Abstract
The jumping spider genus Cytaea is an iconic member of the Australo-Pacific region. The genus as recognized today is reportedly not monophyletic, most of the forty-two species described here in the 19th century lacking modern diagnoses and adequate illustrations. The genus clearly needs a throughout revision. To avoid future synonyms, species redescriptions based on integrative taxonomy are necessary. Several species of the genus Cytaea are studied here in terms of species taxonomy. Cytaea alburna Keyserling 1882, the type species, is re-described. We propose Cytaea severa (Thorell, 1881) and Cytaea barbatissima (Keyserling, 1881) are as junior synonyms of the new combination for Ascyltus asper (Karsch, 1878), Cytaea asper (Karsch, 1878) comb nov. as we find to belong to the genus as well. The integrative approach was used based on diagnostic morphological characters (presented as images and drawings), DNA barcodes and a barcode gap analysis which tested the species distinctness. Genitalic structures are redefined and discussed in terms of function. The conclusion for the 4 species were based on 22 specimens, 14 DNA barcodes, publication supplemented with 46 digital micrographs and 7 drawings.http://LSIDurn:lsid:zoobank.org:pub:001FB3A0-074E-4290-B2D8-AD246D629E93
Introduction
Spiders are one of the most successful groups of arthropods and an interesting model in biological studies (Mammola et al. Citation2017). So far, nearly 50 thousand species in 120 families have been described (WSC Citation2021), but recent estimates range from 76 to 170 thousand species (Agnarsson et al. Citation2013; Platnick & Raven Citation2013). A large part of species described in XIXth and early XXth centuries lack proper diagnoses, documentation, were placed in wrong genera, need verification and revision (Paquin & Dupérré Citation2009; Dimitrov & Hormiga Citation2010; Sudhin et al. Citation2016). Although studies of the type specimens are pivotal in revisional works, several hindering circumstances like the poor condition of the specimens, unknown depository, being destroyed or lost, could be managed (Arnedo Citation2003; Nentwig et al. Citation2020). Molecular markers also can help support species delimitation (Hebert et al. Citation2003). The DNA barcoding has variety of applications in spider research, while is apply for estimate country scale species diversity (Astrin et al. Citation2016; Coddington et al. Citation2016), identification species in tropical regions (Gaikwad et al. Citation2017; Tahir et al. Citation2019; Tyagi et al. Citation2019) in few cases problems arose (Ortiz et al. Citation2020). With over 6200 species and 646 genera (WSC Citation2021), salticids are the most diverse family of spiders. Still however, the actual numbers may be 2–3 times higher, especially in tropical regions (Żabka Citation1991). Almost half of the species have been described from single sex and 367 species still lack illustrations (Metzner Citation2021). The genus Cytaea Keyserling (Citation1882) well illustrates these issues. It includes 42 nominal species (WSC Citation2021) from the Australian and Oriental Regions (Żabka Citation1991; Patoleta & Trębicki Citation2015). Many species described over a century ago lack clear diagnoses and documentation (Trębicki et al. Citation2016). The phylogenetic studies on Salticidae family indicate that genus in its present composition is not monophyletic and has to be revised (Zhang & Maddison Citation2013, Citation2015; Maddison Citation2015). Some Cytaea species have already been studied by Prószyński (Citation1976, Citation1984), Davies and Żabka (Citation1989), Żabka (Citation1991), Berry et al. (Citation1998), Patoleta and Gardzińska (Citation2010), Prószyński and Deeleman-Reinhold (Citation2010, Citation2013), Cao et al. (Citation2016), Wang and Li (Citation2020); however, the status of type species, of the genus and its closest relatives remains unclear. Cytaea alburna Keyserling Citation1882 is the generic type. In 1991 Żabka synonymized it with C. severa (Thorell Citation1881), while Prószyński (Citation2017) reinstated the species. Here we revisit the problem based on type and new material for four nominal species: Cytaea alburna Keyserling Citation1882, Cytaea severa (Thorell Citation1881), Cytaea barbatissima (Keyserling Citation1881) and Ascyltus asper (Karsch Citation1878) - all being previously involved in the problem. The taxonomical decisions were supported by DNA barcoding analysis.
The goals of this study are as follows: (I) to redescribe the type species of the genus Cytaea and its relatives, using integrative methods, (II) to provide a new reliable documentation enabling species identification, (III) to propose new characters for the genus.
Material and methods
Morphological methods
The study was based on type and new specimens; the latter being collected during various biodiversity surveys in Australia and preserved in 75% or in 96% ethanol. The specimens used in taxonomic and phylogenetic studies belong to the following institutions: Zoologisches Institut und Zoologisches Museum, Universität Hamburg (ZMH), Museo Civico di Storia Naturale Giacomo Doria, Genoa (MCSN), Zoologisches Museum der Humboldt-Universität, Berlin (ZMB), Muséum National d’Histoire Naturelle, Paris (MNHN), Queensland Museum, Brisbane (QMB). Specimens were examined with Nikon SMZ800, SMZ1000 and Ci microscopes and photographed with Nikon D5100 digital camera. Pictures were digitally processed with HeliconFocus and Adobe Photoshop software. The drawings were made from digital photographs. Epigynes were cleared in 10% potassium hydroxide (KOH) and in methyl salicylate (C8H8O3). All measurements are given in millimetres. Photographs of live spiders and fresh specimens were provided by Robert Whyte, through Queensland Museum Brisbane, Australia. Terminology follows Zhang and Maddison (Citation2015). For further explanations see . Abbreviation used: CL: cephalothorax length, CW: cephalothorax width, CH: cephalothorax height, AL: abdomen length, AW: abdomen width, AH: abdomen height, EFL: eye field length, AEW: anterior eye row width, PEW: posterior eye row width, DAM: diameter of anterior median eye. The embolic base and course have been described using clock position.
Molecular methods
Specimens used in phylogenetic analysis are listed in . New sequence data were generated from 14 Cytaea specimens. Total genomic DNA was extracted from single leg of each specimen, using the DNeasy Blood and Tissue Kit (Qiagen GmbH, Hilden, Germany) according to manufacturer’s instructions. The COI gene fragment was amplified with bcdF01 and bcdR04 primers (Dabert et al. Citation2010). PCRs were conducted in 10 μL reaction volumes containing 5 μL of the Type-it Microsatellite PCR Kit (Qiagen), 0.5 μM of each primer and 4 μL of DNA template. Thermocycling profile was as follows: one cycle of 5 min at 95°C followed by 35 steps of 30 s each at 95°C, 60 s at 50°C, 1 min at 72°C, and with a final step of 5 min at 72°C.
Table I. List of specimens studied (F: female, M: male specimen)
After amplification, PCR reactions were diluted with 10 μL of MQ water and 5 μL were analysed by electrophoresis on 1% agarose gel. Samples containing visible bands were purified with exonuclease I and Fast alkaline phosphatase (Thermo Fisher Scientific, USA) and sequenced using the BigDye Terminator v3.1 kit and the ABI Prism 3130xl Genetic Analyzer (Applied Biosystems), following the manufacturer’s instructions. Sequence chromatograms were checked for accuracy in FinchTV 1.3.1 (Geospiza Inc.). Sequences were aligned using MUSCLE (Edgar Citation2004) with default parameters, performed in Geneious 10.2.3 (Biomatters Ltd.). Genetic distances were estimated using the Kimura 2-parameter model (K2P) (Kimura Citation1980), Bootstrap values calculated with 500 replicates (Felsenstein Citation1985) as implemented in MEGA X (Stecher et al. Citation2020). DNA barcoding-gap was calculated using ABGD method with K2P model and default settings (http://wwwabi.snv.jussieu.fr/public/abgd/) (Puillandre et al. Citation2012). All new sequences used in this work have been deposited in BOLD Systems (https://www.boldsystems.org/) and GenBank (https://www.ncbi.nlm.nih.gov/) (). In addition, comparative pictures of specimens preserved in ethanol are attached to sequences deposited in BOLD.
Results
The final alignment for species delimitation comprised 644 nucleotide positions (nps) for COI gene sequences representing 14 specimens (). The nucleotide sequences could be translated into amino acid sequences without any stop codons. In the dataset, 77 nps out of 644 were variable, and transition-to-transversion ratio (R) amounted to 1.47. Neighbour-Joining (NJ) analysis clustered Cytaea COI sequences into two maximally supported clades () grouping members of C. alburna and C. asper. Genetic distance between C. alburna and C. asper COI sequences amounted to 12.13%. Mean intraspecific distances were 0.91% (SD = 0.27%) and 0.84% (SD = 0.33) for C. alburna and C. asper, respectively. Using ABGD method, we found a 3–10% barcoding gap between the species of C. alburna and C. asper (), supporting species distinctiveness.
Figure 1. Neighbor-joining tree of the COI sequences representing Cytaea alburna and C. asper. Numbers near branches show bootstrap values
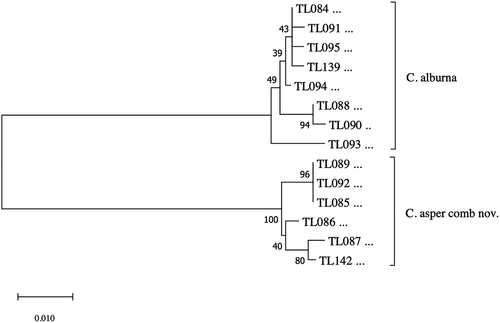
Figure 2. Results of Automatic Barcode Gap Discovery (ABGD) analysis for COI sequences. (a) Distribution of pairwise differences; (b) Ranked pairwise differences
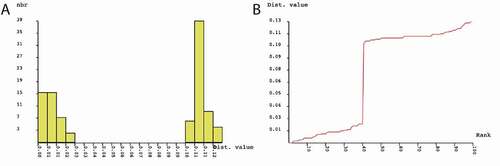
Closer examination of the type specimens of C. severa, C. barbatissima and Ascyltus asper has revealed their copulatory organs do not differ to put them into separate species; all are here transferred to Cytaea as Cytaea asper comb nov. The analysis () of C. alburna and Cytaea asper revealed that both are separate species, differing by general morphology () and details of gentilic structures ().
Taxonomy
Family Salticidae Blackwall 1841
Tribe Euophryini Simon 1901
Genus Cytaea Keyserling Citation1882
Cytaea Keyserling Citation1882: 1380–1381; Simon Citation1903: 810–817; Żabka Citation1991: 24; Berry et al. Citation1998: 150–151; Murphy & Murphy Citation2000: 349; Prószyński & Deeleman-Reinhold Citation2010: 162; Prószyński & Deeleman-Reinhold Citation2013: 117–118; Patoleta & Trębicki Citation2015: 556; Zhang i Maddison Citation2015: 31; Trębicki et al. Citation2016: 379.
Type species
Cytaea alburna Keyserling, Citation1882.
List of transferred species
Ascyltus asper (Karsch Citation1878) = Cytaea asper (Karsch Citation1878), comb nov.
Cytaea barbatissima (Keyserling Citation1881) = C. asper (Karsch Citation1878), comb nov.
Cytaea severa (Thorell Citation1881) = C. asper (Karsch Citation1878), comb nov.
Cytaea alburna (Keyserling Citation1882).
Figure 3. Cytaea alburna. (a–c) female habitus S103096; (d–e) male habitus S103083; (f) male habitus S103032; (g–i) male habitus S103087. Photos: Robert Whyte (QMB)
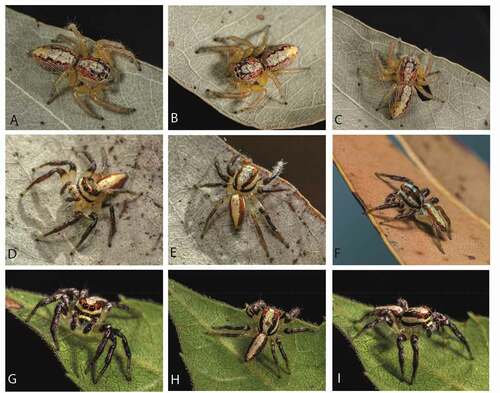
Figure 4. Cytaea alburna habitus. (a) Syntype ZMH dorsal view; (b) S103096 female dorsal view; (c) same, ventral view; (d) same, lateral view; (e) same, frontal view; (f) same, chelicerae dentation; (g) S103079 male dorsal view; (h) same, ventral view; (i) same, lateral view; (j) same, frontal view; (k) same close-up on chelicerae. Scale bars 1 mm
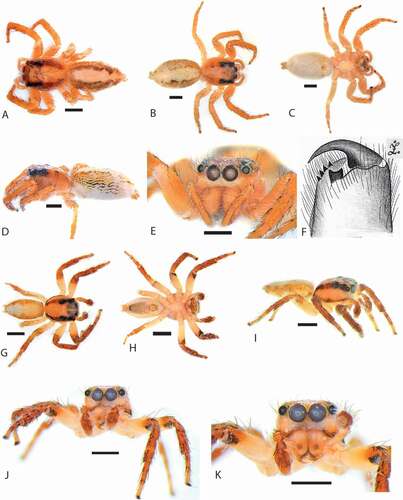
Figure 5. Cytaea alburna copulatory organs. (a) C. female S103096 epigyne ventral view; (b) same, dorsal view; (c) same, schematic drawings (lam. lateral atrial margin; mg. median guide; co. copulatory opening; cd. copulatory duct; s. spermatecae; ag I – ag II. accessory glands; fd. fertilization duct; (d) male S103079 pedipalp ventral view; (e) same, schematic drawing; (f) pedipalp lateral view; (g) same, schematic drawing (c. cymbium; e. embolus; ed. embolic disc; edi. embolic disc indentation; t. tegulum; sd. seminal duct; rta. retrolateral tibial apophysis). Scale bars 0.2 mm
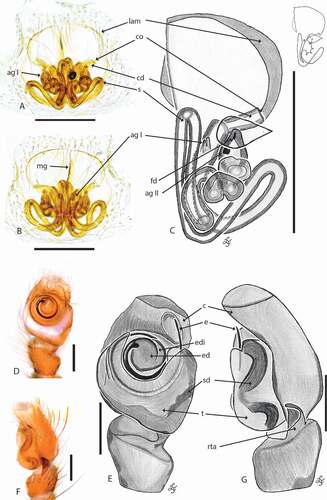
Cytaea alburna Keyserling Citation1882: 1383, Pl. 117, Figs 3–4; Prószyński Citation1984: Pl. 30; Davies & Żabka Citation1989: 220, Pl. 30; Żabka Citation1991: 25: synonymized with Cytaea severa (Thorell Citation1881), reinstated by Prószyński Citation2017: 74, Fig. 39C, Fig. 39D; Whyte & Anderson Citation2017: 238–240.
Material
Cytaea alburna Keyserling Citation1882, 3♀, syntypes, Peak Downs, Rockhampton, ZMH.
New material examined: 1♂, Queensland, Townsville, Alligator Creak, 11.11.2012, coll. I. R. Macaulay, QMB S103032; 1♂, Queensland, Mareeba, 28.04.2014, coll. R. Whyte, QMB S103079; 1♂, Queensland, Mareeba, 27.11.2013, coll. I. R. Macaulay QMB S103081; 1♀, Queensland, Glenmorgan, Myall Park Botanic Garden, 22.12.2012, coll. R. Whyte, QMB S103222. 1♂, Northern Territory, Katherine, 14.01.2013, coll. I. R. Macaulay, QMB S103083; 1♂, New South Wales, Kyogle, 28.10.2014, coll. R. Whyte, QMB S103087; 1♀, New South Wales, Grafton, 25.10.2014, coll. R. Whyte, QMB S103096.
Diagnosis
Embolus shorter than in C. fibula (Berland 1938): 2 circles vs. 3.5 circles, longer than in C. asper (Karsch Citation1878): 1.5 circle (). Embolus based on round disc, vs. longitudinal disc in C. nimbata (Thorell Citation1881). Unlike in C. laticeps (Thorell 1878), embolus is located on the ventral side of the bulb (in C. laticeps is perpendicular to the longitudinal axis of the bulb). Spermathecae in C. alburna () differ from C. nimbata (Thorell Citation1881) by presence of sclerotized lateral atrial margins on the epigynal windows and location of copulatory openings: posteriorly in C. alburna vs. anteriorly in C. nimbata (Thorell Citation1881).
Female S103096
Cephalothorax dark orange, eye surroundings black. Whole surface covered with brown numerous setae and white scales, the latter form a belt along the lower margin (). Eye field wider than long, its length 54% of CL. PME halfway between PLE and ALE. Fovea located between PLE (). Abdomen elongate, whitish, covered with sparse brown hairs and orange scales, the latter numerous on sides (). Spinnerets orange, not distinctive. Clypeus narrower (33%) than AME diameter, pale orange, covered with long, white scales ()). Chelicerae orange, massive, elongate, inclined downwards, promargin with four teeth, retromargin with a single bicuspidate tooth (). Endites and labium orange, with pale chewing margins. Sternum yellowish ()). Legs I orange, others lighter, all legs covered with numerous spines and setae (). Leg formula: I-IV-III-II.
Epigyne with two circular windows separated by narrow median septum () and with sclerotized lateral atrial margins. Copulatory openings located posteriorly and oriented laterally (). Copulatory ducts short. Spermathecae channel-like, long and twisted, with 2 accessory glands: one located near the copulatory duct entry and another close to the fertilization duct ().
Dimensions: CL 3.5, CW 2.65, CH 1.55, AL 4.75, AW 2.75, AH 2.5, EFL 1.9, AEW 2.05, PEW 2, DAM 0.6, clypeus 0.2, epigyne length 0.45, epigyne width 0.56, leg I: 8.4 (1 + 0.4 + 2 + 1.1 + 1.8 + 1.2 + 0.9); leg II: 7.65 (0.9 + 0.35 + 1.9 + 1.15 + 1.4 + 1.15 + 0.8); leg III: 8 (0.9 + 0.4 + 2.05 + 1.2 + 1.2 + 1.4 + 0.85); leg: IV: 8.2 (0.9 + 0.35 + 1.95 + 1.1 + 1.5 + 1.5 + 0.9).
Male S103079
Cephalothorax orange, eye surroundings dark. Whole surface covered with numerous setae and black scales, the latter form two longitudinal thoracic stripes (). White scales present on eye field and on sides (). Eye field wider than long, narrowing posteriorly ()), its length 54% of CL. Fovea short, located between PLE. Abdomen elongate, pale, with dark orange and brown scales on sides, covered with dark brown setae (). Spinnerets orange. Clypeus light orange, narrower (44%) than AME diameter (), covered with long whitish scales. Chelicerae vertical, elongate, orange with black spots and transverse stripes of white scales at the base ()). Promargin with three teeth, retromargin with a single bicuspidate tooth. Endites and labium slender, not distinctive, orange, with lighter chewing margins. Sternum longer than wide, pale ()). Venter dark brown, pale on sides. All legs covered by numerous spines and setae (). Proximal parts of tibiae pale, the rest of legs pale covered with red shimmering scales. Leg formula: I-III-II-IV.
Palpal organ covered with red, metallic scales and setae (). RTA of medium length, wider at the base, with an apical hook, with distal notch (). Embolus originated at 11nd hour, based on a round disc with almost circa 2 loops ().
Dimensions: CL 2.3, CW 1.7; CH 1.05; AL 2.5; AW 1.3; AH 0.9; EFL 1.1; AEW 1.4; PEW 1.35; DAM 0.45; Clypeus 0.2, leg I: 5.4 (0.5 + 0.2 + 1.35 + 0.65 + 1.15 + 0.9 + 0.65), leg II: 5.15 (0.55 + 0.2 + 1.4 + 0.55 + 1.05 + 0.75 + 0.65), leg III: 5.35 (0.55 + 0.2 + 1.4 + 0.6 + 1 + 0.95 + 0.65), leg IV: 5 (0.6 + 0.2 + 1.45 + 0.45 + 0.9 + 0.8 + 0.6).
Cytaea asper Karsch Citation1878 comb nov..
Attus asper Karsch Citation1878: 24; Euryattus senex Simon Citation1885: 90; Zenodorus asper Żabka Citation1988: 476, Figs 151–157; Ascyltus asper Prószyński Citation2017: 73–74, Figs 1B: D8+E8+G8+H8, syn. nov.; Hasarius barbatissimus Keyserling Citation1881: 1272, Pl. 109, Figs 1–2; Cytaea barbatissima Keyserling Citation1883: 1477; Servaea barbatissima: Simon Citation1903: 819, Figs 965; Cytaea barbatissima: Żabka Citation1991: 24, syn. nov.; Plexippus severus Thorell Citation1881: 596; Cytaea severa Żabka Citation1991: 25; Prószyński Citation2017: Figs 39C, Figs 39D, syn. nov.
Material
Plexippus severus Thorell Citation1881 2♂♂, Somerset (Cape York), 1875, L. M. D’Albertis (MCSN). Hasarius barbatissima (Keyserling), 2♀♀, Syntypes (ZMH). Attus asper Karsch, 1 male Type, N. S. Wales, Daemel”, ZMB 1684.
New material examined: 1♀ Australian Capital Territory, Canberra, 16.12.2012, coll. I. R. Macaulay, (QMB S103029); 1♂ Queensland, Townsville, R. Whyte, (QMB S103076); 1♂, Queensland, Cooktown, 9.03.2010, I. R. Macaulay, (QMB S103084); 1♂, Queensland, Mareeba, 6.03.2012, R. Whyte, (QMB S103080); 1♂, Queensland, Tree Creek, 29.10.12, I. R. Macaulay, (QMB S103049); 1♂, New South Wales, Wollomombi Falls, 1.11.2012, I. R. Macaulay, (QMB S103045).
Diagnosis
C. asper is grater in size than C. alburna, covered with metallic shimmering scales and is dull in colour () vs. red, in alburna (). Also, the first pair of legs longer in C. severa. Palpal tibia twice longer than in C. alburna, embolus shorter by 0.5 loop length, spermatecae differ by course of ducts ().
Female S103029
Cephalothorax dark brown, eye surroundings black. Whole surface covered with numerous setae and white scales, the later more numerous along the central part and on sides (). Eye field wider than long, its length 54% of CL. PME halfway between PLE and ALE. Fovea located between PLE (). Abdomen elongate, dark brown, covered with brown setae and white scales (. Spinnerets brown. Clypeus brown, covered with long, white scales, narrower (36%) than AME diameter. Chelicerae brown, elongate, inclined downward (). Promargin with three teeth, retromargin with bicuspidate tooth. Endites and labium dark brown, with pale chewing margins, sternum brown (). Ventral abdomen dark brown. Legs brown, covered with numerous spines and setae (). Leg formula: I-III-II-IV.
Epigyne with two circular windows separated by narrow median septum () and sclerotized lateral atrial margins. Copulatory openings located posteriorly and oriented laterally (). Copulatory ducts short. Spermathecae long and twisted, channel-like, with two accessory glands; one located near the copulatory duct, second close to fertilization duct ().
Dimensions: CL 2.85; CW 2.55; CH 1.9; AL 4; AW 2.6; AH 2; EFL 1.45; AEW 2.1; PEW 2.25; DAM 0.55; Clypeus 0.2. Leg I: 7.95 (0.8 + 0.55 + 2 + 1 + 1,5 + 1.2 + 0.9); leg II: 7.35 (0.7 + 0.35 + 1.95 + 1.15 + 1.4 + 1.1 + 0.7); leg III: 7.55 (0.7 + 0.5 + 2.05 + 0.9 + 1.2 + 1.4 + 0.8); leg: IV: 7.05 (0.9 + 0.5 + 2.05 + 0.7 + 1.25 + 0.85 + 0.8). Epigyne length 0.5; width 0.88.
Male S103076
Cephalothorax dark brown, eye surroundings black. Whole surface covered with numerous setae and white scales, the latter numerous along central part and sides (). Eye field wider than long, its length 54% of CL. PME halfway between PLE and ALE. Fovea located between PLE (). Abdomen elongate, dark brown, covered with brown setae and white scales (). Spinnerets brown. Clypeus brown, covered with long, white scales, narrower (30%) than AME diameter. Chelicerae brown, elongate, inclined downwards (). Promargin with three teeth, retromargin with bicuspidate tooth ()). Endites and labium dark brown, with pale chewing margins, sternum brown ()). Ventral abdomen brownish. Legs brown covered by numerous spines and setae (). Leg formula: II-I-III- IV. Pedipalps brownish, covered with numerous white setae (). Palpal femur pale, RTA of medium length, wider at the base, hooked apically (). Embolus set on a rounded embolic disc, starts at the 7nd hour and makes 1,5 loop ().
Dimensions: CL 3.1; CW 2.6; CH 1.7; AL 3.55; AW 1.95; AH 1.7; EFL 1.85; AEW 2.3; PEW 2.25; DAM 0.65; Clypeus 0.2. Leg I: 7.7 (0.95 + 0.55 + 1.75 + 1.05 + 1.4 + 1.25 + 0.75); leg II: 10.05 (0.85 + 0.5 + 2.25 + 1.45 + 2.45 + 2 + 0.55); leg III: 7.4 (0.75 + 0.6 + 1.65 + 0.9 + 1.4 + 1.35 + 0.75); leg: IV: 7.35 (0.8 + 0.55 + 1.9 + 0.9 + 1.2 + 1.25 + 0.75).
Figure 6. Cytaea asper. (a) female habitus S103029; (b–c) male habitus S103084; (d–e) male habitus S103080; (f) male habitus S103049. Photos: Robert Whyte (QMB)
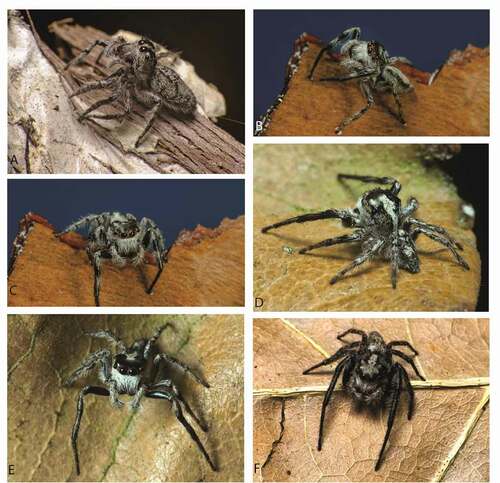
Figure 7. Cytaea asper comb nov. (a) female Syntype ZMH of Hasarius barbatissima dorsal view; (b) S103029 dorsal view; (c) same, ventral view; (d) same, lateral view; (e) same, frontal view; (f) same, epigyne; (g) male Syntype MCSN of Plexippus severus dorsal view; (h) male Syntype of Euryattus senex MNHN dorsal view; (i) male S103076 dorsal view; (j) same, ventral view; (k) same lateral view; (l) same, frontal view; (m) same chelicerae. Scale bars 1 mm
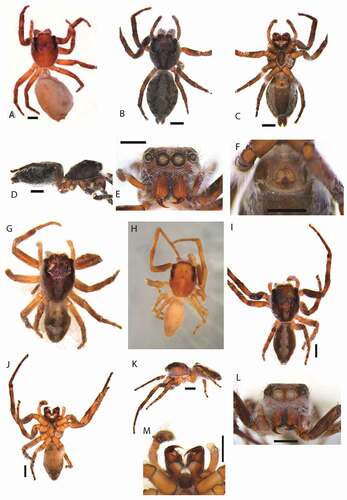
Figure 8. Cytaea asper comb nov. (a) female S103029 epigyne ventral view; (b) same, dorsal view; (c) same, schematic drawings (lam. lateral atrial margin; mg. median guide; co. copulatory opening; cd. copulatory duct; s. spermatecae; ag I – ag II. accessory glands; fd. fertilization duct); (d) male S103076 pedipalp ventral view; (e) same, schematic drawing; (f) pedipalp lateral view; (g) same, schematic drawing (c. cymbium; e. embolus; ed. embolic disc; edi. embolic disc indentation; t. tegulum; sd. seminal duct; rta. retrolateral tibial apophysis). Scale bars 0.2 mm
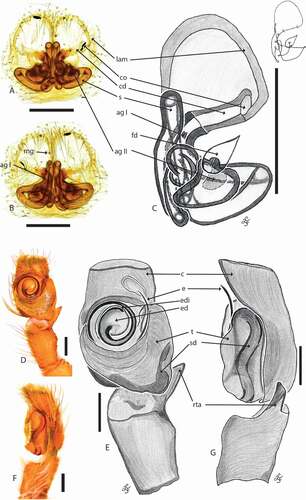
Discussion
The nominal type species of the genus Cytaea has been redescribed and 8 COI sequences have been obtained for molecular characterization of the species. Integrated morphological and molecular data allowed us to distinguish several species of the genus Cytaea and to propose 3 synonyms. Cytaea alburna, type species of the genus is well separated by morphology and DNA barcodes. Ascyltus asper, Cytaea severa and C. barbatissima were transferred and synonymized as Cytaea asper comb nov. Diagnostic illustration and DNA barcoding data proved species distinctiveness and clarified that Cytaea alburna is not the junior synonym of Cytaea severa (Żabka Citation1991; Prószyński Citation2017) – here proposed as Cytaea asper comb nov. Additionally, genitalic structures of studied species were redefined. To delimitate the boundaries between animal species, Hebert et al. (Citation2003) proposed a cytochrome c oxidase subunit 1 (COI) segment of approximately 650 nucleotides and the COI gene fragment has proven to be a good and most widely used marker for animal species delimitation (Hebert et al. Citation2003; Ratnasingham and Hebert Citation2007; DeSalle and Goldstein Citation2019), including spiders (Barrett & Hebert Citation2005). To establish the boundaries between species, for most taxa, the genetic discrepancy of 3% proved sufficient (Sbordoni Citation2010). However, due to different rates of evolution, the value may differ between groups of animals and even closely related species. To support species redescription in Salticidae, DNA barcoding method was used by Yamasaki et al. (Citation2018). The 28S gene was additionally sequenced to support the COI results (Vink et al. Citation2011; Macías-Hernández et al. Citation2020). The gene 16S was used to designate the species (Rezác et al. Citation2008; Marusik et al. Citation2018), while COI was also used in generic revisions (Richardson & Gunter Citation2012; Pekar et al. Citation2017). In our study, we applied DNA barcoding for studied species delimitation. Result of Neighbor-joining and ABGD analysis support distinctiveness of C. alburna and C. asper. COI DNA barcoding revealed that the mean nearest-neighbour distance based on species was 10 times higher than the mean intraspecific divergence in Canadian spiders (7.85% vs. 0.78%), and 7 times higher in Canadian Salticid spiders (7.57% vs. 1.18%) (Blagoev et al. Citation2016). Other studies on various arthropod taxa including spiders (Hebert et al. Citation2003; Barrett & Hebert Citation2005; Robinson et al. Citation2009) suggested that the interspecific divergence values of COI are usually greater than 2–3%, or the intraspecific divergence values of COI are usually less than 2–3%. Applying these thresholds showed that the distinctiveness of Cytaea alburna and C. asper comb nov. is strongly supported, it has to remembered though that some authors considered the DNA barcoding gap analyze as not conclusive (Puillandre et al. Citation2012) and suggest using morphological, distributional, environmental, microhabitat and behavioral data. In this research we analyze all available data, including DNA and morphology.
We redefined morphology of genitalic structures in studied species. Long channel-like spermathecae in Cytaea have been so far considered copulatory ducts (Zhang & Maddison Citation2015; Trębicki et al. Citation2016). This may be important defining the genus as a monophyletic group. Both Cytaea alburna and C. asper comb nov. have two spermathecal accessory glands, previously only the one nearby copulatory opening was known in those species. We discovered second accessory gland nearby fertilization duct. Accessory glands located in spider epigyne play a nutritive and protective function (desiccation and pathogens) for sperm cells stored in (Michalik & Uhl Citation2005). Female secretory products also have been suggested to control sperm activation. The accessory glands in Cytaea located nearby fertilization ducts may play similar role. Indentation at the embolus base correspond with anterior lateral atrium in epigyne window are probably part of lock and key mechanism – well known in many arthropods and plays a role of effective reproductive barrier (Masly Citation2012). All those aspects suggest need for future study on biology of C. alburna, C. asper comb nov. as well as on other species in the genus.
Geolocation information
Australia.
Acknowledgements
We thank for providing specimens for this study to Drs Danilo Harms and Nadine Dupérré (ZMH), Christine Rollard (MNHN), Robert Whyte and Wendy Hebron (QMB). Joanna Gardzińska for provision data for type specimens deposed in Museo Civico di Storia Naturale Giacomo Doria, Genoa (MCSN). The manuscript benefitted much from the critical remarks of two anonymous referees.
Disclosure statement
No potential conflict of interest was reported by the author(s).
Additional information
Funding
References
- Agnarsson I, Coddington JA, Kuntner M. 2013. Systematics: Progress in the study of spider diversity and evolution. In: Penney D, editor. Spider research in the 21st century: Trends and perspectives. Siri Scientific Press. pp. 58–111.
- Arnedo MA. 2003. Lost and found: Rediscovery of type material of some endemic species of the spider genus Dysdera (Araneae, Dysderidae) from the Canary Islands, and its nomenclatural and taxonomic implications. Revista Ibérica de Aracnología 7, 30-VI-2003 Sección: Artículos y Notas:141–148
- Astrin J, Höfer H, Spelda J, Holstein J, Bayer S, Hendrich L, et al. 2016. Towards a DNA Barcode reference database for spiders and harvestmen of Germany. PLoS ONE 11(9):e0162624. DOI: 10.1371/journal.pone.0162624.
- Barrett R, Hebert P. 2005. Identifying spiders through. DNA barcodes. Canadian Journal of Zoology 83:481–491. DOI: 10.1139/z05-024.
- Berry JW, Beatty JA, Prószyński J. 1998. Salticidae of the Pacific islands. III. Distribution of seven genera, with descriptions of nineteen new species and two new genera. Journal of Arachnology 26:149–189.
- Blagoev GA, deWaard JR, Ratnasingham S, deWaard L, Lu L, Robertson J, Telfer AC, Hebert DN. 2016. Untangling taxonomy: A DNA barcode reference library for Canadian spiders. Molecular Ecology Resources 16:325–341. DOI: 10.1111/1755-0998.12444.
- Cao X, Li S, Żabka M. 2016. The jumping spiders from Xishuangbanna, Yunnan, China (Araneae, Salticidae). ZooKeys 630:43–104. DOI: 10.3897/zookeys.630.8466.
- Coddington JA, Agnarsson I, Cheng R, Čandek K, Driskell A, Frick H, Gregorič M, Kostanjšek R, Kropf C, Kweskin M, Lokovšek T, Pipan M, Vidergar N, Kuntner M. 2016. DNA barcode data accurately assign higher spider taxa. PeerJ 4:e2201. DOI: 10.7717/peerj.2201.
- Dabert M, Witalinski W, Kazmierski A, Olszanowski Z, Dabert J. 2010. Molecular phylogeny of acariform mites (Acari, Arachnida): Strong conflict between phylogenetic signal and long-branch attraction artifacts. Molecular Phylogenetics and Evolution 56(1):222–241. DOI: 10.1016/j.ympev.2009.12.020.
- Davies TV, Żabka M. 1989. Illustrated keys to the genera of jumping spiders (Araneae: Salticidae) in Australia. Memoirs of the Queensland Museum 27:189–266.
- DeSalle R, Goldstein P. 2019. Review and interpretation of trends in DNA barcoding. Frontiers in Ecology and Evolution 7:302. DOI: 10.3389/fevo.2019.00302.
- Dimitrov D, Hormiga G. 2010. Mr. Darwin’s mysterious spider: On the type species of the genus Leucauge White, 1841 (Tetragnathidae, Araneae). Zootaxa 2396:19–36. DOI: 10.11646/zootaxa.2396.1.2.
- Edgar RC. 2004. MUSCLE: Multiple sequence alignment with high accuracy and high throughput. Nucleic Acids Research 32(5):1792–1797. DOI: 10.1093/nar/gkh340.
- Felsenstein J. 1985. Confidence limits on phylogenies: An approach using the bootstrap. Evolution 39(4):783–791. DOI: 10.1111/j.1558-5646.1985.tb00420.x.
- Gaikwad S, Warudkar A, Shouche Y. 2017. Efficacy of DNA barcoding for the species identification of spiders from Western Ghats of India. Mitochondrial DNA Part A: DNA Mapping, Sequencing, and Analysis 28(5):638–644. DOI: 10.3109/24701394.2016.1166219.
- Hebert P, Ratnasingham S, DeWaard J. 2003. Barcoding animal life: Cytochrome c oxidase subunit 1 divergences among closely related species. Proceedings of the Royal Society of London. Series B: Biological Sciences 270(Suppl.):S96–S99. DOI: 10.1098/rsbl.2003.0025.
- Karsch F. 1878. Diagnoses Attoidarum aliquot novarum Novae Hollandiae collectionis Musei Zoologici Berolinensis. Mittheilungen des Münchener Entomologischen Vereins 2:22–32.
- Keyserling E. 1881. Die Arachniden Australiens. Nürnberg 1:1272–1324.
- Keyserling E. 1882. Die Arachniden Australiens. Nürnberg 1:1325–1420.
- Keyserling E. 1883. Die Arachniden Australiens. Nürnberg 1:1421–1489.
- Kimura M. 1980. A simple method for estimating evolutionary rate of base substitutions through comparative studies of nucleotide sequences. Journal of Molecular Evolution 16:111–120. DOI: 10.1007/BF01731581.
- Macías-Hernández N, Domènech M, Cardoso P, Emerson BC, Borges PAV, Lozano-Fernandez J, Paulo OS, Vieira A, Enguídanos A, Rigal F, Amorim IR, Arnedo MA. 2020. Building a robust, densely-sampled spider tree of life for ecosystem research. Diversity 12:288. DOI: 10.3390/d12080288.
- Maddison W. 2015. A phylogenetic classification of jumping spiders (Araneae: Salticidae). Journal of Arachnology 43:231–292. DOI: 10.1636/arac-43-03-231-292.
- Mammola S, Michalik P, Hebets EA, Isaia M. 2017. Record breaking achievements by spiders and the scientists who study them. PeerJ 5:e3972. DOI: 10.7717/peerj.3972.
- Marusik Y, Fomichev A, Vahtera V. 2018. A new species of Chalcoscirtus (Araneae: Salticidae) from Altai, South Siberia, Russia. Entomologica Fennica 29:39–48. DOI: 10.33338/ef.70079.
- Masly JP. 2012. 170 years of “Lock-and-Key”: Genital morphology and reproductive isolation. International Journal of Evolutionary Biology, Article ID 247352, 10 p. DOI: 10.1155/2012/247352.
- Metzner H. 2021. Jumping spiders (Arachnida: Araneae: Salticidae) of the world. Available: https://www.jumping-spiders.com. Accessed Feb 2021 1.
- Michalik P, Uhl G. 2005. The male genital system of the cellar spider Pholcus phalangioides (Fuesslin, 1775) (Pholcidae, Araneae): Development of spermatozoa and seminal secretion. Frontiers in Zoology 2:12. DOI: 10.1186/1742-9994-2-12.
- Murphy F, Murphy J. 2000. An introduction to the spiders of South East Asia. Kuala Lumpur: Malaysian Nature Society. 624 pp.
- Nentwig W, Blick T, Gloor D, Jäger P, Kropf C. 2020. How to deal with destroyed type material? The case of Embrik Strand (Arachnida: Araneae). Arachnologische Mitteilungen/Arachnology Letters 59:22–29. DOI: 10.30963/aramit5904.
- Ortiz D, Pekár S, Bilat J, Alvarez N. 2020. Poor performance of DNA barcoding and the impact of RAD loci filtering on the species delimitation of an Iberian ant-eating spider. Molecular Phylogenetics and Evolution 154:106997. DOI: 10.1016/j.ympev.2020.106997.
- Paquin P, Dupérré N. 2009. A first step towards the revision of Cicurina: Redescription of type specimens of 60 troglobitic species of the subgenus Cicurella (Araneae: Dictynidae), and a first visual assessment of their distribution. Zootaxa 2002:1–67. DOI: 10.11646/zootaxa.2002.1.1.
- Patoleta B, Gardzińska J. 2010. Description of a new species of Cytaea Keyserling 1882 from Fiji (Araneae: Salticidae). Genus 21(4):631–635.
- Patoleta B, Trębicki Ł. 2015. Redescription of some poorly known species of Cytaea Keyserling, 1882 (Araneae: Salticidae), with new synonymies. Zootaxa 3949(4):555–566. DOI: 10.11646/zootaxa.3949.4.5.
- Pekar S, Petrakova L, Corcobado G, Whyte R. 2017. Revision of eastern Australian ant-mimicking spiders of the genus Myrmarachne (Araneae, Salticidae) reveals a complex of species and forms. Zoological Journal of the Linnean Society 179(3):642–676.
- Platnick NI, Raven RJ. 2013. Spider systematics: Past and future. Zootaxa 3683(5):595–600. DOI: 10.11646/zootaxa.3683.5.8.
- Prószyński J. 1976. Studium systematyczno-zoogeograficzne nad rodziną Salticidae (Aranei) Regionów Palearktycznego i Nearktycznego. Wyzsza Szkola Pedagogiczna Siedlcach 6:1–260.
- Prószyński J. 1984. Atlas rysunków diagnostycznych mniej znanych Salticidae (Araneae). Wyzsza Szkola Rolniczo- Pedagogiczna w Siedlcach 2:1–177.
- Prószyński J. 2017. Pragmatic classification of the world’s Salticidae (Araneae). Ecologica Montenegrina 12:1–133. DOI: 10.37828/em.2017.12.1.
- Prószyński J, Deeleman-Reinhold CL. 2010. Description of some Salticidae (Araneae) from the Malay Archipelago. I. Salticidae of the Lesser Sunda Islands, with comments on related species. Arthropoda Selecta 19(3):153–188. DOI: 10.15298/arthsel.19.3.05.
- Prószyński J, Deeleman-Reinhold CL. 2013. Description of some Salticidae (Aranei) from the Malay Archipelago. III. Salticidae of Borneo, with comments on adjacent territories. Arthropoda Selecta 22(2):113–144. DOI: 10.15298/arthsel.22.2.02.
- Puillandre N, Lambert A, Brouillet S, Achaz G. 2012. ABGD, Automatic Barcode Gap Discovery for primary species delimitation. Mol Ecol. Apr;21(8):1864–77. DOI:10.1111/j.1365-294X.2011.05239.x.
- Ratnasingham S, Hebert P. 2007. BOLD: The Barcode of Life Data System. Molecular Ecology Notes 7:355–364. DOI: 10.1111/j.1471-8286.2007.01678.x.
- Rezác M, Pekár S, Johannesen J. 2008. Taxonomic review and phylogenetic analysis of central European Eresus species (Araneae: Eresidae). Zoologica Scripta 37:263–287. DOI: 10.1111/j.1463-6409.2008.00328.x.
- Richardson B, Gunter N. 2012. Revision of Australian jumping spider genus Servaea Simon 1887 (Araneae: Salticidae) including use of DNA sequence data and predicted distributions. Zootaxa 3350:1–33. DOI: 10.11646/zootaxa.3350.1.1.
- Robinson E, Blagoev G, Hebert P, Adamowicz S. 2009. Prospects for using DNA barcoding to identify spiders in species-rich genera. ZooKeys 16:27–46. DOI: 10.3897/zookeys.16.239.
- Sbordoni V. 2010. Strength and Limitations of DNA Barcode under the Multidimensional Species Perspective. In: Nimis PL, Lebbe RV, editors. “Tools for Identifying Biodiversity: Progress and Problems. Proceedings of the International Congress, Paris, September 20–22, 2010”, Trieste: EUT Edizioni Università di Trieste. pp. 275–280.
- Simon E. 1885. Matériaux pour servir à la faune arachnologique de la Nouvelle Calédonie. Annales de la Société Entomologique de Belgique 29(C.R.):87–92.
- Simon E. 1903. Histoire naturelle des araignées. Deuxième édition, tome second. Paris: Roret. pp. 669–1080. DOI:10.5962/bhl.title.51973.
- Stecher G, Tamura K, Kumar S. 2020. Molecular Evolutionary Genetics Analysis (MEGA) for macOS. Molecular Biology and Evolution 37:1237–1239. DOI: 10.1093/molbev/msz312.
- Sudhin PP, Nafin KS, Simmons Z, Sudhikumar AV. 2016. On the type species of the genus Aetius O. Pickard-Cambridge, 1896: The first description of male with notes on cymbial notch and mating plug (Araneae: Corinnidae: Castianeirinae). Zootaxa 4154(4):489–500. DOI: 10.11646/zootaxa.4154.4.9.
- Tahir MH, Summer M, Mehmood S, Ashraf S, Naseem S. 2019. DNA barcoding of spiders from agricultural fields. Mitochondrial DNA Part B 4(2):4144–4151. DOI: 10.1080/23802359.2019.1693283.
- Thorell T. 1881. Studi sui Ragni Malesi e Papuani. III. Ragni dell’Austro Malesia e del Capo York, conservati nel Museo civico di storia naturale di Genova. Annali del Museo Civico di Storia Naturale di Genova 17:1–727.
- Trębicki Ł, Patoleta B, Gardzińska J. 2016. Redescription of four species of Cytaea Keyserling, 1882 (Araneae: Salticidae). Zootaxa 4189(2):378–386. DOI: 10.11646/zootaxa.4189.2.11.
- Tyagi K, Kumar V, Kundu S, et al. 2019. Identification of Indian Spiders through DNA barcoding: Cryptic species and species complex. Scientific Reports 9:14033. DOI: 10.1038/s41598-019-50510-8.
- Vink C, Dupérré N, McQuillan B. 2011. The black-headed jumping spider, Trite planiceps Simon, 1899 (Araneae: Salticidae): Redescription including cytochrome c oxidase subunit 1 and paralogous 28S sequences. New Zealand Journal of Zoology 38(4):317–331. DOI: 10.1080/03014223.2011.613939.
- Wang C, Li SQ. 2020. On eight species of jumping spiders from Xishuangbanna, Yunnan, China (Araneae, Salticidae). ZooKeys 909:25–57. DOI: 10.3897/zookeys.909.47137.
- Whyte R, Anderson G. 2017. A field guide to spiders of Australia. Clayton, Australia: CSIRO Publishing. 464 pp.
- World Spider Catalog. 2021. World Spider Catalog. Version 21.5. Natural History Museum Bern. Available: http://wsc.nmbe.ch. Accessed Feb 2021.
- Żabka M. 1988. Salticidae (Araneae) of Oriental, Australian and Pacific regions, III. Annales Zoologici 41:421–479.
- Żabka M. 1991. Studium taksonomiczno-zoogeograficzne nad Salticidae (Arachnida: Araneae) Australii. Wyższa Szkoła Rolniczo-Pedagogiczna w Siedlcach Rozprawa Naukowa 32:1–110.
- Yamasaki T, Yamaguchi M, Phung LTH, Huang PS, Tso IM. 2018. Redescription of Chrysilla lauta Thorell 1887 (Araneae: Salticidae) based on the comparison with the holotype, and DNA barcoding. Acta Arachnologica 67(1):23–29. DOI: 10.2476/asjaa.67.23.
- Zhang J, Maddison W. 2013. Molecular phylogeny, divergence times and biogeography of spiders of the subfamily Euophryinae (Araneae: Salticidae). Molecular Phylogenetics and Evolution 68:81–92. DOI: 10.1016/j.ympev.2013.03.017.
- Zhang J, Maddison W. 2015. Genera of euophryine jumping spiders (Araneae: Salticidae), with a combined molecular morphological phylogeny. Zootaxa 3938(1):001–147. DOI: 10.11646/zootaxa.3938.1.1.