2015) for behavioural differences among sites, discussing the various factors potentially involved (including boat traffic and corresponding noise).
Results
Ambient noise
The differences in noise level among the four areas/treatments were empirically quantified (). As expected, due to the prohibition of boat traffic, the Vendicari Nature Reserve (C) was the area with lower noise levels, while Zingaro (B), surprisingly, exhibited overlap with the noisy harbour of Palermo (A) across most frequency intervals, and even proved to be “louder” at 400–630 Hz (). In the manipulative treatment (D), the noise-generating system was successful in increasing the ambient noise to levels similar to the ones recorded in A (; ).
Table I. Relative dB uncalibrated ambient noise level (mean, with 95% C.I. in brackets) for each 1/3 OBCF tested for the three study sites and the manipulative treatment. dB values were normalised by setting ‘0’ as the highest value reported.
Figure 3. Examples of selected spectrograms and oscillograms of the three study areas caracherised by different level of noise: a) Palermo; b) Zingaro; c) Vendicari; and d) the artificially altered area Vendicari. These are representative (most common visual representation) of the sound recorded in the different areas.
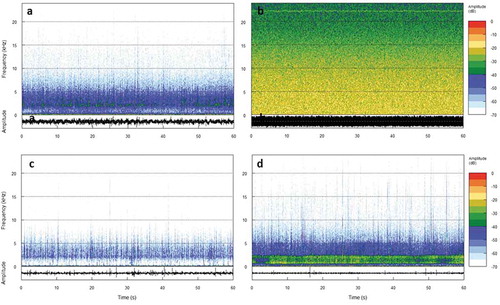
Behavioural responses
During the field observations, the abundance of individuals per colony varied across the three areas (–)). A negative relationship was found between conspecific abundance and the rate of visual displays (z = −2.204, P = 0.0275; )), thus abundance needed to be incorporated in the analyses. Nesting males from the three study areas (A, B and C) showed different numbers of VD per hour (); mean and 95% C.I., back transformed from logarithmic to linear scale: A = 27.9 [15.2, 51.1], B = 13.3 [4.7, 37.4], C = 5.3 [1.6, 17.1]). Specifically, a significant difference was found when comparing A and C: males in the area near the main commercial harbour of Palermo performed significantly more VD than males in the natural reserve (z = 2.786, P = 0.0053). No significant difference was found between B and the other two areas (B vs C, z = 1.121, P = 0.2622; B vs A, z = 1.227, P = 0.2197). After being subjected to an artificially increased ambient noise level (manipulative treatment with playback of boat noise, D), nesting males from C did not change their VD (z = −0.215, P = 0.829; )). Note that in this case damselfish abundance was not used as a covariate, as the two treatments (C and D) occurred in the same study area (Vendicari).
Figure 4. (a-c) Examples of snapshots of the number of individuals in the colonies in the three study locations: a) Palermo; b) Zingaro; c) Vendicari. (d) Effect of number of individuals in the colony on the number of visual displays (mean and 95% C.I.) performed by focal nesting males (plotted with the effect display in R).
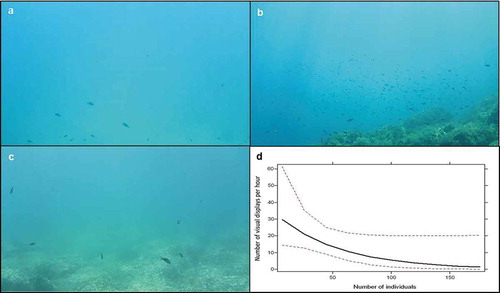
Figure 5. (a) Number of VD * h-1 (back-transformed to linear scale and adjusted for abundance of individuals within the colony) performed by focal nesting males (mean and 95% C.I.) in the three areas, Palermo (A); Zingaro (B); Vendicari (C). (b) Number of VD * h-1 (back-transformed to linear scale) performed by focal nesting males for the two treatments (C and D) in Vendicari (mean and 95% C.I.).
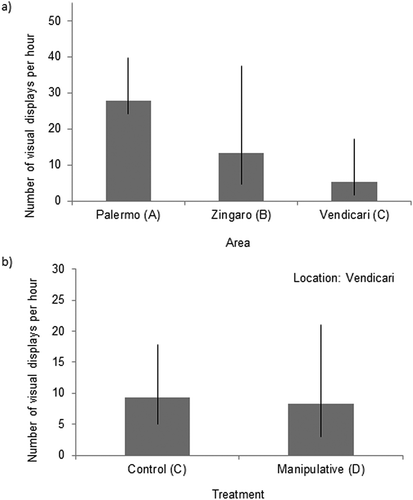
Discussion
Measuring underwater sounds: lesson learned
This observational study was performed to investigate variations in courtship strategies used by male damselfish to attract females to their nests, in different areas, in the field. Acoustic recordings for fish vocalization in open water are methodologically challenging (Benoit-Bird & Lawson Citation2016; Slabbekoorn Citation2016) and often ecologists do not have easy access to the correct instruments or the required expertise from acousticians, from the early planning stages of the experiment. As the techniques advance and allow more relevant underwater measurements (e.g., from sound pressure to particle motion and sound exposure levels; Radford et al. Citation2012; Spiga et al. Citation2012; Hawkins & Popper Citation2018; Popper & Hawkins Citation2019) not only can we use eco-acoustic (Sueur & Farina Citation2015) to monitor biodiversity (Pieretti et al. Citation2017; Akamatsu et al. Citation2018; Doray et al. Citation2018; Linke et al. Citation2018; Gibb et al. Citation2019) and the effects of anthropogenic influences on its distribution, but also we can perform evolutionary and behavioural ecological studies on the effect of noise pollution (Benoit-Bird & Lawson Citation2016) and move from the individual to the population and ecosystem level (Kunc et al. Citation2016; Nabe-Nielsen et al. Citation2018; Lowerre-Barbieri et al. Citation2019). In this study, a lack of calibration of the hydrophone (as sometime still reported in literature; Merchant et al. Citation2015) and the difficulty to record fish vocalizations, resulted in proxy values for ambient noise and the impossibility to analyse male calls. A more multidisciplinary framework to connect ecologists with acousticians should be encouraged to produce stronger analyses.
A comparison of sites characterised by differing levels of boat traffic
We can hypothesize that, when vocalisations fail to attract females to the nest (because of the masking effect of anthropogenic noise), males possibly rely more on visual displays, which are not affected by ambient noise (Neenan et al. Citation2016). Such a multimodal shift from acoustic to visual signalling has been described in a variety of other animals, including frogs, birds and mammals (Caldart et al. Citation2016; Partan Citation2017) and reported in fish (under lab conditions; de Jong et al. Citation2018). In this observational study, a statistically significant difference between two of the three areas under investigation was recorded. Male damselfish from the Palermo commercial harbour (A) performed more courtship-related visual displays (over five times) than individuals from a natural reserve (C). One measured difference between these two sites is ambient noise (which reflects boat traffic). Interestingly, the other area (B), where maritime traffic is considerably less prevalent and less regular than in Palermo harbour, presented a different overall soundscape. By listening to the recordings collected in B, and by looking at the spectrograms of the sound files (), it is reasonable to assume that this result was due to a high baseline noise of non-anthropogenic origin (such as the biophony produced by snapping shrimps, family Alpheidae; Radford et al. Citation2010; Nedelec et al. Citation2015; Pieretti et al. Citation2017; Putland et al. Citation2017; audio files in supplementary material). Snapping shrimp produce a very broad frequency spectrum, with a low frequency start and mean peak levels between 2 and 5 kHz but up to 200 kHz and beyond (Au & Banks Citation1998). They are colonial and defend territories (e.g., Ferguson & Cleary Citation2001), so their sound might be prevalent in some areas but not others. Visual courtship behaviours, in B, were intermediate on average but not statistically different from either A or C. Significant differences might not have been detected due to the great inter-individual variability, typical of animal behaviour (Dall et al. Citation2012; Siegenthaler et al. Citation2018): when comparing two situations extremely different from one another (at least in terms of noise), as A and C, despite individual variability we can still detect significant differences; this is not the case for intermediate situations, as B. Biological components (e.g., the sound produced by snapping shrimp) should interfere less than anthropogenic sounds with acoustic signalling, since during evolutionary time different taxa have utilised different “acoustic windows” (at different frequencies), to avoid interspecific acoustic overlapping (Brumm & Slabbekoorn Citation2005; Lugli Citation2010; Radford et al. Citation2014; Ruppé et al. Citation2015).
Despite the most evident difference among the three sites being maritime traffic, other biological and environmental factors might have played an important role in the trade-off between a more visual or vocal strategy. An increase in visual displays was the hypothesised response to the masking of vocal cues, but a visual strategy can also be risky if detected by predators. Presence and abundance of predators were not assessed in this study; nevertheless the high noise area is likely to be the one with the lowest presence of natural predators of C. chromis while the low noise area, being a natural reserve, is likely to be also the area with highest biodiversity and higher presence of natural predators (Madin et al. Citation2015). The observed reduction of visual displays in the latter might not only depend on the low noise level but also on predation pressure (birds and piscivorous fishes; Pinnegar Citation2018). Moreover, while the males are performing their visual displays (signal jumps), nest predators (i.e., egg eating predators such as the ornate wrasse Thalassoma pavo; Milazzo et al. Citation2006) might take advantage of the absence of the male from the nest to attack the eggs. Thus, visually conspicuous displays might pose a higher risk for nesting males employing them, making predation pressure another likely explanation for the difference in courtship strategy observed between areas characterised by different levels of maritime traffic.
The high number of individuals in one colony seemed to play a role in the investment in visual displays. High abundance of conspecific means high presence of females (thus possibly reduced effort to attract them to the nest) as well as higher presence of sneakers (Picciulin et al. Citation2004; Mascolino et al. Citation2016), conspecific males who can “sneak” in another male’s nest to release sperm while the female is spawning, parasitically fertilising eggs at the expenses of the nesting male. The visual display is a signal that both females (the intended target) and sneakers (the opportunistic receiver) can detect. Again, it is important to underline that noise is not the only driver able to modulate courtship behaviours. In this study, damselfish abundance was included in the analyses, but predation was not, and these two factors seem to play a combined role in other fish species, where in the presence of predators, sneakers were chased less than in the absence of predators (Järvi-Laturi et al. Citation2011).
Visual displays might not be effective in turbid water. Even though turbidity was not measured, the most turbid area of the study was the noisier, Palermo (Mascolino personal observation; see also ), where more visual displays were recorded. Studies on visual communication in fishes have focused on colouration and colour perception and indeed turbidity can mask this important signal to attract females (Seehausen et al. Citation1997). Nevertheless, colour is not important in C. chromis mate choice, and at the low distances at which C. chromis interact, movement can still be easily detected.
Given the limitations associated with the methodology adopted to collect data, the fact that acoustic vocalisations produced by male C. chromis were not recorded and the potential interaction of multiple factors, our results might not be conclusive. Still, on the basis of our evidence it is reasonable to infer that boat traffic, with the concomitant anthropogenic noise, is one of the drivers of the differences seen in the three areas.
Short-term plasticity in response to increased noise levels
When faced with artificially increased ambient noise (D), focal nesting males accustomed to a low-noise environment were not able to adjust their courtship strategy to the new noisier condition after one-hour acclimation and they did not increase their number of visual displays. This result suggests the absence of short-term plasticity. Behavioural plasticity can facilitate the adjustment to anthropogenic pressures or environmental changes. For instance, male guppies are able to quickly adjust their mating behaviour from visual courtship displays in higher light conditions to a sneaky mating strategy under low light and visibility conditions (Chapman et al. Citation2009). In our case a lack of short-term immediate behavioural flexibility might result in more durable adaptive changes in the long term (Swaddle et al. Citation2015; Harding et al. Citation2018). Also, a recent study on Pomacentrus amboinensis juveniles (Holmes et al. Citation2017) showed a short response of fish to small boat noise (20 min only) before fish resume normal behaviours: the used one-hour acclimation time might have allowed a desensitization of the fish by the beginning of the recordings (but this would not be the case if the vocalization would be masked and males had to rely more on visual displays). Collectively, our findings suggest that courtship behaviour in C. chromis may be a locally adapted complex trait, shaped by long-term (multi-generational) exposure to ambient noise levels. The visual vs vocalisation trade-off may represent an important biocomplexity that allows individuals to attain optimal reproductive success in divergent noise environments.
In many species, differential fitness of “courtship genotypes” may be an important factor to be considered for population viability and management, similar to life history traits, such as growth and maturation, which are more commonly considered in a fishery perspective (van Wijk et al. Citation2013). Molecular studies can offer the framework to address this aspect more in detail. In this regards, C. chromis is typically characterised by localised habitat use and marked territoriality as well as a relatively short pelagic larval stage (Raventós & Macpherson Citation2001). Domingues et al. (Citation2005) detected a certain extent of genetic structure among C. chromis population across the Mediterranean Sea, but small-scale empirical evidence for substructure and demographic independence in this species is missing. The two populations from Palermo and Zingaro are characterised by reduced genetic connectivity: a high degree of population structure was recorded (FST = 0.024, 95% BootStrap C.I. 0.02, 0.03), despite being separated by only 70 Km of coastline. If ambient noise proves to select for certain traits over others (i.e., visual display vs vocalisation), it is reasonable to infer that anthropogenic noise-induced evolutionary change will be particularly rapid in sedentary species with low dispersal.
Male C. chromis use both visual and acoustic displays to communicate with conspecifics and attract mates (Picciulin et al. Citation2010). In particular, female reproductive responses are triggered by both visual and auditory stimuli and this multimodal communication is quite common in fishes (Brumm & Slabbekoorn Citation2005; Bolgan et al. Citation2013; Pedroso et al. Citation2013). The fact that there might be the possibility to modulate the signalling modality (Radford et al. Citation2014 and references therein) has been considered as a potential mitigation of the disturbance produced by anthropogenic noise, thus minimizing fitness consequences (Brumm Citation2014). On the other hand, the two modes of communication could be complementary more than redundant, as seen in gobies (Torricelli et al. Citation1986) and the effects of anthropogenic stressors could affect more behaviours at once (McLaughlin & Kunc Citation2015). Indeed, the dynamics of multiple signalling are complex (Bro-Jørgensen Citation2010) and it makes sense that they would evolve in heterogeneous environments, such as the soundscape of coastal areas (Nedelec et al. Citation2015).
Furthermore, anthropogenic noise could have an effect, not only on adult damselfish, but also on juveniles: it has been shown that juveniles of other pomacentrid species are able to use acoustic cues to discriminate among habitats and to be guided during the homing process (Radford et al. Citation2011). This mechanism seems to be plastic (Simpson et al. Citation2010) and can be affected by boat noise (Holles et al. Citation2013). Considering that all fish studied to date are able to hear and that maritime traffic is a frequent, widespread phenomenon at a global scale, it can be expected that every-day human activities could have a pervasive impact on ecological and evolutionary processes of coastal fish species.
Conclusions
There is an increased awareness of the detrimental impact of anthropogenic noise on biodiversity, at multiple levels (Francis & Barber Citation2013; Shannon et al. Citation2016). Recent studies have targeted fitness effects (McGregor et al. Citation2013), especially in birds (e.g., Halfwerk et al. Citation2011) and marine mammals (e.g., New et al. Citation2014). This is one of few field studies observing different behaviours of fish in different areas characterised by different boat traffic and thus noise levels. The overall preliminary findings of this study suggest that investment in visual versus acoustic displays can be affected by ambient noise levels. The association between courtship behaviour and anthropogenic noise does not appear to have short-term plasticity and may result from local adaptation (Swaddle et al. Citation2015); hence ambient noise may represent a key factor in conservation biology and environmental management, not only in relation to short-term disruption, but also as a potential driver of evolutionary change.
Our results suggest that underwater noise pollution could significantly impact the evolutionary trajectories of marine organisms’ communication systems at local scales. More specific studies should be designed to assess this more in detail (Underwood Citation2000): our analysis has pointed out a variety of other possible factors (including predator pressure, population density, water turbidity, courtship genotypes) that can affect changes in courtship displays from acoustic to visual, in the field.
There is still relatively little understanding of the ecological responses of marine organisms to the increase in ambient sound (Slabbekoorn et al. Citation2010; Kunc et al. Citation2016) and this preliminary investigation paves the way towards filling this gap together with other studies on the same species (Codarin et al. Citation2009; Picciulin et al. Citation2010; Bracciali et al. Citation2012). However, the potential long-term effects of these processes warrant an increased awareness in the context of environmental impact assessment and resource management. Special concern should be given to marine protected areas where noise mitigation strategies, such as navigation-speed restrictions or rerouting of shipping lanes (as suggested by Merchant et al. Citation2012), and specific guidelines (Popper & Hawkins Citation2019) are overall necessary to ensure the protection of ecological/evolutionary processes.
Vendicari__D_.wav
Download WAV Audio (11 MB)Vendicari__C_.wav
Download WAV Audio (11 MB)Zingaro__B_.wav
Download WAV Audio (11 MB)Palermo__A_.wav
Download WAV Audio (11 MB)Acknowledgements
We would like to thank: Devin Lyons and Julius Piercy for their assistance with data analysis; Mark Avis and Andy Moorhouse for their advice on acoustics; Donal Lennon for the guidance provided to build the underwater sound-generating system; Natalia Niceta, Jennifer Coughlan, and Siobhán Bradley, Kelly Hickey, Emma Lawlor, Meabh Nic Mhathúna, Claire Morton and Martina O’Brien for their help in the field. Access to the Vendicari no-navigation zone was kindly granted by the crew of R.N.O. Oasi Faunistica di Vendicari. The research reported here follows the Association for the Study of Animal Behaviour and Animal Behavior Society Guidelines for the Use of Animals in Research and does not include any collection of samples (just video recordings). Ethical approval was obtained by UCD, University College Dublin (AREC-P-10-33).
Disclosure statement
No potential conflict of interest was reported by the authors.
Supplementary material
Supplemental data for this article can be accessed here.
Additional information
Funding
References
- Aguilar de Soto N. 2016. Peer-reviewed studies on the effects of anthropogenic noise on marine invertebrates: From scallop larvae to giant squid. In: Popper AN, Hawkins A, editors. The effects of noise on aquatic life II. New York: Springer. pp. 17–26. DOI: 10.1007/978-1-4939-2981-8_3.
- Aguilar de Soto N, Gkikopoulou K, Hooker S, Isojunno S, Johnson M, Miller P, Tyack P, Wensveen P, Donovan C, Harris CM, Harris D, Marshall L, Oedekoven C, Prieto R, Thomas L. 2016. From physiology to policy: A review of physiological noise effects on marine fauna with implications for mitigation. Proceedings of Meetings on Acoustics 27:040008. DOI: 10.1121/2.0000299.
- Akamatsu T, Lin TH, Tsao Y, Sinniger F, Harii S. 2018. Coral reef soundscape to measure the species distribution and biodiversity. 2018. Kobe: OCEANS-MTS/IEEE Kobe Techno-Oceans (OTO). pp. 1–4. DOI: 10.1109/OCEANSKOBE.2018.8559475
- Allen GR. 1991. Damselfish of the World. Melle, Germany: Mergus Press.
- Amorim MCP. 2006. Diversity of sound production in fish. In: Ladich F, Collin SP, Moller P, Kapoor BG, editors. Communication in fishes. Enfield: Science Publishers. pp. 71–104.
- Amorim MCP, Neves A. 2007. Acoustic signalling during courtship in the painted goby, Pomatoschistus pictus. Journal of Marine Biological Association United Kingdom 87:1017–1023. DOI: 10.1017/S0025315407056822.
- Amorim MCP, Pedroso SS, Bolgan M, Jordao JM, Caiano M, Fonseca PJ. 2012. Painted gobies sing their quality out loud: Acoustic rather than visual signals advertise male quality and contribute to mating success. Functional Ecology 27:289–298. DOI: 10.1111/1365-2435.12032.
- André M, Kaifu K, Solé M, van der Schaar M, Akamatsu T, Balastegui A, Sánchez AM, Castell JV. 2016. Contribution to the understanding of particle motion perception in marine invertebrates. In: Popper AN, Hawkins A, editors. The effects of noise on aquatic life II. New York: Springer. pp. 47–55. DOI: 10.1007/978-1-4939-2981-8_6.
- Au WW, Banks K. 1998. The acoustics of the snapping shrimp Synalpheus parneomeris in Kaneohe Bay. Journal of the Acoustical Society of America 103:41–47. DOI: 10.1121/1.423234.
- Barber JR, Crooks KR, Fristrup KM. 2009. The costs of chronic noise exposure for terrestrial organisms. Trends in Ecology & Evolution 25:180–189. DOI: 10.1016/j.tree.2009.08.002.
- Bass AH, Ladich F. 2008. Vocal-acoustic communication: From neurons to behavior. In: Webb JF, Fay RR, Popper AN, editors. Fish bioacoustics. Springer Handbook of Auditory Research 32. New York, NY: Springer. pp. 253–278.
- Benoit-Bird KJ, Lawson GL. 2016. Ecological insights from pelagic habitats acquired using active acoustic techniques. Annual Review Marine Science 8:463–490. DOI: 10.1146/annurev-marine-122414-034001.
- Bessey C, Heithaus MR. 2013. Alarm call production and temporal variation in predator encounter rates for a facultative teleost grazer in a relatively pristine seagrass ecosystem. Journal of Experimental and Marine Biology and Ecology 449:135–141. DOI: 10.1016/j.jembe.2013.09.008.
- Blumstein DT, Daniel JC. 2007. Quantifying behavior the JWatcher Way. Massachusetts, USA: Sinauer Associates. DOI: 10.1093/icb/icn005.
- Bolgan M, Chorazyczewska E, Winfield IJ, Codarin A, O’Brien J, Gammell M. 2016. First observations of anthropogenic underwater noise in a large multi-use lake. Journal of Limnology 75:644–651.
- Bolgan M, Pedroso SS, Picciulin M, Fonseca PJ, Amorim MCP. 2013. Differential investment in acoustic communication during social interactions in two closely-related sand goby species. Behaviour 150:133–152. DOI: 10.1163/1568539X-00003041.
- Bracciali C, Campobello D, Giacoma C, Sarà G. 2012. Effects of nautical traffic and noise on foraging patterns of Mediterranean damselfish (Chromis chromis). PLoS ONE 7:e40582. DOI: 10.1371/journal.pone.0040582.
- Brandt MJ, Diederichs A, Betke K, Nehls G. 2011. Responses of harbour porpoises to pile driving at the Horns Rev II offshore wind farm in the Danish North Sea. Marine Ecology Progress Series 421:205–216. DOI: 10.3354/meps08888.
- Bro-Jørgensen J. 2010. Dynamics of multiple signalling systems: Animal communication in a world in flux. Trends in Ecology & Evolution 25:292–300. DOI: 10.1016/j.tree.2009.11.003.
- Brumm H. 2014. Fish struggle to be heard-but just how much fin waving is there? A comment on Radford et al. Behavioral Ecology 25:1033–1034. DOI: 10.1093/beheco/aru029.
- Brumm H, Slabbekoorn H. 2005. Acoustic communication in noise. Advances in the Study of Behavior 35:151–209. DOI: 10.1016/S0065-3454(05)35004-2.
- Caldart VM, Iop S, Lingnau R, Cechin SZ. 2016. Communication in a noisy environment: Short-term acoustic adjustments and the underlying acoustic niche of a Neotropical stream-breeding frog. Acta Ethologica 19:151–162. DOI: 10.1007/s10211-016-0235-2.
- Casaretto L, Picciulin M, Hawkins AD. 2015. Seasonal patterns and individual differences in the calls of male haddock Melanogrammus aeglefinus. Journal of Fish Biology 87:579–603. DOI: 10.1111/jfb.12740.
- Chapman BB, Morrell LJ, Krause J. 2009. Plasticity in male courtship behaviour as a function of light intensity in guppies. Behavioral Ecology and Sociobiology 63:1757–1763. DOI: 10.1007/s00265-009-0796-4.
- Codarin A, Wysocki LE, Ladich F, Picciulin M. 2009. Effects of ambient and boat noise on hearing and communication in three fish species living in a marine protected area (Miramare, Italy). Marine Pollution Bulletin 58:1880–1887. DOI: 10.1016/j.marpolbul.2009.07.011.
- Colin PL, Sadovy YJ, Domeier ML. 2003. Manual for the study and conservation of reef fish spawning aggregations. Society for the conservation of reef fish aggregations special publication No. 1 (Version 1.0). pp. 1–98+iii. https://www.researchgate.net/profile/Michael_Domeier/publication/228436345_Manual_for_the_Study_and_Conservation_of_Reef_Fish_Spawning_Aggregations/links/0deec528510e82dc7b000000.pdf.
- Dall SRX, Bell AM, Bolnick DI, Ratnieks FLW. 2012. An evolutionary ecology of individual differences. Ecology Letters 15:1189–1198. DOI: 10.1111/j.1461-0248.2012.01846.x.
- Davies GM, Gray A. 2015. Don’t let spurious accusations of pseudoreplication limit our ability to learn from natural experiments (and other messy kinds of ecological monitoring). Ecology and Evolution 5:5295–5304. DOI: 10.1002/ece3.1782.
- de Jong K, Amorim M, Fonseca PJ, Heubel KU. 2018. Noise affects multimodal communication during courtship in a marine fish. Frontiers in Ecology and Evolution 6:113. DOI: 10.3389/fevo.2018.00113.
- Domingues VS, Bucciarelli G, Almada V, Bernardi G. 2005. Historical colonization and demography of the Mediterranean damselfish, Chromis chromis. Molecular Ecology 14:4051–4063. DOI: 10.1111/j.1365-294X.2005.02723.x.
- Dooling RJ, Leek MR, Popper AN. 2015. Effects of noise on fishes: What we can learn from humans and birds. Integrative Zoology 10:29–37. DOI: 10.1111/1749-4877.12094.
- Doray M, Petitgas P, Romagnan JB, Huret M, Duhamel E, Dupuy C, Spitz J, Authier M, Sanchez F, Berger L, Dorémus G, Bourriau P, Grellier P, Massé J. 2018. The PELGAS survey: Ship-based integrated monitoring of the Bay of Biscay pelagic ecosystem. Progress in Oceanography 166:15–29. DOI: 10.1016/j.pocean.2017.09.015.
- Ellison W, Southall B, Clark C, Frankel A. 2011. A new context-based approach to assess marine mammal behavioral responses to anthropogenic sounds. Conservation Biology 26:21–28. DOI: 10.1111/j.1523-1739.2011.01803.x.
- Fakan E, McCormick M. 2019. Boat noise affects the early life history of two damselfishes. Marine Pollution Bulletin 141:493–500. DOI: 10.1016/j.marpolbul.2019.02.054.
- Ferguson BG, Cleary JL. 2001. In situ source level and source position estimates of biological transient signals produced by snapping shrimp in an underwater environment. The Journal of the Acoustical Society of America 109:3031–3037. DOI: 10.1121/1.1339823.
- Fox J, Hong J. 2009. Effect displays in R for multinomial and proportional-odds logit models: Extensions to the effects package. Journal of Statistical Software 32:1–24. DOI: 10.1111/j.1467-9531.2006.00180.x.
- Francis CD, Barber JR. 2013. A framework for understanding noise impacts on wildlife: An urgent conservation priority. Frontiers in Ecology and the Environment 11:305–314. DOI: 10.1890/120183.
- Gibb R, Browning E, Glover-Kapfer P, Jones KE. 2019. Emerging opportunities and challenges for passive acoustics in ecological assessment and monitoring. Methods in Ecology and Evolution 10:169–185. DOI: 10.1111/2041-210X.13101.
- Gordon TAC, Harding HR, Clever FK, Davidson IK, Davison W, Montgomery DW, Weatherhead RC, Windsor FM, Armstrong JD, Bardonnet A, Bergman E, Britton JR, Côté IM, D’agostino D, Greenberg LA, Harborne AR, Kahilainen KK, Metcalfe NB, Mills SC, Milner NJ, Mittermayer FH, Montorio L, Nedelec SL, Prokkola JM, Rutterford LA, Salvanes AGV, Simpson SD, Vainikka A, Pinnegar JK, Santos EM. 2018. Fishes in a changing world: Learning from the past to promote sustainability of fish populations. Journal of Fish Biology 92:804–827. DOI: 10.1111/jfb.2018.92.issue-3.
- Greggor AL, Berger-Tal O, Blumstein DT, Angeloni L, Bessa-Gomes C, Blackwell BF, St Clair CC, Crooks K, De Silva S, Fernández-Juricic E, Goldenberg SZ, Mesnick SL, Owen M, Price CJ, Saltz D, Schell CJ, Suarez AV, Swaisgood RR, Winchell CS, Sutherland WJ. 2016. Research priorities from animal behaviour for maximising conservation progress. Trends in Ecology & Evolution 31:953–964. DOI: 10.1016/j.tree.2016.09.001.
- Haelters J, Debusschere E, Botteldooren D, Dulière V, Hostens K, Norro A. 2013. The effects of pile driving on marine Mammals and fish in Belgian waters. In: Degraer S, Brabant R, Rumes B, editors. Environmental impacts of offshore wind farms in the Belgian part of the North Sea. pp. 70–77. DOI: 10.1088/1748-9326/9/3/034012.
- Halfwerk W, Holleman LJM, Lessels CM, Slabbekoorn H. 2011. Negative impact of traffic noise on avian reproductive success. Journal of Applied Ecology 48:210–219. DOI: 10.1111/j.1365-2664.2010.01914.x.
- Harding HR, Gordon TAC, Hsuan RE, Mackaness ACE, Radford AN, Simpson SD. 2018. Fish in habitats with higher motorboat disturbance show reduced sensitivity to motorboat noise. Biology Letters 14:20180441. DOI: 10.1098/rsbl.2018.0441.
- Hasan MR, Crane AL, Ferrari MC, Chivers DP. 2018. A cross-modal effect of noise: The disappearance of the alarm reaction of a freshwater fish. Animal Cognition 21:419–424. DOI: 10.1007/s10071-018-1179-x.
- Haviland-Howell G, Frankel AS, Powell CM, Bocconcelli A, Herman RL, Sayigh LS. 2007. Recreational boating traffic: A chronic source of anthropogenic noise in the Wilmington, North Carolina Intracoastal Waterway. Journal of Acoustic Society of America 122:151–160. DOI: 10.1121/1.2717766.
- Hawkins AD, Popper AN. 2018. Effects of man-made sound on fishes. In: Slabbekoorn H, Dooling R, Popper AN, Fay RR, editors. Effects of anthropogenic noise on animals. New York, NY: Springer. pp. 145–177.
- Hildebrand JA. 2009. Anthropogenic and natural sources of ambient noise in the ocean. Marine Ecology Progress Series 395:5–20. DOI: 10.3354/meps08353.
- Holles S, Simpson SD, Radford AN, Berten L, Lecchini D. 2013. Boat noise disrupts orientation behaviour in a coral reef fish. Marine Ecology Progress Series 485:295–300. DOI: 10.3354/meps10346.
- Holmes LJ, McWilliam J, Ferrari MC, McCormick MI. 2017. Juvenile damselfish are affected but desensitize to small motor boat noise. Journal of Experimental Marine Biology and Ecology 494:63–68. DOI: 10.1016/j.jembe.2017.05.009.
- Hurlbert SH. 1984. Pseudoreplication and the design of ecological field experiments. Ecological Monographs 54:187–211. DOI: 10.2307/1942661.
- Järvi-Laturi M, Lindstrӧm K, Kvarnemo C, Svensson O. 2011. Sand goby males trade off between defence against egg predators and sneak intrusions. Journal of Zoology 283:269–275. DOI: 10.1111/j.1469-7998.2011.00788.x.
- Jordan CY. 2018. Population sampling affects pseudoreplication. PLoS Biology 16:e2007054. DOI: 10.1371/journal.pbio.2007054.
- Kasumyan A. 2008. Sounds and sound production in fishes. Journal of Ichthyology 48:981–1030. DOI: 10.1134/S0032945208110039.
- Kunc HP, McLaughlin KE, Schmidt R. 2016. Aquatic noise pollution: Implications for individuals, populations, and ecosystems. Proceedings of the Royal Society B: Biological Sciences 283:20160839. DOI: 10.1098/rspb.2016.0839.
- Ladich F. 2013. Effects of noise on sound detection and acoustic communication in fishes. In: Brumm H, editor. Animal communication and noise. Berlin Heidelberg: Springer-Verlag. pp. 65–90. DOI: 10.1007/978-3-642-41494-7_4.
- Ladich F, Fay RR. 2013. Auditory evoked potential audiometry in fish. Reviews in Fish Biology and Fisheries 23:317–364. DOI: 10.1007/s11160-012-9297-z.
- Laglbauer BJ, Afonso P, Donnay A, Santos RS, Fontes J. 2017. Reproductive synchrony in a temperate damselfish, Chromis limbata. Acta Ethologica 20:297–311. DOI: 10.1007/s10211-017-0269-0.
- Lazic SE, Clarke-Williams CJ, Munafò MR. 2018. What exactly is ‘N’ in cell culture and animal experiments? PLoS Biology 16:e2005282. DOI: 10.1371/journal.pbio.2005282.
- Linke S, Gifford T, Desjonquères C, Tonolla D, Aubin T, Barclay L, Karaconstantis C, Kennard MJ, Rybak F, Sueur J. 2018. Freshwater ecoacoustics as a tool for continuous ecosystem monitoring. Frontiers in Ecology and the Environment 16:231–238. DOI: 10.1002/fee.2018.16.issue-4.
- Lobel PS, Kaatz IM, Rice AN. 2010. Acoustical behaviour of coral reef fishes. In: Cole KS, editor. Reproduction and sexuality in marine fishes. Patterns and processes. Berkeley, CA, USA: University of California Press. pp. 307–385. DOI: 10.1525/california/9780520264335.001.0001.
- Lowerre-Barbieri SK, Catalán IA, Frugård Opdal A, Jørgensen C. 2019. Preparing for the future: Integrating spatial ecology into ecosystem-based management. ICES Journal of Marine Science 76:467–476. DOI: 10.1093/icesjms/fsy209.
- Lugli M. 2010. Sounds of shallow water fishes pitch within the quiet window of the habitat ambient noise. Journal of Comparative Physiology 196:439–451. DOI: 10.1007/s00359-010-0528-2.
- Madin EM, Dill LM, Ridlon AD, Heithaus MR, Warner RR. 2015. Human activities change marine ecosystems by altering predation risk. Global Change Biology 22:44–60. DOI: 10.1111/gcb.13083.
- Mann DA, Lobel PS. 1997. Propagation of damselfish (Pomacentridae) courtship sounds. The Journal of the Acoustical Society of America 101:3783–3791. DOI: 10.1121/1.418425.
- Maruska KP, Ung US, Fernald RD. 2012. The African cichlid fish Astatotilapia burtoni uses acoustic communication for reproduction: Sound production, hearing, and behavioral significance. PLoS One 7:e37612. DOI: 10.1371/journal.pone.0037612.
- Mascolino S, Benvenuto C, Gubili C, Sacchi C, Boufana B, Mariani S. 2016. The ART of mating: Alternative reproductive tactics and mating success in a nest-guarding fish. Journal of Fish Biology 89:2643–2657. DOI: 10.1111/jfb.13130.
- McGregor PK, Horn AG, Leonard ML, Thomsen F. 2013. Anthropogenic noise and conservation. In: Brumm H, editor. Animal communication and noise. Berlin Heidelberg: Springer-Verlag. pp. 409–444. DOI: 10.1007/978-3-642-41494-7_1.
- McLaughlin KE, Kunc HP. 2015. Changes in the acoustic environment alter the foraging and sheltering behaviour of the cichlid Amititlania nigrofasciata. Behavioural Processes 116:75–79. DOI: 10.1016/j.beproc.2015.04.012.
- Merchant ND, Fristrup KM, Johnson MP, Tyack PL, Witt MJ, Blondel P, Parks SE. 2015. Measuring acoustic habitats. Methods in Ecology and Evolution 6:257–265. DOI: 10.1111/2041-210X.12330.
- Merchant ND, Witt MJ, Blondel P, Godley BJ, Smith GH. 2012. Assessing sound exposure from shipping in coastal waters using a single hydrophone and Automatic Identification System (AIS) data. Marine Pollution Bulletin 64:1320–1329. DOI: 10.1016/j.marpolbul.2012.05.004.
- Mickle MF, Higgs DM. 2017. Integrating techniques: A review of the effects of anthropogenic noise on freshwater fish. Canadian Journal of Fisheries and Aquatic Sciences 75:1534–1541. DOI: 10.1139/cjfas-2017-0245.
- Milazzo M, Anastasi I, Willis TJ. 2006. Recreational fish feeding affects coastal fish behavior and increases frequency of predation on damselfish Chromis chromis nests. Marine Ecology Progress Series 310:165–172. DOI: 10.3354/meps310165.
- Miller P, Kvadsheim P, Lam F, Tyack P, Curé C, DeRuiter S, Wensveen PJ, von Benda-beckmann AM, MartínLópez LM, Narazaki T, Hooker SK. 2015. First indications that northern bottlenose whales are sensitive to behavioural disturbance from anthropogenic noise. Royal Society Open Science 2:140484. DOI: 10.1098/rsos.140484.
- Millot S, Vandewalle P, Parmentier E. 2011. Sound production in red-bellied piranhas (Pygocentrus nattereri, Kner): An acoustical, behavioural and morphofunctional study. Journal of Experimental Biology 214:3613–3618. DOI: 10.1242/jeb.061218.
- Myrberg AA. 1997. Sound production by a coral reef fish (Pomacentrus partitus): Evidence for a vocal, territorial “Keep Out” signal. Bulletin Marine Science 60:1017–1025.
- Myrberg AA, Spires JY. 1972. Sound discrimination by the bicolor damselfish, Eupomacentrus partitus. Journal of Experimental Biology 57:727–735.
- Nabe-Nielsen J, van Beest FM, Grimm V, Sibly RM, Teilmann J, Thompson PM. 2018. Predicting the impacts of anthropogenic disturbances on marine populations. Conservation Letters 11:e12563. DOI: 10.1111/conl.12563.
- Nedelec SL, Simpson SD, Holderied M, Radford AN, Lecellier G, Radford C, Lecchini D. 2015. Soundscapes and living communities in coral reefs: Temporal and spatial variation. Marine Ecology Progress Series 524:125–135. DOI: 10.3354/meps11175.
- Neenan ST, Piper R, White PR, Kemp P, Leighton TG, Shaw PJ. 2016. Does masking matter? Shipping noise and fish vocalizations. In: Popper AN, Hawkins A, editors. The effects of noise on aquatic life II. New York: Springer. pp. 747–753.
- New LF, Clark JS, Costa DP, Fleishman E, Hindell MA, Klanjšček T, Lusseau D, Kraus S, McMahon CR, Robinson PW, Schick RS, Schwarz LK, Simmons SE, Thomas L, Tyack P, Harwood J. 2014. Using short-term measures of behaviour to estimate long-term fitness of southern elephant seals. Marine Ecology Progress Series 496:99–108. DOI: 10.3354/meps10547.
- O’Hara RB, Kotze DJ. 2010. Do not log-tranform count data. Methods in Ecology and Evolution 1:118–122. DOI: 10.1111/j.2041-210X.2010.00021.x.
- Parmentier E, Kever L, Casadevall M, Lecchini D. 2010. Diversity and complexity in the acoustic behaviour of Dacyllus flavicaudus (Pomacentridae). Marine Biology 157:2317–2327. DOI: 10.1007/s00227-010-1498-1.
- Partan SR. 2017. Multimodal shifts in noise: Switching channels to communicate through rapid environmental change. Animal Behaviour 124:325–337. DOI: 10.1016/j.anbehav.2016.08.003.
- Pedroso SS, Barber I, Svensson O, Fonseca PJ, Amorim MCP. 2013. Courtship sounds advertise species identity and male quality in sympatric Pomatoschistus spp. gobies. PLoS ONE 8:e64620. DOI: 10.1371/journal.pone.0064620.
- Pereira R, Rismondo S, Caiano M, Pedroso SS, Fonseca PJ, Amorim MCP. 2014. The role of agonistic sounds in male nest defence in the painted goby Pomatoschistus pictus. Ethology 120:53–63. DOI: 10.1111/eth.12180.
- Picciulin M, Costantini M, Hawkins AD, Ferrero EA. 2002. Sound emissions of the Mediterranean damselfish Chromis chromis (Pomacentridae). Bioacoustics 12:236–238. DOI: 10.1080/09524622.2002.9753707.
- Picciulin M, Sebastianutto L, Codarin A, Farina A, Ferrero EA. 2010. In situ behavioural responses to boat noise exposure of Gobius cruentatus (Gmelin, 1789; fam. Gobiidae) and Chromis chromis (Linnaeus, 1758; fam. Pomacentridae) living in a Marine Protected Area. Journal of Experimental Marine Biology and Ecology 386:125–132. DOI: 10.1016/j.jembe.2010.02.012.
- Picciulin M, Verginella L, Spoto M, Ferrero EA. 2004. Colonial nesting and the importance of the brood size in male parasitic reproduction of the Mediterranean damselfish Chromis chromis (Pisces: Pomacentridae). Environmental Biology of Fishes 70:23–30. DOI: 10.1023/B:EBFI.0000022851.49302.df.
- Pieretti N, Martire ML, Farina A, Danovaro R. 2017. Marine soundscape as an additional biodiversity monitoring tool: A case study from the Adriatic Sea (Mediterranean Sea). Ecological Indicators 83:13–20. DOI: 10.1016/j.ecolind.2017.07.011.
- Pinnegar J. 2018. Why the damselfish Chromis chromis is a key species in the Mediterranean rocky littoral-a quantitative perspective. Journal of Fish Biology 92:851–872. DOI: 10.1111/jfb.13551.
- Pirotta V, Grech A, Jonsen ID, Laurance WF, Harcourt RG. 2019. Consequences of global shipping traffic for marine giants. Frontiers in Ecology and Environment 17:39–47. DOI: 10.1002/fee.1987.
- Popper AN. 2003. Effects of anthropogenic sounds on fishes. Fisheries 28:24–31. DOI: 10.1111/j.1095-8649.2009.02319.x.
- Popper AN, Fay RR. 2011. Rethinking sound detection by fishes. Hearing Research 273:25–36. DOI: 10.1016/j.heares.2009.12.023.
- Popper AN, Hastings MC. 2009. The effects of human-generated sound on fish. Integrative Zoology 4:43–52. DOI: 10.1111/j.1749-4877.2008.00134.x.
- Popper AN, Hawkins AD. 2019. An overview of fish bioacoustics and the impacts of anthropogenic sounds on fishes. Journal of Fish Biology 94:692–713. DOI: 10.1111/jfb.13948.
- Popper AN, Smith ME, Cott PA, Hanna BW, MacGillivray AO, Austin ME, Mann DA. 2005. Effects of exposure to seismic airgun use on hearing of three fish species. Journal of the Acoustical Society of America 117:3958–3971. DOI: 10.1121/1.1904386.
- Putland R, Constantine R, Radford C. 2017. Exploring spatial and temporal trends in the soundscape of an ecologically significant embayment. Scientific Reports 7:5713. DOI: 10.1038/s41598-017-06347.
- Putland RL, Merchant ND, Farcas A, Radford CA. 2018. Vessel noise cuts down communication space for vocalizing fish and marine mammals. Global Change Biology 24:1708–1721. DOI: 10.1111/gcb.13996.
- Quignard JP, Pras A. 1986. Pomacentridae. In: Whitehead PJP, Bauchot ML, Hureau JC, Nielsen J, Tottonese E, editors. Fishes of the North-eastern Atlantic and Mediterranean (Volume II, pp. 916–918). UK: UNESCO.
- R Core Team. 2018. R: A language and environment for statistical computing. Vienna, Austria: R Foundation for Statistical Computing. Available: https://www.R-project.org/. Accessed Nov 2019 27.
- Radford AN, Kerridge E, Simpson SD. 2014. Acoustic communication in a noisy world: Can fish compete with anthropogenic noise? Behavioral Ecology 25:1022–1030. DOI: 10.1093/beheco/aru029.
- Radford AN, Purser J, Bruintjes R, Voellmy IK, Everley KA, Wale MA, Holles S, Simpson SD. 2015. Beyond a simple effect: Variable and changing responses to anthropogenic noise. In: Popper AN, Hawkins A, editors. The effects of noise on aquatic life II. New York: Springer. pp. 901–907. DOI: 10.1007/978-1-4939-2981-8_111.
- Radford CA, Montgomery JC, Caiger P, Higgs DM. 2012. Pressure and particle motion detection thresholds in fish: A re-examination of salient auditory cues in teleosts. Journal of Experimental Biology 215:3429–3435. DOI: 10.1242/jeb.073320.
- Radford CA, Stanley JA, Simpson SD, Jeffs AG. 2011. Juvenile coral reef fish use sound to locate habitats. Coral Reefs 30:295–305. DOI: 10.1007/s00338-010-0710-6.
- Radford CA, Stanley JA, Tindle CT, Montgomery JC, Jeffs AG. 2010. Localised coastal habitats have distinct underwater sound signatures. Marine Ecology Progress Series 401:21–29. DOI: 10.3354/meps08451.
- Raventós N, Macpherson E. 2001. Planktonic larval duration and settlement marks on the otoliths of Mediterranean littoral fishes. Marine Biology 138:1115–1120. DOI: 10.1007/s002270000535.
- Robinson SP, Lepper PA, Hazelwood RA. 2014. Good practice guide for underwater noise measurement. National Measurement Office, Marine Scotland, The Crown Estate, NPL Good Practice Guide 133. ISSN: 1368-6550. https://www.npl.co.uk/special-pages/guides/gpg133underwater.
- Ruppé L, Clément G, Herrel A, Ballesta L, Décamps T, Kéver L, Parmentier E. 2015. Environmental constraints drive the partitioning of the soundscape in fishes. Proceedings of the National Academy of Sciences 112:6092–6097. DOI: 10.1073/pnas.1424667112.
- Schneider CA, Rasband WS, Eliceiri KW. 2012. NIH Image to ImageJ: 25 years of image analysis. Nature Methods 9:671–675. DOI: 10.1038/nmeth.2089.
- Seehausen O, Van Alphen JJ, Witte F. 1997. Cichlid fish diversity threatened by eutrophication that curbs sexual selection. Science 277:1808–1811. DOI: 10.1126/science.277.5333.1808.
- Shannon G, McKenna MF, Angeloni LM, Crooks KR, Fristrup KM, Brown E, Warner KA, Nelson MD, White C, Briggs J, Briggs J, McFarland S, Wittemyer G. 2016. A synthesis of two decades of research documenting the effects of noise on wildlife. Biological Reviews 91:982–1005. DOI: 10.1111/brv.12207.
- Siegenthaler A, Mastin A, Dufaut C, Mondal D, Benvenuto C. 2018. Background matching in the brown shrimp Crangon crangon: Adaptive camouflage and behavioural-plasticity. Scientific Reports 8:3292. DOI: 10.1038/s41598-018-21412-y.
- Simpson SD, Meekan MG, Larsen NJ, McCauley RD, Jeffs A. 2010. Behavioral plasticity in larval reef fish: Orientation is influenced by recent acoustic experiences. Behavioral Ecology 21:1098–1105. DOI: 10.1093/beheco/arq117.
- Slabbekoorn H. 2016. Aiming for progress in understanding underwater noise impact on fish: Complementary need for indoor and outdoor studies. In: Popper AN, Hawkins A, editors. The effects of noise on aquatic life II. New York: Springer. pp. 1057–1065.
- Slabbekoorn H, Bouton N, van Opzeeland I, Coers A, Ten Cate C, Popper AN. 2010. A noisy spring: The impact of globally rising underwater sound levels on fish. Trends in Ecology & Evolution 25:419–427. DOI: 10.1016/j.tree.2010.04.005.
- Soucy S, Travis J. 2003. Multiple paternity and population genetic structure in natural populations of the poeciliid fish, Heterandria formosa. Journal of Evolutionary Biology 16:1328–1336. DOI: 10.1046/j.1420-9101.2003.00608.x.
- Southall BL, Bowles AE, Ellison WT, Finneran JJ, Gentry RL, Greene JCR, Kastak D, Ketten DR, Miller JH, Nachtigal PE, Richardson WJ, Thomas JA, Tyack PL. 2008. Marine mammal noise-exposure criteria: Initial scientific recommendations. Bioacoustics 17:273–275. DOI: 10.1578/AM.33.4.2007.411.
- Spiga I, Cheesman S, Hawkins A, Perez-Dominguez R, Roberts L, Hughes D, Elliott M, Nedwell J, Bentley M. 2012. Understanding the scale and impacts of anthropogenic noise upon fish and invertebrates in the marine environment. Defra: SoundWaves Consortium Technical Review (ME5205).
- Sueur J, Aubin T, Simonis C. 2008. Seewave: A free modular tool for sound analysis and synthesis. Bioacoustics 18:213–226. DOI: 10.1080/09524622.2008.9753600.
- Sueur J, Farina A. 2015. Ecoacoustics: The ecological investigation and interpretation of environmental sound. Biosemiotics 8:493–502. DOI: 10.1007/s12304-015-9248-x.
- Swaddle JP, Francis CD, Barber JR, Cooper CB, Kyba CC, Dominoni DM, Shannon G, Aschehoug E, Goodwin SE, Kawahara AY, Luther D, Spoelstra K, Voss M, Longcore T. 2015. A framework to assess evolutionary responses to anthropogenic light and sound. Trends in Ecology & Evolution 30:550–560. DOI: 10.1016/j.tree.2015.06.009.
- Tidau S, Briffa M. 2016. Review on behavioral impacts of aquatic noise on crustaceans. In: Proceedings of Meetings on Acoustics 4ENAL. Volume 27: Fourth International Conference on the Effects of Noise on Aquatic Life, Dublin, Ireland, 10-16 July. https://asa.scitation.org/doi/pdf/10.1121/2.0000302?class=pdf.
- Tolimieri N, Haine O, Jeffs A, McCauley R, Montgomery J. 2004. Directional orientation of pomacentrid larvae to ambient reef sound. Coral Reefs 23:184–191. DOI: 10.1007/s00338-004-0383-0.
- Torricelli P, Lugli M, Gandolfi G. 1986. A quantitative analysis of the occurrence of visual and acoustic displays during the courtship in the freshwater goby, Padogobius martensi (Günther, 1961) (Pisces, Gobiidae). Italian Journal of Zoology 53:85–89. DOI: 10.1080/11250008609355488.
- Underwood A. 2000. Importance of experimental design in detecting and measuring stresses in marine populations. Journal of Aquatic Ecosystem Stress and Recovery 7:3–24. DOI: 10.1023/A:1009983229076.
- van Wijk SJ, Taylor MI, Creer S, Dreyer C, Rodrigues FM, Ramnarine IW, van Oosterhout C, Carvalho GR. 2013. Experimental harvesting of fish populations drives genetically based shifts in body size and maturation. Frontiers in Ecology and Environment 11:181–187. DOI: 10.1890/120229.
- Verginella L, Spoto M, Ciriaco S, Ferrero EA. 1999. Ethogram of the reproductive territorial male of the Mediterranean damselfish Chromis chromis L. (Pisces: Pomacentridae). Bollettino della Società Adriatica di Scienze Naturali LXXVIII:437–454.
- Villanueva-Rivera L, Pijanowski BC, Doucette J, Pekin B. 2011. A primer of acoustic analysis for landscape ecologists. Landscape Ecology 9:1233–1246. DOI: 10.1007/s10980-011-9636-9.
- Wahlberg M, Westerberg H. 2005. Hearing in fish and their reactions to sounds from offshore wind farms. Marine Ecology Progress Series 288:295–309. DOI: 10.3354/meps288295.
- Wale MA, Simpson SD, Radford AN. 2013. Noise negatively affects foraging and antipredator behaviour in shore crabs. Animal Behaviour 86:111e118. DOI: 10.1016/j.anbehav.2013.05.001.
- Waples DM, Thorne LH, Hodge LEW, Burke EK, Urian KW, Read AJ. 2013. A field test of acoustic deterrent devices used to reduce interactions between bottlenose dolphins and a coastal gillnet fishery. Biological Conservation 157:163–171. DOI: 10.1016/j.biocon.2012.07.012.
- Weilgart LS. 2007. The impacts of anthropogenic ocean noise on cetaceans and implications for management. Canadian Journal of Zoology 85:1091–1116. DOI: 10.1139/Z07-101.
- Weimann SR, Black AN, Leese J, Richter ML, Itzkowitz M, Burger RM. 2018. Territorial vocalization in sympatric damselfish: Acoustic characteristics and intruder discrimination. Bioacoustics 27:87–102. DOI: 10.1080/09524622.2017.1286263.
- Whitfield A, Becker A. 2014. Impacts of recreational motorboats on fishes: A review. Marine Pollution Bulletin 83:24–31. DOI: 10.1016/j.marpolbul.2014.03.055.
- Williams R, Wright AJ, Ashe E, Blight L, Bruintjes R, Canessa R, Clark C, Cullis-Suzuki S, Dakin D, Erbe C, Hammond PS, Merchant ND, O’Hara PD, Purser J, Radford AN, Simpson SD, Thomas L, Wale MA. 2015. Impacts of anthropogenic noise on marine life: Publication patterns, new discoveries, and future directions in research and management. Ocean & Coastal Management 115:17–24. DOI: 10.1016/j.ocecoaman.2015.05.021.
- Wong BB. 2014. Animal communication in a human-dominated world: A comment on Radford et al. Behavioral Ecology 25:1033. DOI: 10.1093/beheco/aru139.
- Wysocki LE, Codarin A, Ladich F, Picciulin M. 2009. Sound pressure and particle acceleration audiograms in three marine fish species from the Adriatic Sea. Journal of the Acoustical Society of America 126:2100–2107. DOI: 10.1121/1.3203562.