Abstract
Life cycle of the aquatic moth Cataclysta lemnata (Lepidoptera: Crambidae) was studied in laboratory conditions to obtain a basic biological knowledge useful for predicting the possible success of the herbivorous larvae of this insect as potential control agents in limiting the spread of the invasive American duckweed Lemna minuta (Alismatales: Araceae) in Italy. The multivoltinism of C. lemnata, as well as the high overall emergence from the pupal stage (85%), the high success in mating among the formed couples (>90%), and the high number of larvae born from each egg laying (on average 310 individuals), suggest that the insect can be successfully bred in the laboratory for the purposes of an augmentative biological control. Under experimental conditions, larvae developed in 23 days (through six larval instars, distinguishable by cephalic capsule dimensions) and pupae in 10, with no difference in duration between females and males. The larval phase resulted longer than the adult one (23 vs 10 days); therefore, it can be considered the most suitable stage for releasing the insect in the field for biocontrol purposes. Indeed, the larvae having a herbivorous diet might consume a large amount of the invasive plant, contrarily to the adult phase which is focused exclusively on reproduction. Our results not only contribute to the knowledge of aquatic Lepidoptera that are scarcely known, but also support the effectiveness of a possible protocol for an augmentative biological control of the invasive alien duckweed L. minuta.
1. Introduction
Lepidoptera is one of the richest and ubiquitous insect orders (Kristensen et al. Citation2007). They are commonly terrestrial, and only a small percentage is associated with aquatic environments. In fact, among approximately 165,000 Lepidoptera species that were described to date, only 800 (about 0.5% of the total) are aquatic (Pabis Citation2018). These Lepidoptera species live entirely underwater during the whole larval phase, while the winged adults have a subaerial life.
Aquatic Lepidoptera belong exclusively to two families: Arctiidae, with one single known aquatic species, Paracles laboulbeni (Bar, 1873), and Crambidae, very rich in aquatic species, which are mostly included in the Acentropinae subfamily. Acentropinae are known as the “China-mark” moths and include more than 700 aquatic species distributed worldwide, 12 of which occurring in Europe (Karsholt & Razowski Citation1996; Speidel Citation2005). Despite the unique characteristics of the aquatic Lepidoptera, they are one of the least studied groups within the order (Pabis Citation2018), probably because the lepidopterists are traditionally specialized in the study of terrestrial moths, while aquatic entomologists or limnologists, who are more likely to encounter these aquatic species, generally do not use equipment and sampling techniques suitable for adult moth handling and specific sample preparation (Mey & Speidel Citation2008).
Among the interesting aspects of these Lepidoptera, there is the role of their aquatic larvae as herbivores, which is important in ecological balances and interspecific interactions within freshwater ecosystems (Gross et al. Citation2002). Indeed, some of these species populate aquatic environments at such densities that have a considerable impact on some freshwater plant communities (Gross et al. Citation2001, Citation2002) and this feature could be used for biological control of invasive aquatic plants. For example, crambid larvae have often been evaluated as potential biocontrol agents of invasive aquatic plants, such as Salvinia molesta DS Mitchell in Australia (Buckingham Citation1994) and species of the genera Hydrilla and Elodea in Florida (Sands & Kassulke Citation1984; Julien & Bourne Citation1988).
Within this framework, the present study aims to contribute to the knowledge of aquatic Lepidoptera, by investigating the life cycle of the European aquatic moth Cataclysta lemnata (Linnaeus, 1758) (Lepidoptera: Crambidae). In particular, phenology, behavior, sex ratio, longevity and development of the larval, pupal and adult phases of this moth were investigated under laboratory conditions. The collection of such data on the biology of C. lemnata can provide the basic knowledge useful for evaluating the potential of this phytophagous insect as a natural enemy limiting the spread of the alien duckweed Lemna minuta Kunth (Alismatales: Araceae) in Italy. Lemna minuta is an aquatic plant considered highly invasive in many European countries, including Italy (DAISIE Citation2009; Ceschin et al. Citation2018), due to its high vegetative growth rate (Landolt Citation1986; Ceschin et al. Citation2016), and the severe impacts exerted by its free-floating mats on the invaded aquatic ecosystems (Dussart et al. Citation1993; Ceschin et al. Citation2019, Citation2020). Since the herbivorous aquatic larvae of C. lemnata have a diet that preferably includes native duckweeds, such as Lemna minor L. and Spirodela polyrrhiza (L.) Schleid., they might be also able to contain the spread of the alien duckweed, having proved to accept it as a new host plant and use it as food and material to build larval protective cases in water (Mariani et al. Citation2020a).
Results emerging from this study might support the effectiveness of a possible protocol for an augmentative biological control of the invasive alien duckweed L. minuta utilizing this moth species.
2. Materials and methods
2.1. Collection and rearing of Cataclysta lemnata
Cataclysta lemnata larvae were collected in waterbodies of Latium (Central Italy) from June to September 2018 and reared in the laboratory.
To be sure to deal with the species in question, identification of the collected specimens was done using taxonomic guides for aquatic Lepidoptera, in particular Vallenduuk and Cuppen (Citation2004) for larvae, and Goater (Citation1986) and Speidel (Citation1981, Citation2005) for adults. In addition, to confirm the identification, male and female genitalia of emerged moths were examined following Lee and Brown (Citation2006).
Field observations were conducted bimonthly to verify the presence of active larvae and adults in nature during the year.
The collected larvae of C. lemnata were placed inside glass tanks (40 × 34 × 27 cm), each filled with 20 l of tap water and covered with a fine-mesh net to prevent the winged adults to fly away. Larvae were fed with fresh plants of the alien duckweed L. minuta.
During the experiments, air and water temperature were in the range of 18–20°C and 16–19°C, respectively. These temperature conditions corresponded to those recorded in the field during the same seasonal period (Mariani et al. Citation2020b).
An oxygenator was immersed in each tank for keeping continuously oxygenated the still water according to the dissolved oxygen values found in the field (around 5 mg/l).
2.2. Mating and oviposition analysis
To study mating and oviposition behaviors, newly emerged adults were sexed according to their dimorphic features (Goater Citation1986; Speidel Citation2005). Then, male and female moths were combined into couples. Each couple was placed in a separate mating box, which was a transparent, cylindrical plastic container (5 cm in diameter, 7 cm in height) filled with 125 ml of tap water and a thin monolayer of L. minuta (2 g, fresh weight). To prevent adults from flying away, all mating boxes were closed with a cap, on which five holes (1 mm in diameter) were made to allow air flow.
For each experiment, five replicates were prepared, and observations were repeated six times for a total of 30 couples. Each couple could mate and oviposit within its own mating box. The produced eggs were photographed under a stereomicroscope (Olympus SZX2-ILLT) equipped with an Olympus OM-D EM-5 camera, and their dimensions (mean ± standard error) were measured by using the software ImageJ vers. 1.47. After the death of the female moth of each couple, Lemna plants on which the female had probably laid the eggs were transferred into transparent plastic boxes (21 × 28 × 12 cm) until the larvae emerged. After hatching, newly emerged larvae were counted and then transferred with a paintbrush into plastic boxes filled with fresh Lemna plants to start a new rearing cycle.
2.3. Larval stage analysis
Observations on the morphology of larvae and pupae were made under a stereomicroscope (Olympus SZX2-ILLT). The total number of larval instars was obtained by counting the number of exuviae released. In particular, since many insects eat the cuticle of their exuviae after moulting (Mira Citation2000), but usually avoid the sclerified cephalic capsules, the number of the latters was counted. For this procedure, 18 individuals at the very early instar were randomly selected among newly emerged larvae and moved into separate plastic containers supplied with L. minuta plants. Every 24 hours, the bottom of each container was sifted to find cephalic capsules and to determine their number and the duration of the instars. The capsules were collected with a paintbrush, photographed and measured by using the software ImageJ vers. 1.47. Observations on the development of each larva were made from larval etching to pupation. Stopping of plant consumption and fecal production was considered as an evidence of pupation.
2.4. Emergence and sex ratio studies
Pupae inside their cases (cocoons) were transferred into plastic containers (5 cm in diameter, 7 cm in height: one pupa per container) filled with 125 ml of tap water and closed at the top with a cap with 5 holes to allow air flow. Plastic containers were checked daily for observing the emergence of the moths, and the number of males and females was recorded. Observations were repeated on 10 larval cohorts for a total of 134 pupae.
2.5. Statistical methods
Differences in the average duration of the pupal stage and wingspan between males and females were assessed using Student’s t-tests. A two-way ANOVA was used to test if longevity was influenced by sex and/or mating. Data were checked for normality and homoscedasticity prior analyses. To test changes in the size of the cephalic capsules with instar, we first tested possible covariation between length and width. Since the two variables were strongly correlated (Spearman rank correlation coefficient, rs = 0.977, p << 0.001), we conducted the analysis only on the length values. Because of significant deviations from normality and homoscedasticity, a non-parametric approach was used to test differences in median values using a Kruskal-Wallace test followed by Mann-Whitney U-tests for pairwise post hoc comparisons with sequential Bonferroni corrections. Exact binomial tests were used to test if the sex ratio deviated significantly from 1:1. All analyses were conducted using R vers. 3.4.3 (R Development Core Team Citation2008). Errors refer to standard errors.
3. Results and discussion
3.1. Phenology of Cataclysta lemnata
Active larvae of C. lemnata were found in the field throughout the entire year, contrary to what was reported by Vallenduuk and Cuppen (Citation2004) who observed them only from May to October. In addition, the adults were observed in February, May and October, suggesting the species is multivoltine and therefore has multiple generations throughout the year.
Multivoltinism supports the possibility of using C. lemnata as a potential natural enemy for controlling the perennial mats of the alien L. minuta. Thanks to multivoltinism, the species might be continuously bred in the laboratory for a mass rearing finalized to periodic releases in the field, as required by an augmentative biocontrol protocol, which aims to control pest populations by increasing the populations of their native enemies (Hoy Citation2008). Moreover, the larvae would be able to feed on the alien plant all year round, ensuring efficiency and continuity in the containment of its growth.
3.2. Biological cycle of Cataclysta lemnata
3.2.1. Egg stage
Egg morphology. As soon as the eggs were laid, they were quite flattened ()), but thickened during maturation. Mature eggs were dorso-ventrally compressed and elliptical. Their dimensions are shown in .
Figure 1. Newly laid eggs (a), 72 hours (b), 48 hours (c), 1 hour (d) before hatching. Larva perforating the chorion (e) and coming out of the eggshell first with the head (f) and then with the rest of the body (g) (Photos by F. Mariani)
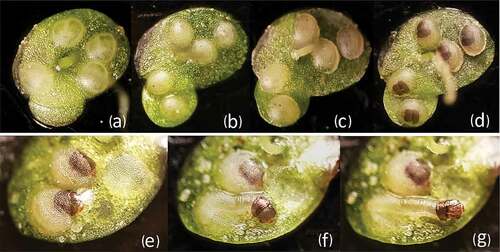
Table I. Biological data for C. lemnata. Dimensions of eggs, larval head capsules, cases and wingspan of adults are reported
Egg maturation
The chorion was finely wrinkled and transparent, which allowed direct observation of the embryonic development. Seventy-two hours before hatching, the embryo was transparent, but two lateral dark dots on the head (corresponding to the eyes of the future larva) began to appear together with two translucent and curled tracheal tubes running longitudinally along pleural areas of the body ()).
Forty-eight hours before hatching, the embryo’s mandibles became visible ()), while 24 hours before, the whole cephalic capsule began to darken, becoming more dark brown as time passed. When approaching the hatching moment, the larvae became clearly distinguishable and appeared curled up inside the egg ()). Within a few minutes before hatching, larvae started to actively move inside the eggs, opening and closing their mandibles. Finally, they perforated the chorion with the mandibles and came out of the eggshell (). All eggs belonging to the same oviposition hatched approximately together, about 6 days after laying.
3.2.2. Larval stage
Development and morphology of larval instars. Although four larval instars are usually reported for C. lemnata (Van Der Velde Citation1988), in this study six larval instars (I–VI) were distinguished on the basis of the number of cephalic capsules found (). The cephalic capsule of the last larval stage was always found inside the cocoons of the pupa, broken in several pieces due to the metamorphosis, so it was not possible to measure its size. The duration of the entire larval stage and each instar is shown in .
Table II. Development times (days) for C. lemnata. Development time of eggs, larvae, pupae and longevity of adults are reported
Morphological features were different among the six larval instars. In the larvae belonging to the I instar, the body was transparent and about 1.3 mm long. One evident whitish, longitudinal tracheal tube on each side was present, and some setae occurred in longitudinal rows dorsally and along each side of the abdomen. The cephalic capsule with black stemmata, the epicranial suture and the pronotal shield (about equal in width to cephalic capsule, ) were brown. The mandibles were reddish-brown in color.
From instar II to VI, the body became dark brown in color, while the cephalic capsule was whitish; the body length increased with the advancing of the instars up to a maximum length of 18 mm in the last instar.
Median size (length) of cephalic capsules varied significantly among instars (Kruskal-Wallace median test, χ2 = 113, df = 4, p ≪ 0.001), and all post hoc tests were significant (Mann-Whitney U-tests, U = 0, p ≪ 0.001 after Bonferroni correction in all cases). Different instars showed perfectly non-overlapping ranges in cephalic capsule lengths ().
Figure 2. Box plots (median, 25%-75% and range) of lengths (µm) of cephalic capsules of Cataclysta lemnata at different instars. Different colors indicate significant differences. The sixth instar is not represented because it was not possible to measure the size of the cephalic capsules
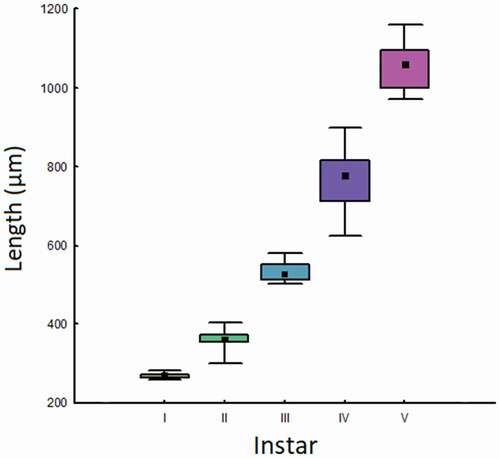
This result is particularly important since it allows direct identification of the age of larvae collected in the field and the knowledge of the exact timing of their development. In fact, overall body length is scarcely informative for instar identification, since the soft membranous cuticle of caterpillars is quite stretchable and can vary depending on nutrition state. Even the size of the larval cases is widely variable and therefore not useful to establish the instar. By contrast, the cephalic capsule remains constant for each larval instar and therefore allows establishing the exact larval instar. Knowing the exact larval instar can be important also for choosing the optimal instar to release in the field for controlling the overgrowth of the alien L. minuta.
Behaviour
Newly emerged larvae () lived completely submerged (hydrophilic phase) and their respiration was guaranteed by direct diffusion of the dissolved oxygen in water through their thin integument, since they do not have tracheal gills and their spiracles are closed.
Figure 3. First-instar larva beginning the construction of its case (a), hydrophilic larva (b), hydrophobic larva partially out of its case with visible plastron around the body (c), hydrophobic advanced-instar larva without case (Photos by F. Mariani)

Neonate larvae immediately started to eat duckweed plants, which changed the color of their digestive system to green, which was visible through their transparent body ()). Duckweed fresh plants were utilized by these larvae also as material for building their protective cases and this construction started from the first moment of life outside the eggshell ()), a finding that is not in accordance with the observations of Gromysz-Kalkowska and Unkiewicz-Winiarczyk (Citation2011) who report that the construction begins 5 days after hatching. From this point on, the case construction continued unceasingly throughout the whole larval period by adding progressively new Lemna plants over the external surface of the case ()), while the inner one is coated with a thin layer of hydrophobic silk ()). The result is a tubular case formed of Lemna plants held together by the sticky silk produced by the larval spinneret (). The case size increased as the larvae grow and ranged from 2 mm to 2 cm in the last larval instar. As the larval body size increased, larvae consume large amounts of Lemna more rapidly, and this positive correlation was also demonstrated in a recent study (Mariani et al. Citation2020a). This results in greater consumption of plants by late-instar larvae that therefore could be the best larval instar to start a containment effort of the invasive alien duckweed in the field.
Figure 4. Protective case (a), inner portion of the case with hydrophobic larva (b) (Photos by F. Mariani)
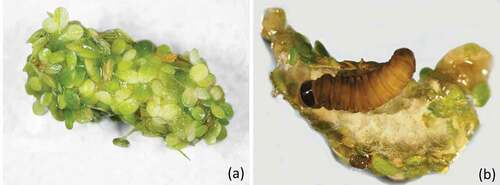
The larvae floated on the water surface inside their cases, which were found to be open at both ends; in fact, it was observed that the larva generally protruded half or more of its body from one end of the case to look for food, while it used the other end to extend the hind part of the abdomen to defecate.
The cases play different important roles for larval life. They not only facilitate floating and respiration under water and protect the larvae from UV radiation (Dorn et al. Citation2001), but also provide protection from predators or parasites (Müeller & Dearing Citation1994); for example, Wojtusiak and Wojtusiak (Citation1960) showed that C. lemnata larvae without a case were much more susceptible to being attacked by predators, such as carp, tench and roach.
With the first moult, the hydrophobic larval phase begins, and this passage was highlighted by the presence of a highly hydrophobic cuticle that showed an array of hairs forming the plastron around the body, with exclusion of the cephalic capsule, pronotal shield and legs ()). The formation of the plastron in C. lemnata was already observed by Pabis (Citation2014) and it proves to be a very common adaptation in Acentropinae (Speidel Citation2005).
An unusual peculiar behavior from larvae in hydrophobic phase was observed in our study. We found that sometimes these larvae descended beneath the water level by adhering to the surface of the container, reaching also a depth of 10 cm. Their descent often stopped when their legs lose adherence with the vertical surface, causing a very fast ascent towards the water level. This behavior should be energetically very expensive for the tiny larvae; in fact, the presence of floating Lemna plants on their cases, their hydrophobic cuticle and the air layer that surrounds their bodies make it easier for the larvae floating than diving. However, it is not yet clear if this behavior is performed only under laboratory conditions or if it is part of a more complex natural strategy to disperse and possibly reduce competition in cases of overcrowding on the water surface.
3.2.3. Pupal stage
General features. Cataclysta lemnata pupae were reddish brown in color and approximately 7 mm in length. The body was narrow, elongate, with 3 large, functional spiracles on abdominal segments II–IV with thick, protruding peritremes; the other five abdominal spiracles were closed. The presence of raised and chimney-like spiracles on abdominal segments II–IV is a morphological feature occurring in all Acentropinae species (Speidel Citation1981; Passoa Citation1988).
Pupation occurred in the larval cases, which have been adapted to pupal cases (cocoons), floating freely on the water surface, which were internally characterized by a white silken septum that divided the inner part of the case into two portions, a smaller one containing the pupa and the exuvia of the last larval instar, and a larger one that was empty and very probably has the function of facilitating the buoyancy of the case and providing air for the pupa.
The case of the pupa had a nearly oval shape and it was very compact, with the plants firmly attached to each other.
Development
The average duration of the pupal stage did not vary between sexes [average ± SE: 9.613 ± 0.364 days in females (range: 6–15 days, n = 31), 10.487 ± 0.320 days in males (range: 7–16 days, n = 39); Student’s t-test: t = 1.806, df = 68, p = 0.075]. Additional data on pupal development are presented in .
Adult emergence from the pupal stage was recorded in 115 out of 134 pupae (86%). This high percentage argues in favor of the hypothesis of using this insect as a natural agent to control the invasive alien duckweed, as it proves to be easy to laboratory mass-production.
3.2.4. Adults
Coloration, habitus and behavior. Adult insects were sexually distinguishable on the basis of different wing chromatic pattern, size, and behavior. Males showed light brown head and thorax, and wings off-white in color, with brown or tan bands; females had brown head and thorax, and wings dark-brown in color, with lighter hindwings than the forewings and with patterns similar to those of males; both sexes were characterized by the presence of a darker area on the edge of the hindwings, crossed centrally by a dark brown-black band with brilliant white spots. Females had a wider wingspan than males (, Student’s t-test: t = 14.191, df = 60, p < 0.001), a more robust abdomen, and antennae shorter than males. Regarding the behavior, males were more active and flew more often than females, which were almost always immobile and remained closer to the water level.
Emergence and sex ratio
During the emergence, the adults never came into contact with the water, and thus the moths came out of the floating cases directly above the water level. This behavior is in contrast with that observed in other aquatic Crambidae, such as the wingless adult females of Acentria ephemerella ([Denis & Schiffermüller], 1775), which live in water (Berg Citation1941; Ward Citation1992), and Petrophila confusalis (Walker, 1866), whose females, in order to lay the eggs, submerge completely, press the wings against their body and remain surrounded by an air layer, resisting even several hours underwater (Tuskes Citation1977).
As for the sex ratio of C. lemnata, neither the overall sex ratio, nor those relative to the various cohorts, differed significantly from 1:1 (Exact binomial tests: p > 0.05) (), a condition very common in insect species (Price Citation1997; Hardy Citation2002; Sapir et al. Citation2008). Knowing population sex ratio is important to understand, and eventually to predict, the reproductive success of it (Weir et al. Citation2011). This becomes even more important in the application of biological control programs that require sex ratio values in the biocontrol agent population adequate to determine what is its optimal sex ratio for a successful mass rearing (Fitz-Earle & Barclay Citation1989).
Table III. Sex ratio and percentage emergence of moths. P values refer to binomial tests
Mating and oviposition. Mating occurred in all the couples formed (30 out 30), but observation of sexual dynamics was only possible in a third of these couples, when they did not occur overnight. Among these, in six couples the copulation started from the first moment of formation of the couple, with the male immediately flying towards the female to begin mating. In the remaining couples, before mating, moths waited from 2 to 5 hours.
Contrary to Pabis (Citation2014), who observed that copulations occurred mainly at the surface of the water covered with Lemna mats, in all the couples observed in this study, mating always occurred on the vertical surfaces of the containers, with the male bringing its abdomen close to that of the female moth, and oriented in the opposite direction to the female ()). Duration of copulations varied from 15 to 25 minutes (mean = 21 minutes). Only in one couple, the copulation occurred twice, each lasting around 15 minutes. These laboratory observations do not agree with the field observations of Pabis (Citation2014), who reports 40-minute mating. The discrepancies recorded between our laboratory observations and Pabis’ field observations could be due both to differences in microclimatic conditions, and to the different target plant species, that is, Lemna minuta and Lemna minor, respectively.
When the copulation ended, females waited a variable time before starting to lay eggs: in 33% of the cases observed, they waited only 25 minutes, otherwise 24 hours or even more time. The oviposition times ranged from 15 minutes to even 48 hours. These oviposition times appear much longer than those estimated by Pabis (Citation2014), who reported 15–20 minutes for the complete egg laying. The adult females were found to walk on the Lemna layer for hours, stopping at some points and immersing their ovipositor into the water to attach from two to five eggs on the submerged part of each Lemna plant ()). The oviposition stage is the only time when the C. lemnata moths had a minimal contact with water.
During oviposition, the females were poorly reactive and this factor, together with the long time needed for the oviposition, could make them more vulnerable to the attacks of possible predators. The oviposition often ended with the death of the female, which remained lying on Lemna mat.
Fecundity. The number of larvae born from each oviposition was on mean (± SE) 310.393 ± 19.022 (range 194–496, n = 30). Mating and oviposition were successful in 93% of the couples formed, and only 7% of the females laid non-fertilized eggs that appeared as small gelatinous masses whiter than the fertilized ones.
Longevity. Males had a higher longevity (average ± SE: 10.806 ± 0.722 days, range 2–19 days, n = 36) than females (8.115 days ± 0.378 days, range 5–13 days, n = 26), and individuals that had mated had a higher longevity (10.694 ± 0.553 days, range = 6–19 days, n = 36) than those that did not mate (8.269 ± 0.770 days, range = 2–18 days, n = 26), regardless of the sex (lack of significant interaction in the ANOVA) (see ).
Table IV. Results of the two-way ANOVA for differences in life duration according to sex and fecundation. SS = Sum of Squares, df = degrees of freedom, MS = Mean Squares, F = Fisher’s F.
4. Conclusions
Although based on laboratory observations, our results not only contribute to the knowledge of the biology of aquatic Lepidoptera that are scarcely known, but also support the effectiveness of a possible protocol for an augmentative biological control of the invasive duckweed L. minuta. The multivoltinism of C. lemnata, as well as the high overall emergences from the pupal stage, the high success in mating among the formed couples, and the high realized fecundity suggest that the insect can be successfully bred in the laboratory.
The relative long larval stage of C. lemnata (23 days on average) further supports the hypothesis of using this insect as biocontrol agent of the alien duckweed, since the herbivorous larvae are voracious consumers of this duckweed, especially at the medium-late instars (Mariani et al. Citation2020a), which also are the larval instars with the longest duration.
By contrast, the adult phase has a short lifespan, during which the female moths are mainly dedicated after the mating to laying their eggs. Therefore, in an active biological control program, C. lemnata adults are not the most suitable for being released in the field. Instead, an appropriate protocol should start with the release of larvae, so as to obtain an initial success in the removal of L. minuta biomass through the larval herbivory action, but especially to ensure that the larvae have time to adapt to the new environmental conditions, pupate and emerge as winged adults. Also the pupae might be a convenient stage to release, which would soon produce adults capable of rapidly distributing their eggs on the Lemna mats. In this way, the short lifespan of the winged adults could be entirely dedicated to the reproduction since the first moment after the emergence and would not be disturbed or stressed by the transport from the laboratory to the field. However, further studies will be necessary to understand which is the best sex ratio for C. lemnata to increase larval production in laboratory colonies, and to know the dispersion capacity of this species. In addition, it is necessary to further investigate the main predators of C. lemnata larvae and the actual predation pressure on them, in order to ensure that the attempt to contain L. minuta in the field is not nullified by the action of aquatic predators, such as macroinvertebrates or fish.
Acknowledgements
The authors are grateful to the staff of the Regional Park of Appia Antica (Rome, Italy) and two trainees of the University of Roma Tre, Dario Raimondi and Alice Rimessi, for their support during fieldwork and data collection.
Disclosure statement
No potential conflict of interest was reported by the authors.
Additional information
Funding
References
- Berg K. 1941. Contributions to biology of the aquatic moth Acentropus niveus (Oliv.). Videnskabelige Meddelelser Naturhistorisk Forening I København 105:59–139.
- Buckingham GR. 1994. Biological control of aquatic weeds. In: Rosen D, Bennett FD, Capinera JL, editors. Pest management in the subtropics: Biological control - a Florida perspective. Andover: Intercept Ltd. pp. 413–480.
- Ceschin S, Abati S, Ellwood NTW, Zuccarello V. 2018. Riding invasion waves: Spatial and temporal patterns of the invasive macrophyte Lemna minuta arrival and spread across Europe. Aquatic Botany 150:1–8. DOI: 10.1016/j.aquabot.2018.06.002.
- Ceschin S, Abati S, Traversetti L, Spani F, Del Grosso F, Scalici M. 2019. Effects of the invasive duckweed Lemna minuta on aquatic animals: Evidence from an indoor experiment. Plant Biosystems - an International Journal Dealing with All Aspects of Plant Biology 153(6):749–755. DOI: 10.1080/11263504.2018.1549605.
- Ceschin S, Della Bella V, Piccari F, Abati S. 2016. Colonization dynamics of the alien macrophyte Lemna minuta Kunth: A case study from a semi-natural pond in Appia Antica Regional Park (Rome, Italy). Fundamental And Applied Limnology / Archiv für Hydrobiologie 188(2):93–101. DOI: 10.1127/fal/2016/0870.
- Ceschin S, Ferrante G, Mariani F, Traversetti L, Ellwood NTW. 2020. Habitat change and alteration of plant and invertebrate communities in waterbodies dominated by the invasive alien macrophyte Lemna minuta Kunth. Biological Invasions 22(4):1325–1337. DOI: 10.1007/s10530-019-02185-5.
- DAISIE. 2009. Handbook of alien species in Europe. Berlin: Springer.
- Dorn NJ, Cronin G, Lodge DM. 2001. Feeding preferences and performance of an aquatic lepidopteran on macrophytes: plant hosts as food and habitat. Oecologia 128:406–415. https://doi.org/10.1007/s004420100662
- Dussart G, Robertson J, Bramley J. 1993. Death of a lake. Biological Sciences Reviews 5(5):8–10.
- Fitz-Earle M, Barclay HJ. 1989. Is there an optimal sex ratio for insect mass rearing? Ecol. Model. 45(3):205–220. https://doi.org/10.1016/0304-3800(89)90082-3
- Goater B. 1986. British pyralid moths: A guide to their identification. Colchester: Harley Books.
- Gromysz-Kalkowska K, Unkiewicz-Winiarczyk A. 2011. Ethological defense mechanisms in insects. II. Active Defense. Annales Universitatis Mariae Curie-Sklodowska 66(1):143–153.
- Gross E, Johnson RL, Hairston N. 2001. Experimental evidence for changes in submersed macrophyte species composition caused by the herbivore Acentria ephemerella (Lepidoptera). Oecologia 127:105–114. DOI: 10.1007/s004420000568.
- Gross EM, Feldbaum C, Choi C. 2002. High abundance of herbivorous Lepidoptera larvae (Acentria ephemerella DENIS and Schifermüller) on submersed macrophytes in Lake Constance (Germany). Fundamental and Applied Limnology 155:1–21. DOI: 10.1127/archiv-hydrobiol/155/2002/1.
- Hardy ICW. 2002. Sex ratios: Concepts and research methods. Cambridge: Cambridge Univ Press.
- Hoy MA. 2008. Augmentative biological control. In: Capinera JL, editor. Encyclopedia of entomology. Dordrecht: Springer. pp. 327–334. DOI: 10.1007/978-1-4020-6359-6_10394.
- Julien AMH, Bourne S. 1988. Effects of leaf-feeding by larvae of the moth Samea multiplicalis Guen. (Lep., Pyralidae) on the floating weed Salvinia molesta. Journal of Applied Entomology 106(5):518–526. DOI: 10.1111/j.1439-0418.1988.tb00627.x.
- Karsholt O, Razowski J. 1996. The Lepidoptera of Europe. A distributional checklist. Stenstrup: Apollo Books.
- Kristensen NP, Scoble MJ, Karsholt O. 2007. Lepidoptera phylogeny and systematics: The state of inventorying moth and butterfly diversity. Zootaxa 1668:699–747. DOI: 10.11646/zootaxa.1668.1.30.
- Landolt E. 1986. The family of Lemnaceae - a monographic study. 1. Zürich: Veröffen. des Geobot. Inst. der ETH, Stiftung Rübel.
- Lee SM, Brown RL. 2006. A new method for preparing slide mounts of whole bodies of microlepidoptera. J. Asia Pac. Entomol 9(3):249–253.
- Mariani F, Di Giulio A, Fattorini S, Ceschin S. 2020a. Experimental evidence of the consumption of the invasive alien duckweed Lemna minuta by herbivorous larvae of the moth Cataclysta lemnata in Italy. Aquatic Botany 161:103172. DOI: 10.1016/j.aquabot.2019.103172.
- Mariani F, Ellwood NWT, Zuccarello V, Ceschin S. 2020b. Compatibility of the invasive alien Lemna minuta and its potential biocontrol agent Cataclysta lemnata. Water 12(10):2719. DOI: 10.3390/w12102719.
- Mey W, Speidel W. 2008. Global diversity of butterflies (Lepidoptera) in freshwater. Hydrobiologia 595:521–528. DOI: 10.1007/978-1-4020-8259-7_50.
- Mira A. 2000. Exuviae eating: A nitrogen meal? J. Journal of Insect Physiology 46(4):605–610. DOI: 10.1016/s0022-1910(99)00146-8.
- Müeller UG, Dearing MD. 1994. Predation and avoidance of tough leaves by aquatic larvae of the moth Parapoynx rugosalis (Lepidoptera: Pyralidae). Ecological Entomology 19:155–158. DOI: 10.1111/j.1365-2311.1994.tb00405.x.
- Pabis K. 2014. Life cycle, host plants and abundance of caterpillars of the aquatic moth Cataclysta lemnata (Lepidoptera: Crambidae) in the post-glacial lake in Central Poland. North-Western Journal of Zoology 10(2):441–444. Available: http://biozoojournals.ro/nwjz/content/v10n2/nwjz_142101_Pabis.pdf.
- Pabis K. 2018. What is a moth doing under water? Ecology of aquatic and semi-aquatic Lepidoptera. Knowledge & Management of Aquatic Ecosystems 419:42. DOI: 10.1051/kmae/2018030.
- Passoa S. 1988. Systematic positions of Acentria ephemerella (Denis & Schiffermüller), Nymphulinae, and Schoenobiinae based on morphology of immature stages (Pyralidae). Journal of the Lepidopterists Society 42:247–262.
- Price PW. 1997. Insect Ecology. New York: John Wiley and Sons.
- R Development Core Team. 2008. R: A language and environment for statistical computing. R Foundation for Statistical Computing, Vienna. http://www.R-project.org
- Sands DPA, Kassulke RC. 1984. Samea multiplicalis (Lep.: Pyralidae), for biological control of two water weeds, Salvinia molesta and Pistia stratiotes in Australia. Entomophaga 29:267–273. DOI: 10.1007/BF02372113.
- Sapir Y, Mazer SJ, Holzapfel C. 2008. Sex ratio. In: Erik JS, Brian F, editors. Encyclopedia of ecology. Oxford: Academic Press. pp. 3243–3248.
- Speidel W. 1981. Die Abgrenzung der Unterfamilie Acentropinae (Lepidoptera, Pyraloidea) (in German). Atalanta 12:117–129.
- Speidel W. 2005. Acentropinae. In: Goater B, Nuss M, Speidel W, editors. Pyraloidea I (Crambidae: Acentropinae, Evergestinae, Heliothelinae, Schoenobiinae, Scopariinae). Microlepidoptera of Europe 4. Stenstrup: Apollo Books. pp. 73–82.
- Tuskes PM. 1977. Observations on the biology of Parargyractis confusalis, an aquatic pyralid (Lepidoptera: Pyralidae). The Canadian Entomologist 109:695–699. DOI: 10.4039/Ent109695-5.
- Vallenduuk HJ, Cuppen HMJ. 2004. The aquatic living caterpillars (Lepidoptera: Pyraloidea: Crambidae) of Central Europe. A key to the larvae and autecology. Lauterbornia 49:1–17.
- Van Der Velde G. 1988. Cataclysta lemnata L. (Lepidoptera, Pyralidae) can survive for several years consuming macrophytes other than Lemnaceae. Aquatic Botany 31:183–189. DOI: 10.1016/0304-3770(88)90048-4.
- Ward JW. 1992. Aquatic insect ecology. New York: John Wiley and Sons.
- Weir LK, Grant JWA, Hutchings JA. 2011. The influence of operational sex ratio on the intensity of competition for mates. Am. Nat. 177(2):167–176. https://doi.org/10.1086/657918
- Wojtusiak H, Wojtusiak RJ. 1960. Biologia, występowanieiuz ytkownos ć motyli wodnych z podrodziny Hydrocampinae w stawach doswiadczalnych (in Polish). Polish Archives of Hydrobiology 8:253–260.