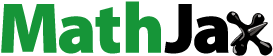
Abstract
Longhorn beetles (Coleoptera: Cerambycidae) is one of the largest, most diverse, ecologically and economically important groups of beetles in the world. In the following paper, through a comprehensive synthesis and review of various sources of data, we have described the general patterns of the distribution of longhorn beetles on the global scale. We found that the vast majority of Cerambycidae diversity is concentrated in two hyper–diverse subfamilies: Lamiinae and Cerambycinae, which together cover 90.5% of all longhorn beetles. Nearly 60% of the global fauna of Cerambycidae is distributed within the Oriental or Neotropical realms. Although the monospecific genera are predominated, most of the taxonomic diversity of longhorn beetles is concentrated on the limited number of large, multi–species genera; only the first 829 richest genera encompass three quarters of the total number of Cerambycidae species. Although the estimated number of described longhorn beetle species (34,490) showed a good agreement with other studies, that figure seems to be far from the actual number, since more than 200 new species are described every year in the recent decade. Most newly–described species originate from Asia and Southern America. The vast majority (88.1%) of the Cerambycidae species were found to be restricted to only one biogeographic realm. The highest number of endemic species can be found in cerambycid fauna of the Australasian, Ethiopian, Madagascan and Neotropical realms. Moreover, our findings highlight the phylogenetic distinctness of the Madagascan fauna of Cerambycidae. More research involving a comprehensive multigene approach is needed to resolve urgent issues regarding both the higher and lower level taxonomy of Cerambycidae and to better understand the factors influencing the distribution and diversification of cerambycids.
Introduction
Coleoptera (beetles) represents almost one-quarter of all currently known animal species. Hence, the understanding of their global diversity and distribution patterns is crucial, especially in the face of a sixth mass extinction crisis (Barnosky et al. Citation2011; Ceballos et al. Citation2015). Within coleopteran families, Cerambycidae (longhorn beetles) is one of the most species–rich, diverse, ecologically, and economically important group, and in addition, many longhorn beetles are considered endangered due to both habitat loss and habitat alterations (Jeppsson & Forslund Citation2014; Seibold et al. Citation2015).
According to recent estimations, as many as 35,000 extant cerambycid species subdivided into nearly 4,000 genera were described globally (Švácha & Lawrence Citation2014; Wang Citation2017). Longhorn beetles are exclusively phytophagous, and their larvae are mostly internal feeders of living or dead plant tissues (Ślipiński & Escalona Citation2016). Cerambycid species play a crucial ecological role, especially in forest habitats where they are one of the major groups of decomposers of dead plant matter. Moreover, longhorn beetles pollinate numerous species of herbaceous plants, shrubs and trees, and serve as an important food resource for a large number of vertebrates (Ślipiński & Escalona Citation2016; Wang Citation2017; Haddad et al. Citation2018). On the other hand, some cerambycid species are considered serious pests in agriculture, horticulture and forestry. Cerambycid larvae feeding habits can significantly weaken cultivated plants or even be fatal to the hosts, leading to an enormous economic loss globally (Wang Citation2017). Some longhorn beetles are also known to be the vectors of dangerous plant pathogens like nematodes and fungi (Jankowiak & Rossa Citation2007; Akbulut & Stamps Citation2012). Furthermore, in recent decades of the progressive globalization of global trade, many cerambycid species have been intercepted outside their native ranges, and some of them have established new populations causing serious ecological and economic issues (Haack Citation2006).
Despite the unquestionable ecological and economical significance of longhorn beetles, a comprehensive, global-scale synthesis on cerambycid distribution and diversity patterns is still lacking. Existing summaries have focused on particular taxonomic groups (Monné Citation2005a; Wallin et al. Citation2013; Lingafelter Citation2015; Huang et al. Citation2020) or faunae of particular region (Bílý & Mehl Citation1989; Sama Citation2002; Monné Citation2005b; Spomer Citation2014; Danilevsky Citation2014; Kariyanna et al. Citation2019), which prevents a comparison of longhorn beetle diversity patterns at a global scale.
The main aim of this paper was to summarize the current knowledge on the worldwide taxonomic diversity and distribution of longhorn beetles. Comprehensive revision and synthesis of data deposited in databases, catalogues, scientific papers, and checklists allowed us to provide new insights into the general pattern of the distribution and taxonomic diversity longhorn beetles.
Material and methods
Data sources and record selection procedure
As a basic source of information on the global taxonomic diversity of longhorn beetles, we used the “Titan” database (Tavakilian & Chevillotte Citation2019) founded by the Institut de Recherche pour le Développement (IRD) (MNHN, Paris, France) which constitutes the most comprehensive and up–to–date information about the worldwide cerambycid species (Tavakilian & Chevillotte Citation2019). For the next step, we critically revised the whole database in order to exclude duplicates, species with invalid taxonomic statuses, identify synonymous species, and to also take into account taxa not included in this database. We have achieved it by confronting the content of the “Titan” database with other sources such as checklists, catalogues, and taxonomic revisions (Monné Citation2005a, Citation2005b, Citation2006; Danilevsky Citation2012; Ślipiński & Escalona Citation2016; Wang Citation2017; Bezark Citation2019; Pirkl Citation2019; Roguet Citation2019; Vitali Citation2019). In the case of data incompatibility between the compared data sources, each unclear record was manually evaluated prior to inclusion in analyses by using the same record selection protocol (). Especially, we checked synonyms and the correctness of the name spelling. In the case of special difficulties in determining the taxonomic status, we relied on the latest revision of the particular taxa published in the peer–reviewed scientific journal. For this reason, we used a simple query in the Google Scholar search engine (https://scholar.google.co.uk): revision “Genus_name species_name”, sorted results by date, and manually evaluated whether the publications found are in fact taxonomic revisions of the species of interest, and if the revision was published in peer–reviewed scientific journal.
Given that many aspects of longhorn beetles taxonomy are still controversial (Haddad & Mckenna Citation2016; Ślipiński & Escalona Citation2016; Wang Citation2017) and there are numerous disputes regarding the higher level taxonomy (e.g. subfamilies and tribes classification) of the group, and because the presented study was not aimed at elucidating taxonomic ambiguities within longhorn beetles, we have decided to adopt one of the most recent and widely applied system of the beetles’ subfamilies classification (Bouchard et al. Citation2011), which recognized up nine subfamilies of Cerambycidae including Apatophyseinae Lacordaire, Cerambycinae Latreille, Dorcasominae Lacordaire, Lamiinae Latreille, Lepturinae Latreille, Necydalinae Latreille, Parandrinae Blanchard, Prioninae Latreille, and Spondylidinae Audinet–Serville. The other groups within the traditional “cerambycoid assemblage’ (Cerambycidae s.l.) (Haddad & Mckenna Citation2016; Haddad et al. Citation2018) were not included in the analyses, and only basic information about the number of species in the families: Oxypeltidae Lacordaire, Vesperidae Mulsant, and Disteniidae J. Thomson were given.
Data analysis
Each of the species were assigned to one (or more) biogeographic realms (Newton Citation2003) and to one (or more) continents (Australia [mainland] and Oceania were separated), based on the distribution data found in the explored databases, catalogues, or scientific papers (Monné Citation2005a, Citation2005b, Citation2006; Danilevsky Citation2012; Ślipiński & Escalona Citation2016; Wang Citation2017; Bezark Citation2019; Pirkl Citation2019; Roguet Citation2019; Tavakilian & Chevillotte Citation2019; Vitali Citation2019). In rare cases when a species was found to have occurred directly on the border of two areas (realms or continents), and the data on its distribution were not precise enough to determine its affiliation to one of the specific areas, the species was assigned to both border areas. The year of the description and the name of the discoverer have been assigned for each species. The compliance with the normal distribution was tested using the Shapiro–Wilk normality test (Shapiro & Wilk Citation1965) in STATISTICA v. 13 software (StatSoft Inc Citation2017). As a measure of the distinctness of the Cerambycidae fauna of particular realms and continents, we applied the widely–used Jaccard Dissimilarity Index (Real & Vargas Citation1996; Carvalho et al. Citation2013; Gergócs & Hufnagel Citation2015):
where a is is the number of genera shared by the two units (realms or continents), b is is the number of genera present only in unit 1, and c is is the number of genera occurring only in unit 2. The Jaccard Dissimilarity Index adopts values between 0 and 1; where value ‘0ʹ indicates that the fauna of the two compared units are identical, whereas value ‘1ʹ indicates that the two compared regions do not have any species in common. The matrix of the Jaccard Dissimilarity Index was then used in the cluster analyses with the Unweighted Pair–Group Method with Arithmetic Averages (UPGMA) in MEGA–X v. 10.1.8 software (Kumar et al. Citation2018).
Results
Taxonomic diversity
According to our estimations, there are 34,490 described species of Cerambycidae s.s. worldwide (March 2019). Whereas their close congeners within the traditional “cerambycoid assemblage” (see Materials and Methods section) encompass only 460 species (March 2019) subdivided into three families: Oxypeltidae (3 species, 1 subfamily), Vesperidae (68 species, 3 subfamilies), and Disteniidae (389 species, 1 subfamily) ().
Table I. The summary of species richness in the families Oxypeltidae, Vesperidae, Disteniidae and Cerambycidae (March 2019)
Most of the Cerambycidae s.s. diversity is concentrated in two hyper–diverse subfamilies: Lamiinae – which contains 20,036 species and constitutes as many as 58% of all longhorn beetle species, and Cerambycinae with 11,172 species which covers 33% of cerambycid species diversity. The other subfamilies of Cerambycidae are less numerous (, ). The subfamily Lepturinae contains 1,500 species which constitutes 4% of all known cerambycids, whereas Prioninae encompasses 1,058 species and covers only 3% of extant longhorn beetle species (). The total contribution of the remaining subfamilies is as follows: Parandrinae (123 species), Apatophyseinae (308 species), Spondylidinae (147 species), Necydalinae (124 species), and Dorcasominae (22 species). Given together, aforementioned families do not exceed 3% of the global species diversity of Cerambycide. The analyses of the frequency of distribution of Cerambycidae species richness was conducted separately for each subfamily, and indicated consistently significant, right–skewed distributions () which clearly deviated from the normal distribution with the mode equal to one ().
Table II. Frequency of distribution of Cerambycidae s.s. species richness
Figure 2. The percentage contribution of subfamilies of Cerambycidae s.s. to the total species diversity of this group
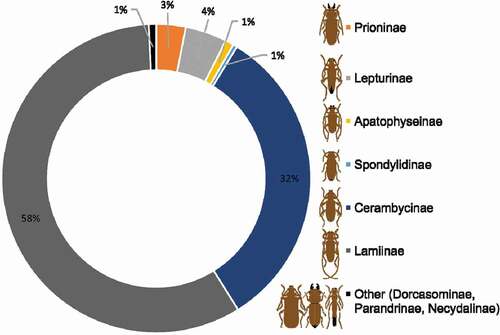
Figure 3. Histograms of the frequency of distribution of Cerambycidae s.s. species diversity. The top left histogram shows the overall frequency of distribution of species per genera in the whole Cerambycidae s.s. family, while the others indicate species distribution in 8 out of 9 subfamilies of Cerambycidae s.s (the smallest subfamily – Dorcasominae not included). The y axis indicates the cumulative number of genera containing the particular number of species, while the x axis shows the number of species in the genus (the original values ordered from the poorest to the most species-rich genera)
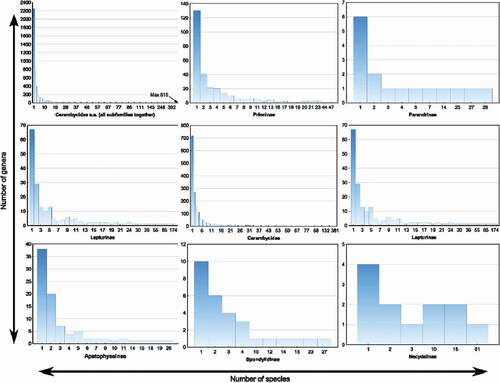
Although the Cerambycidae family encompasses a large number of genera (4,959), most of the species richness in this group is contained in the first 1,000 of the richest genera (78.32%). As many as 50% of the global species diversity of Cerambycidae accounts for the first 215 richest genera, while the first 829 richest genera contained 75% of the total global richness of longhorn beetles (). Although single–species genera were predominant (2253 single–species genera), they contained together only 6.5% of the total species richness of longhorn beetles. The top five of richest genera of Cerambycidae belonged to the Lamiine subfamily, and included: genus Pterolophia Breuning, 1961, Glenea Jordan, 1894, Sybra Hayashi, 1956, Dorcadion Ganglb., 1884, Exocentrus Dejean, 1835 (). The richest genus in the second-largest subfamily of Cerambycidae – Cerambycinae was the Demonax Thomson, 1861 genus. Other Cerambycidae genera did not exceed 200 species ().
Table III. The most species-rich genera of Cerambycidae s.s.
Figure 4. The accumulation curve (solid blue line) of the global Cerambycidae s.s. species richness in the following genera ordered from the richest one (Pterolophia Breuning, 1961; 815 species) to the poorest genera containing single species. As many as 50% of the global species richness of Cerambycidae s.s. accounts for the first 215 richest genera (see green dotted line), while the first 829 richest genera contain 75% of the total global richness of longhorn beetles (see red dotted line)
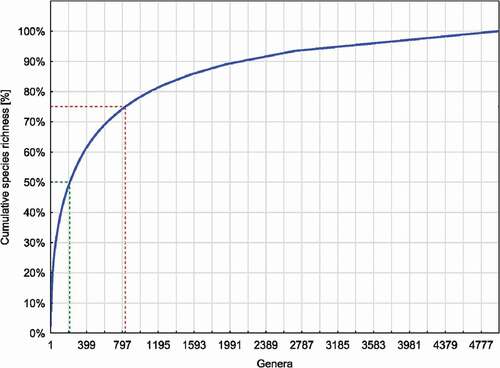
The rate of Cerambycidae description has changed significantly over time from the low and constant rate up to the 1830s, through the rapid increase that had begun in the 1850s, to the second acceleration in the 1940s (). In the twenty-first century (from 2000 up to 2018), the species description ratio was at an average of 257 newly described cerambycidae per year. The species description ratio also varied significantly, both among biogeographic realms and continents (). In the year 1900, as many as 55.28% of all currently described longhorn beetles of the Nearctic were already known, whereas in the case of the Oriental or Etiophian realms, the percentage of known species were still less than 20% during that same time. In the following decades, the step increase in the species description ratio can be found especially in the Oriental and the Neotropical realms, and a slightly lower ratio can be found in the Palearctic realm (). The rest of studied realms, including the Ethiopian, Australasian, Nearctic, and Madagascan were characterized by more flattened description curves. At the continental-level analysis, Asia and South America were found to be the two continents with the steepest species description curve in the 21st century ().
Figure 5. Discovery curves of Cerambycidae s.s. from 1758 up to 20th March 2019: (a) Discovery curves of Cerambycidae s.s. jointly and separately for each of the seven biogeographic realms (Newton Citation2003); (b) Discovery curves of Cerambycidae s.s. jointly and separately for each of the seven continents (Australia (mainland) and Oceania were separated); (c) Number of Cerambycidae s.s. species described in each of the seven biogeographic realms given in 10 years intervals; (d) Number of Cerambycidae s.s. species described in each of the seven continents given in 10 years intervals (Australia (mainland) and Oceania were separated). The last interval (1998–2018) has been increased by the first decade of 2019 (up to 20th March 2019)
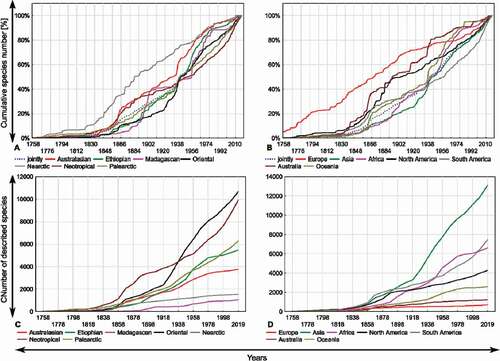
Geographic distribution
Most of the Cerambycidae taxonomic diversity was found to be concentrated in the Oriental and Neotropical realms (30.9%, 28.7%, respectively), however, the Palearctic, Ethiopian, and Australasian regions also contributed significantly to the global longhorn beetle diversity (18.2%, 15.8%, 10.9%, respectively). Only 4.5% of known cerambycid species occurred in the Nearctic, and 3.1% in the Madagascan realm. An analysis at the continental-level showed that Asia and South America, as well as Africa, were the most species–rich continents (37.9%, 21.6%, 19.2%, respectively). A lower contribution was found for North America (12.4%), Oceania (7.5%), Australia (3.5%) and Europe (2.0%).
The percentage contribution of each subfamily of Cerambycidae to the overall species diversity of this group has varied significantly among biogeographic realms ( – left) and continents ( – right). The subfamily Lamiinae was found to be a major contributor of the beta diversity of longhorn beetles in six out of seven analyzed realms, and its percentage contribution surpassed 50% in these regions ( – left). The only exception was the Nearctic, where the Lamiinae contribution (27.8%) was exceeded by Cerambycinae (50.2%) ( – left). Some similarities can be found between the Australasian and Ethiopian realms, where Lamiinae constituted around 70% (65.9%, 71.5% – Australasian and Ethiopian, respectively) and the second most abundant subfamily was Cerambycidae with about 30% of the contribution (30.1%, 24.5% – Australasian and Ethiopian, respectively), while other subfamilies did not exceed 5% in total ( – left). Another similarity can also be found between the Nearctic and Palearctic realms, where the Lepturinae contribution (14.9%, 14.7% – Nearctic and Palearcic, respectively) was markedly higher than in other realms ( – left). A unique pattern can be found in the Madagascan realm, where the endemic Apatophyseinae subfamily constituted as much as 24.5%, the contribution of the subfamily Prioninae (9.7%) was the highest among all realms, and at the same time the contribution of Cerambycinae was the lowest (10.8%) ( – left).
Figure 6. The percentage contribution of each of the nine subfamilies of Cerambycidae s.s to the overall species diversity of the family in each of the even biogeographic realms (left), and in each of the seven continents (right). Australia (mainland) and Oceania were separated
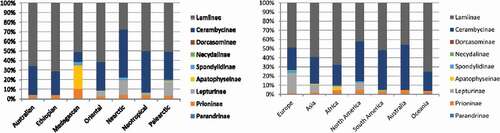
The clustering analysis based on the Jaccard Dissimilarity Index revealed three distinct branches representing three geographical assemblies of Cerambycidae (), including Pan-American, Palearctic-Oriental (with the Australian sub–branch) and Afrotropical. The vast majority (88.1%) of the Cerambycidae species occurred only in one biogeographic realm. Over 95% of cerambycid fauna of the Australasian, Ethiopian, Madagascan, and Neotropical realms were restricted to the only one biogeographic realm (97.3%, 97.0%, 96.6%, 95.6%, respectively). The higher overlap with the other realms was found in the case of the Palearctic – where only 42.7% of longhorn beetles were endemic, while the same index was 67.0% for the Oriental and 71.7% for the Nearctic realms. The analysis at the continental level showed that over 95% of the longhorn beetle fauna of Africa and Asia was restricted to the only one continent (97.5%, 95.9%, respectively), while other continents with a higher level of endemism were Australia, Oceania, and South America (94.8%, 94.7%, 90.8%, respectively). The same index was lower for Europe and North America (41.5%, 83.7%, respectively). Only 12.2% of longhorn species globally were distributed in at least two different realms. Widespread species that occurred in at least 3, but up to even 5 realms were rare and constituted 0.2% of all cerambycids (). The most widespread species (distributed in five biogeographic realms) were: Arhopalus rusticus (L., 1758), Callidiellum rufipenne (Motsch., 1862), Phoracantha recurva Newman, 1840, Ph. semipunctata (Fabr., 1775), Xystrocera globosa (Oliv., 1795) and Batocera rufomaculata (DeGeer, 1775). The same analysis at the genus level indicated that 79.2% of Cerambycidae genera are restricted to the only one biogeographic realm (). Eight genera occurred in more than six biogeographic realms, including: Callidium Fabr., 1775, Ceresium Newman, 1842, Xylotrechus Chevrolat, 1860, Stromatium Audinet–Serville, 1834, Xystrocera Audinet–Serville, 1834, Apomecyna Guerin–Meneville, 1835, Batocera Dejean, 1835, Monochamus Dejean, 1821. The most widespread genera (distributed in seven different biogeographic realms) were Callidium Fabr., Xylotrechus Chevrolat, and Monochamus Dejean.
Table IV. Occurrence of Cerambycidae s.s species and genera in biogeographic realms
Figure 7. Dendrograms based on the UPGMA clustering method for the seven biogeographic realms (a) and for the seven continents (b) (Australia (mainland) and Oceania were separated) based on the matrix of Jaccard dissimilarity (1– Jaccard index) conducted for longhorn beetles (Cerambycidae s.s.) at the genus level
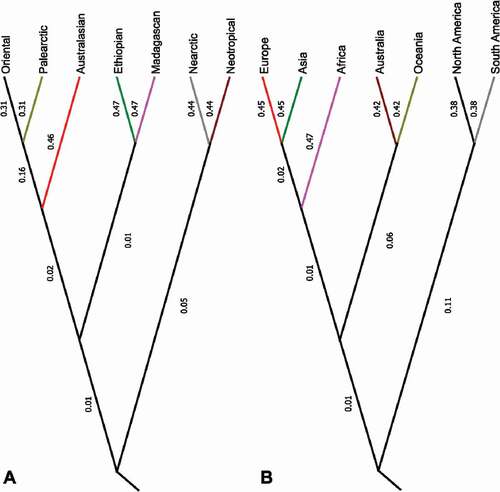
Discussion
Taxonomic richness
With the 34,490 extant species subdivided into 4,959 genera, (March 2019) the Cerambycidae constitute the largest family within the Chrysomeloidea Latreille superfamily (> 63,000 extant species worldwide (Ślipiński et al. Citation2011), and one of the largest families of beetles in existence. Although the obtained number of cerambycid species showed a good agreement with the other studies (Švácha & Lawrence Citation2014; Wang Citation2017) it should be treated with caution as the collected data is an estimation rather than the actual number of species, which is impossible to determine precisely due to numerous taxonomic uncertainties and the group complexity.
The quantitative contribution of other families to the overall taxonomic richness of the traditional “cerambycoid assemblage” (see Materials and Methods section) is rather marginal and does not surpass 1.5%. Most of the Cerambycidae taxonomic diversity is concentrated in two hyper–diverse subfamilies: Lamiinae and Cerambycinae which together cover 90.5% of all longhorn beetles. Although monospecific genera are predominant, the vast majority of the taxonomic diversity of longhorn beetles is concentrated in the large multi–species genera; only the first 829 richest genera encompass three quarters of the total number of Cerambycidae species. This resulted in a highly–asymmetrical, right–skewed frequency of distribution which is a common pattern also found in other groups of animals (Fisher et al. Citation1943; Preston Citation1948; Jetschke & Hubbell Citation2002; Barber-James et al. Citation2008; Pincheira-Donoso et al. Citation2013). These findings suggest that the prominent evolutionary proliferations leading to the formation of a large number of recently radiated species are rather rare events in the recent evolutionary history of Cerambycidae. On the other hand, a large number of monospecific genera might suggest the occurrence of relict lineages of formerly more diversified evolutionary branches, or alternatively, indicate young or even ongoing radiations (Barber-James et al. Citation2008).
Description ratio
We found that the species description ratio of longhorn beetles differed significantly among biogeographic realms and continents, and has also changed significantly throughout time. Dynamic development of the modern taxonomy in Europe contributed to the description of a significant number of European longhorn beetle species quite early in the eighteenth century (). The modern faunistic trends have reached North America relatively quickly and have initiated taxonomic studies on the Nearctic fauna of Cerambycidae. As a result, three-quarters of all currently known Nearctic species were described by the end of the 1950s. Nevertheless, due to political issues and limited study effort, some other areas like Africa remained almost completely unexplored until the end of the nineteenth century. Historical events have also affected the species description curve of Cerambycidae; for example, the number of newly discovered species dropped during the Second World War. Species description curves for some areas e.g. Europe or mainland Australia are currently relatively flat, suggesting they are approaching their asymptotes. This indicates that the vast majority of cerambycid fauna of these areas have been already described. On the other hand, steep curves that can be found for Asia or South America, strongly suggest that the significant number of Cerambycidae species from these areas have not yet been described.
Diversity drivers
The patterns of biodiversity have been driven by a complex interaction of ecological and evolutionary processes acting in the geological time frame. As an example of ecological processes shaping biological diversity, we can count e.g. environmental filtering (Paine et al. Citation2012; Lamanna et al. Citation2014) or specific interspecies interactions (both positive and negative) (Elith & Leathwick Citation2009; Urban Citation2011; Wiens Citation2011), while the most prominent evolutionary processes affecting biodiversity patterns are extinction and the radiation of species (Foote et al. Citation1999; Roelants et al. Citation2007; Simões et al. Citation2016). Although the main drivers of organismic diversity are well known, there is still an ongoing debate on the importance of particular ecological and evolutionary factors in the shaping of the diversity patterns of the particular group or organism. Given that Coleoptera (beetles) are the largest group of animals in the world, the understanding of the drivers of their diversification have been a crucial challenge for evolutionary biologists. Several different hypotheses were proposed to explain the extraordinary diversity of Coleoptera (including Cerambycidae) (Hunt et al. Citation2007). One of the most frequently discussed explanations highlights the key role of co–radiation of Chrysomelidae with the early angiosperms (Farrell Citation1998; Farrell & Sequeira Citation2004; Wang et al. Citation2013). Nevertheless, these mechanisms can only partially explain the diversity of cerambycids (Haddad & Mckenna Citation2016; Haddad et al. Citation2018). Numerous species of longhorn beetles are known to be highly polyphagous at the larva stage, and some species may feed on both gymnospermous as well as on angiospermous plants. Even species within one genus may exhibit high diversity in respect to the host plants which strongly suggest that there were multiple host switches between angiospermous and gymnospermous plants during the cerambycid evolution (Haddad et al. Citation2018). We found that many genera containing exclusive polyphagous species are species–rich and widely distributed, which highlights an adaptive role of host plasticity in Cerambycidae evolution.
Biogeographic patterns
We found that at the global scale, the cerambycid fauna are geographically clustered into three major assemblages, including Pan–American, Afrotropical, and Palearctic-Oriental assemblage (with the Australasian branch). Our results also highlighted the high endemism of the Australasian, Ethiopian, Madagascan and Neotropical realms, and the phylogenetic distinctiveness of Madagascan fauna of Cerambycidae; whereas high species turnover was found for the Palearctic, Oriental and Nearctic realms. Moreover, we found the vast majority of the species are distributed within only one biogeographic realm. Most of the species assigned to two realms were distributed at the transition zones, especially within the Nearctic–Neotropical and Palearctic–Oriental transition zones. A small number of genera with the Trans–Pacific disjunct distribution were found, suggesting that the vicariance events were rather rare in the evolution of Cerambycidae (Kim et al. Citation2018). Other factors that might have potentially shaped the large–scale distribution patterns of Cerambycidae are continental drift, especially the break–up of Gondwana and the split of Laurasia (Haddad et al. Citation2018), or climatic oscillations - in particular, glacial events (Hewitt Citation1996; Shoda et al. Citation2003; Vitali & Schmitt Citation2017; Goczał et al. Citation2020). Some similarities in the distribution patterns of the Cerambycidae subfamilies among realms representing relatively similar environmental conditions, e.g. a significant contribution of Lepturinae to the longhorn beetle fauna of the Northern Hemisphere (Nearctic and Palearctic) might suggest the significant influence of environmental filtering on the distribution patterns of cerambycids. Furthermore, it was shown that live species’ history traits, especially dispersal abilities, might have had a significant influence on the Cerambycidae fauna composition (Vitali & Schmitt Citation2017). Finally, it should be strongly emphasized that all of the longhorn beetle species that exhibit exceptionally wide geographic distribution (which can be found in six different biogeographic realms) were accidentally introduced outside their native range by humans, which might have a negative effect on the native Cerambycidae fauna (Dearborn et al. Citation2016), and might affect the evolutionary patterns of this group.
Further challenges
Although Cerambycidae constitutes one of the largest, most diverse, ecologically, and economically important group of beetles in the world, there are still numerous issues and controversy regarding both their higher– and lower–level taxonomy (Haddad & Mckenna Citation2016; Ślipiński & Escalona Citation2016; Wang Citation2017). Moreover, most of the taxa revisions and new species descriptions are based only on morphological characters, rather than a genetic approach. This results in a high number of synonymous species, and hinders the comparison of Cerambycidae assemblages. A significant number of longhorn beetles, especially newly described species, have a dramatically poorly studied distribution; some species are known for only one uncertain location, which does not allow for an analysis of longhorn beetle distribution patterns based on high-resolution data. Finally, due to the limited number of high-quality genetic data, the phylogeography of Cerambycidae is poorly studied, and the main drivers of their diversity are still unclear. We strongly suggest that more research involving a comprehensive, multigene approach is needed to resolve the urgent taxonomy issues of Cerambycidae, and to better the understanding of the drivers of cerambycid diversity.
Acknowledgements
We would like to express our gratitude to Anna Dudzik for her help in data acquisition. We also thank anonymous reviewers for valuable comments on earlier version of the manuscript.
Disclosure statement
No potential conflict of interest was reported by the authors.
Additional information
Funding
References
- Akbulut S, Stamps WT. 2012. Insect vectors of the pinewood nematode: A review of the biology and ecology of Monochamus species. Forest Pathology 42:89–99. DOI: 10.1111/j.1439-0329.2011.00733.x.
- Barber-James HM, Gattolliat JL, Sartori M, Hubbard MD. 2008. Global diversity of mayflies (Ephemeroptera, Insecta) in freshwater. Hydrobiologia 595:339–350. DOI: 10.1007/s10750-007-9028-y.
- Barnosky AD, Matzke N, Tomiya S, Wogan GOU, Swartz B, Quental TB, Marshall C, McGuire JL, Lindsey EL, Maguire KC, Mersey B, Ferrer EA. 2011. Has the Earth’s sixth mass extinction already arrived? Nature 471:51–57. DOI: 10.1038/nature09678.
- Bezark L. 2019. A photographic Catalog of the Cerambycidae of the World. Available: http://bezbycids.com/byciddb/wdefault.asp?w=n. Accessed Mar 2019 15.
- Bílý S, Mehl O. 1989. Longhorn Beetles-Coleoptera, Cerambycidae-Of Fennoscandia and Denmark. Fauna Entomologica Scandinavica 22:1–204.
- Bouchard P, Bousquet Y, Davies AE, Alonso-Zarazaga MA, Lawrence JF, Lyal CHC, Newton AF, Reid CAM, Schmitt M, Ślipiński SA, Smith ABT. 2011. Family-group names in Coleoptera (Insecta). Zookeys 88:1–972. DOI: 10.3897/zookeys.88.807.
- Carvalho JC, Cardoso P, Borges PAV, Schmera D, Podani J. 2013. Measuring fractions of beta diversity and their relationships to nestedness: A theoretical and empirical comparison of novel approaches. Oikos 122:825–834. DOI: 10.1111/j.1600-0706.2012.20980.x.
- Ceballos G, Ehrlich PR, Barnosky AD, García A, Pringle RM, Palmer TM. 2015. Accelerated modern human-induced species losses: Entering the sixth mass extinction. Science Advances 1(5):e1400253. DOI: 10.1126/sciadv.1400253.
- Danilevsky M. 2012. Additions and corrections to the new Catalogue of Palaearctic Cerambycidae (Coleoptera) edited by I. Löbl and A. Smetana, 2010. Part. III. Munis Entomology & Zoology 7(1):109–173.
- Danilevsky ML. 2014. Longicorn Beetles (Coleoptera, Cerambycoidea) of Russia and Adjacent Countries. Part 1. Moscow: Higher School Consulting. pp. 522.
- Dearborn KW, Heard SB, Sweeney J, Pureswaran DS. 2016. Displacement of Tetropium cinnamopterum (Coleoptera: Cerambycidae) by Its Invasive Congener Tetropium fuscum. Environmental Entomology 45:848–854. DOI: 10.1093/ee/nvw045.
- Elith J, Leathwick JR. 2009. Species distribution models: Ecological explanation and prediction across space and time. Annual Review of Ecology, Evolution, and Systematics 40:677–697. DOI: 10.1146/annurev.ecolsys.110308.120159.
- Farrell BD. 1998. “Inordinate fondness” explained: Why are there so many beetles? Science 281(5376):555–559. DOI: 10.1126/science.281.5376.555.
- Farrell BD, Sequeira AS. 2004. Evolutionary rates in the adaptive radiation of beetles on plants. Evolution 58(9):1984–2001. DOI: 10.1111/j.0014-3820.2004.tb00484.x.
- Fisher RA, Corbet AS, Williams CB. 1943. The relation between the number of species and the number of individuals in a random sample of an animal population. Journal of Animal Ecology 12(1):42–58. DOI: 10.2307/1411.
- Foote M, Hunter JP, Janis CM, Sepkoski JJ. 1999. Evolutionary and preservational constraints on origins of biologic groups: Divergence times of eutherian mammals. Science 283(5406):1310–1314. DOI: 10.1126/science.283.5406.1310.
- Gergócs V, Hufnagel L. 2015. Global pattern of oribatid mites (Acari: Oribatida) revealed by fractions of beta diversity and multivariate analysis. International Journal of Acarology 41:574–583. DOI: 10.1080/01647954.2015.1084044.
- Goczał J, Oleksa A, Rossa R, Chybicki I, Meyza K, Plewa R, Landvik M, Gobbi M, Hoch G, Tamutis V, Balalaikins M, Telnov D, Dascălu MM, Tofilski A. 2020. Climatic oscillations in Quaternary have shaped the co-evolutionary patterns between the Norway spruce and its host-associated herbivore. Scientific Reports 10(1):1–14. DOI: 10.1038/s41598-020-73272-0.
- Haack RA. 2006. Exotic bark- and wood-boring Coleoptera in the United States: Recent establishments and interceptions. Canadian Journal of Forest Research 36:269–288. DOI: 10.1139/x05-249.
- Haddad S, Mckenna DD. 2016. Phylogeny and evolution of the superfamily Chrysomeloidea (Coleoptera: Cucujiformia). Systematic Entomology 41(4):697–716. DOI: 10.1111/syen.12179.
- Haddad S, Shin S, Lemmon AR, Lemmon EM, Svacha P, Farrell B, Ślipiński A, Windsor D, Mckenna DD. 2018. Anchored hybrid enrichment provides new insights into the phylogeny and evolution of longhorned beetles (Cerambycidae). Systematic Entomology 43(1):68–89. DOI: 10.1111/syen.12257.
- Hewitt G. 1996. Some genetic consequences of ice ages, and their role in divergence and speciation. Biological Journal of the Linnean Society 58:247–276. DOI: 10.1111/j.1095-8312.1996.tb01434.x.
- Huang GQ, Huang JB, Liu YF. 2020. Review of the genus Hechinoschema Thomson, 1857 (Coleoptera: Cerambycidae: Lamiinae: Monochamini), with description of a new genus and two new species. Zootaxa 4768(4):517–537. DOI: 10.11646/zootaxa.4768.4.4.
- Hunt T, Bergsten J, Levkanicova Z, Papadopoulou A, John OS, Wild R, Hammond PM, Ahrens D, Balke M, Caterino MS, Gómez-Zurita J, Ribera I, Barraclough TG, Bocakova M, Bocak L, Vogler AP. 2007. A comprehensive phylogeny of beetles reveals the evolutionary origins of a superradiation. Science 318(5858):1913–1916. DOI: 10.1126/science.1146954.
- Jankowiak R, Rossa R. 2007. Filamentous fungi associated with Monochamus galloprovincialis and Acanthocinus aedilis (Coleoptera: Cerambycidae) in scots pine. Polish Botanical Journal 52(2):143–149.
- Jeppsson T, Forslund P. 2014. Species’ traits explain differences in Red list status and longterm population trends in longhorn beetles. Animal Conservation 17(4):332–341. DOI: 10.1111/acv.12099.
- Jetschke G, Hubbell SP. 2002. The unified neutral theory of biodiversity and biogeography. Monographs in population biology 32. Princeton: Princeton Univeristy Press. pp. 375.
- Kariyanna B, Muthugounder M, Vitali F, Kurzawa J. 2019. Checklist of Disteniidae and Vesperidae (Coleoptera) from India. Oriental Insects 53(4):470–482. DOI: 10.1080/00305316.2018.1531079.
- Kim S, de Medeiros BAS, Byun BK, Lee S, Kang JH, Lee B, Farrell BD. 2018. West meets East: How do rainforest beetles become circum-Pacific? Evolutionary origin of Callipogon relictus and allied species (Cerambycidae: Prioninae) in the New and Old Worlds. Molecular Phylogenetics and Evolution 125:163–176. DOI: 10.1016/j.ympev.2018.02.019.
- Kumar S, Stecher G, Li M, Knyaz C, Tamura K. 2018. MEGA X: Molecular evolutionary genetics analysis across computing platforms. Molecular Biology and Evolution 35(6):1547–1549. DOI: 10.1093/molbev/msy096.
- Lamanna C, Blonder B, Violle C, Kraft NJB, Sandel B, Šímová I, Donoghue JC, Svenning JC, McGill BJ, Boyle B, Buzzard V, Dolins S, Jørgensen PM, Marcuse-Kubitza A, Morueta-Holme N, Peet RK, Piel WH, Regetz J, Schildhauer M, Spencer N, Thiers B, Wiser SK, Enquist BJ. 2014. Functional trait space and the latitudinal diversity gradient. Proceedings of the National Academy of Sciences of the United States of America 111(38):13745–13750. DOI: 10.1073/pnas.1317722111.
- Lingafelter SW. 2015. The Prioninae (Coleoptera: Cerambycidae) of Hispaniola, with diagnoses, descriptions of new species, distribution records, and a key for identification. The Coleopterists Bulletin 69(3):353–388. DOI: 10.1649/0010-065X-69.3.353.
- Monné MA. 2005a. Catalogue of the Cerambycidae (Coleoptera) of the Neotropical Region. Part I. Subfamily Cerambycinae. Zootaxa 946(946):1–765. DOI: 10.11646/zootaxa.946.1.1.
- Monné MA. 2005b. Catalogue of the Cerambycidae (Coleoptera) of the Neotropical region. Part II. Subfamily Lamiinae. Zootaxa 1023(1023):1–739. DOI: 10.11646/zootaxa.1023.1.1.
- Monné MA. 2006. Catalogue of the Cerambycidae (Coleoptera) of the Neotropical Region. Part III. Subfamilies Parandrinae, Prioninae, Anoplodermatinae, Aseminae, Spondylidinae, Lepturinae, Oxypeltinae, and addenda to the Cerambycinae and Lamiinae. Zootaxa 1212(1):1–244. DOI: 10.11646/zootaxa.1212.1.1.
- Newton I. 2003. The speciation and biogeography of birds. 1st ed. London - San Diego: Academic Press. pp. 668.
- Paine CET, Norden N, Chave J, Forget PM, Fortunel C, Dexter KG, Baraloto C. 2012. Phylogenetic density dependence and environmental filtering predict seedling mortality in a tropical forest. Ecology Letters 15(1):34–41. DOI: 10.1111/j.1461-0248.2011.01705.x.
- Pincheira-Donoso D, Bauer AM, Meiri S, Uetz P. 2013. Global taxonomic diversity of living reptiles. PLoS ONE 8(3):e59741. DOI: 10.1371/journal.pone.0059741.
- Pirkl J. 2019. Prioninae of the world. Available: http://www.prioninae.eu. Accessed Mar 2019.
- Preston FW. 1948. The commonness, and rarity, of species. Ecology 29(3):254–283. DOI: 10.2307/1930989.
- Real R, Vargas JM. 1996. The probabilistic basis of Jaccard’s Index of similarity. Systematic Biology 45(3):380–385. DOI: 10.1093/sysbio/45.3.380.
- Roelants K, Gower DJ, Wilkinson M, Loader SP, Biju SD, Guillaume K, Moriau L, Bossuyt F. 2007. Global patterns of diversification in the history of modern amphibians. Proceedings of the National Academy of Sciences of the United States of America 104(3):887–892. DOI: 10.1073/pnas.0608378104.
- Roguet J. 2019. Lamiinae of World. Available: https://lamiinae.org. Accessed Mar 2019 20.
- Sama G. 2002. Atlas of the Cerambycidae of Europe and the Mediterranean Area. Vol. 1, Northern, Western, Central and Eastern Europe British Isles and Continental Europe From France (excl.Corsica) to Scandinavia and Urals. Zlín: Kabourek. pp. 173.
- Seibold S, Brandl R, Buse J, Hothorn T, Schmidl J, Thorn S, Müller J. 2015. Association of extinction risk of saproxylic beetles with ecological degradation of forests in Europe. Conservation Biology 29(2):382–390. DOI: 10.1111/cobi.12427.
- Shapiro SS, Wilk MB. 1965. An analysis of variance test for normality. Biometrika 52(3/4):591–611. DOI: 10.2307/2333709.
- Shoda E, Kubota K, Makihara H. 2003. Geographical structuring of mitochondrial DNA in Semanotus japonicus (Coleoptera: Cerambycidae). Applied Entomology and Zoology 38(3):339–345. DOI: 10.1303/aez.2003.339.
- Simões M, Breitkreuz L, Alvarado M, Baca S, Cooper JC, Heins L, Herzog K, Lieberman BS. 2016. The evolving theory of evolutionary radiations. Trends in Ecology & Evolution 31(1):27–34. DOI: 10.1016/j.tree.2015.10.007.
- Ślipiński A, Escalona H. 2016. Australian Longhorn Beetles (Coleoptera: Cerambycidae). Vol. 2, Subfamily Cerambycinae. Clayton: CSIRO Publishing. pp. 640. DOI: 10.1071/9781486304592.
- Ślipiński SA, Leschen RAB, Lawrence JF. 2011. Order Coleoptera Linnaeus, 1758. In: Zhang, Z-Q, editors. Animal biodiversity: An outline of higher-level classification and survey of taxonomic richness. Zootaxa 3148(1):203–208. DOI: 10.11646/zootaxa.3148.1.39.
- Spomer SM. 2014. The longhorn beetles (Coleoptera: Cerambycidae) of Nebraska, USA: New state records, a checklist of known species, and distribution maps. The Coleopterists Bulletin 68(2):297–315. DOI: 10.1649/0010-065X-68.2.297.
- StatSoft Inc. 2017. TIBCO Software Inc. (2017). Statistica (data analysis software system), version 13.
- Švácha P, Lawrence J. 2014. Chapter 2.4 Cerambycidae Latreille, 1802. In: Leschen RAB, Beutel RG, editors. Handbook of zoology: Arthropoda: Insecta: Coleoptera, Beetles. Vol. 3, Morphology and Systematics (Phytophaga). Berlin/Boston: Walter de Gruyter. pp. 16–177.
- Tavakilian G, Chevillotte H. 2019. Base de données Titan sur les Cerambycidés ou Longicornes. Available: http://titan.gbif.fr. Accessed Mar 2019 20.
- Urban MC. 2011. The evolution of species interactions across natural landscapes. Ecology Letters 14(7):723–732. DOI: 10.1111/j.1461-0248.2011.01632.x.
- Vitali F. 2019. Cerambycoidea. Available: https://www.cerambycoidea.com. Accessed Mar 2019 20.
- Vitali F, Schmitt T. 2017. Ecological patterns strongly impact the biogeography of western Palaearctic longhorn beetles (Coleoptera: Cerambycoidea). Organisms Diversity & Evolution 17:163–180. DOI: 10.1007/s13127-016-0290-6.
- Wallin H, Schroeder M, Kvamme T. 2013. A review of the European species of Monochamus Dejean, 1821 (Coleoptera, Cerambycidae) - with a description of the genitalia characters. Norvegian Journal of Entomology 60:11–38.
- Wang B, Zhang H, Jarzembowski EA. 2013. Early Cretaceous angiosperms and beetle evolution. Frontiers in Plant Science 4:360. DOI: 10.3389/fpls.2013.00360.
- Wang Q. 2017. Cerambycidae of the world: Biology and pest management. London-New York: CRC Press, Taylor & Francis Group. pp. 628. DOI: 10.1201/b21851.
- Wiens JJ. 2011. The niche, biogeography and species interactions. Philosophical Transactions of the Royal Society B Biological Sciences 366(1576):2336–2350. DOI: 10.1098/rstb.2011.0059.