Abstract
To evaluate whether ontogenetic development over the grow-out culture period can cause geographical plasticity of carapace shape in a certain region, geometric morphometric analysis was used, in this study, to determine the ontogenetic changes in the carapace morphology of Chinese mitten crabs (Eriocheir sinensis) originating from Yangcheng Lake, China, during a year of growth from coin-sized juveniles to market-sized adults. The morphological differences in the carapace throughout the year of culture were characterized using a 35-landmark point system. We used principal component analysis and linear discriminant analysis to determine morphological variation using thin-plate spline analysis and mesh deformation. During the growth process, the changes in the carapace were mainly concentrated in the third and fourth anterolateral teeth and on the M-shaped pattern. During the growth of male and female crabs throughout the year, the shape of the carapace changed considerably over the first six months. Afterwards, the shape of the carapace began to stabilize and could not be differentiated through discriminant analysis. This study is the first to use geometric morphometrics to analyze the ontogenetic changes in the carapace shape of E. sinensis crabs native to Yangcheng Lake. The results demonstrate that it takes a long time for the carapace morphology of native crabs to stabilize.
1. Introduction
Chinese mitten crabs, also known as Chinese hairy crabs (Eriocheir sinensis H. Milne Edwards, 1853), are claded in Crustacea, Decapoda, Grapsidae, and Eriocheir, and first recorded from the eastern pacific coast of China to the Korean Peninsula (Sui et al. Citation2009). Over the past century, E. sinensis have spread to Europe and America, causing the number of wild E. sinensis to continuously increase (Herborg et al. Citation2003; Rudnick et al. Citation2003). E. sinensis has had a long history in China, and it is an important aquaculture product throughout the country (Cheng et al. Citation2008; Wang et al. Citation2018), because it has a high nutritional value regarding protein, vitamins, and mineral elements (Chen et al. Citation2007). In 2019, the total production of E. sinensis was 778,682 tons (MOAC (Ministry of Agriculture,China) Citation2020), with cultivation occurring most frequently in the Yangtze River Basin (Zhang et al. Citation2018), particularly in Jiangsu Province. In the same year, the output of E. sinensis in Jiangsu Province alone reached 364,840 tons, which was nearly 50% of the country’s total output (MOAC (Ministry of Agriculture, China) Citation2020).
As a catadromous species, the Chinese mitten crab has a complex life history. Male and female crabs mate and spawn in estuarine brackish water during the late fall/winter. After the fertilized eggs hatch in the Spring, the planktonic larvae will develop from the zoea larval stages to the megalopal stage in brackish water. The megalopae then gradually metamorphose into early juvenile crab and further grow until they reach the coin-sized juvenile stage while migrating from brackish water to freshwater habitats. As such, commercial seed farms are always located in estuarine areas (particularly in the Yangtze River Estuary) and sell megalopae and coin-sized juvenile crabs. As the most important resource for the culture of this crab, the coin-sized juveniles will grow in freshwater systems (such as lakes and ponds) through 13–16 molts to become market-sized crabs with sexual maturity (Wang et al. Citation2016; Cheng et al. Citation2018; Chen et al. Citation2019; Jiang et al. Citation2020).
E. sinensis is cultured in many regions, but the area around Yangcheng Lake in Suzhou, Jiangsu Province, is the most renowned. In 2005, the General Administration of Quality Supervision, Inspection, and Quarantine recognized crabs harvested from this lake as native/genuine “Yangcheng Lake Hairy Crabs” to protect against the sale of falsely advertised crabs and to ensure the quality of the product. Currently, the main method used to avoid counterfeiting of E. sinensis from Yangcheng Lake is the application of encoded labels on the legitimate products. However, this technology has many limitations in its production and application. For example, the label is easy to lose, and the information encoded into the label is incomplete. Furthermore, because producing the label is relatively simple, many merchants have made imitation labels in order to obtain greater benefits. This counterfeiting has seriously affected the sales of legitimate E. sinensis from Yangcheng Lake. The actual annual output of native E. sinensis from Yangcheng Lake is approximately 2,000 tons, but the E. sinensis claimed to be sold on the market far exceeds the actual output. This is similar to the market returns of the Mexican Interdonato lemon and Thai jasmine rice (Cheajesadagul et al. Citation2013; Potortì et al. Citation2018). Of the counterfeit products, “bathed” market-sized crabs are the most common. These crabs are cultured in Yangcheng Lake for only a few days (similar to bathing) before being sold as genuine lake-raised crabs. However, native/genuine “Yangcheng Lake Hairy Crabs” should be cultured in the lake for at least six months from coin-sized juveniles to market-sized adults, according to the Chinese national standard GB/T 19957–2005 (AQSIQ & SAC (General Administration of Quality Supervision, Inspection and Quarantine of the People’s Republic of China & Standardization Administration of the People’s Republic of China) Citation2005).
Geometric morphometrics is an advanced method that was established in the late 20th century by combining morphometry and statistical shape analysis (Klingenberg Citation2010). Morphological measurements captured in discrete landmarks can be used to describe the characteristics of an organism’s morphological structure and conduct a multidimensional morphological difference analysis on its shape and size (Slice Citation2001) to determine differences in shape among sexes of the same species, for example (Fernández-Montraveta & Marugán-Lobón Citation2017; Balazadeh & Litvak Citation2018), as well as within (among populations) and between species (Adams et al. Citation2004; Echeverry et al. Citation2020; Li et al. Citation2021). In recent years, this method has been increasingly used for studying aquatic organism morphology, and it is most widely used on fish (Meng et al. Citation2018; Argolo et al. Citation2020; Regmi et al. Citation2021) and crustaceans (Sanchez et al. Citation2013; Hampton et al. Citation2014; Choo et al. Citation2021).
Geometric morphometrics can be conducted using either the outline or landmark method (Bookstein Citation1997; Adams et al. Citation2004; Klingenberg Citation2010; Mitteroecker et al. Citation2013). In our previous research, the landmark method was successfully applied to identify E. sinensis originating from different locations in the Yangtze River Basin and to accurately discriminate between “bathed crabs” and native crabs obtained from Yangcheng Lake (Zheng et al. Citation2017, Citation2021; Xue et al. Citation2020). Zheng et al. (Citation2017) first accurately distinguished market-sized E. sinensis crabs cultured in Yangcheng Lake and those cultured in an aquaculture pond in Bacheng Town, near Yangcheng Lake, by conducting geometric morphometrics analysis of their carapace shapes. The accuracy rates were 99% and 84% for the shape of the ventral region of the cephalothorax (i.e., abdomen) of male and female crabs, respectively, suggesting that the carapace would be more suitable in investigating shape variations in E. sinensis. Xue et al. (Citation2020) studied intra-population differences of “bathed” and genuine crabs, and found that one month of “bathing” the culture in the same purse seine in Yangcheng Lake was insufficient to converge the carapace shape profiles of the market-sized ecdemic E. sinensis crabs to those of the native crabs of the lake cultured following the criterion of GB/T 19957–2005. They could be accurately distinguished via geometric morphometrics analysis, with a discrimination rate of 100% before and after the one-month “bathing” period. In addition, Zheng et al. (Citation2021) reported that discriminant analysis using geometric morphometric profiles could accurately distinguish market-sized male and female crabs cultured in the lakes of Gehu, Luoma, Gucheng, Junshan, and Liangzi along the middle and lower reaches of the Yangtze River, with discrimination rates of 100%. In these three cases, although the “coin-sized” juvenile crabs were all purchased from the commercial seed farms in Nantong City, plasticity in carapace shape existed for local adaptation among the E. sinensis crabs at the same ontogenetic stage from different locations until cultured into market-sized adults. The phenomena of the above three cases suggest that the culture process in different lakes or ponds from coin-sized juveniles (usually around March) to market-sized adults (usually around October) caused the carapace shape of the juveniles with the same germplasm background to gradually diverge to adapt to the different environments. It is unclear whether further ontogenetic development during the aforementioned culture process can cause such geographical plasticity of carapace shape in different culture locations. However, to date, few corresponding studies have been conducted on the carapace shape evolution of crabs during grow-out culture (culture for coin-sized juvenile to market size crabs (Wang et al. Citation2016)) in a special farming environment (including the Yangcheng Lake). Therefore, this study used landmark-based geometric morphometrics to monitor morphological changes in the carapace of native “Yangcheng Lake Hairy Crabs” of Yangcheng Lake, monthly during a year-long culture period following the aforementioned standard GB/T 19957–2005. We also aimed to determine the period of time required for the crabs to exhibit relatively stable morphologies that are characteristic of adaptation to Yangcheng Lake, and provide a corresponding theoretical basis for future identification of native “Yangcheng Lake Hairy Crabs” cultured in Yangcheng Lake based on its morphology.
2. Materials and methods
2.1. Sample collection
Native E. sinensis were collected once a month from March 2018 (coin-sized juvenile stage; around 10 g and 12 g of body weight, 25 mm and 27 mm for the male and female crabs, respectively) () to February 2019 (adult stage) from the purse seine culture area of Yangcheng Lake (31°26.8111′ N, 120°49.4606′ E), Suzhou City, Jiangsu Province. Megalopae samples were obtained from a commercial E. sinensis seed farm in Rudong County, Nantong City, Jiangsu Province, near the Yangtze River estuary. They were then transported to an E. sinensis aquaculture base near Yangcheng Lake and cultured to coin-sized juveniles by local crab farmers. In March, the coin-sized crabs were released to the purse seine culture area in Yangcheng Lake to culture according to the Chinese national standard GB/T 19957–2005 (AQSIQ & SAC (General Administration of Quality Supervision, Inspection and Quarantine of the People’s Republic of China & Standardization Administration of the People’s Republic of China) Citation2005) to provide a basis for the identification of crabs developing in Yangcheng Lake (i.e., native “Yangcheng Lake Hairy Crabs”). The sexual dimorphism of the crabs can be identified by the shape of the abdomen (Czerniejewski et al. Citation2007; Yang et al. Citation2018). The carapaces and abdomens of Chinese mitten crabs are shown in ) and 1(c,d), respectively. The female and male abdomens are rounded (U-shaped) and narrow triangular (V-shaped), respectively (Gou et al. Citation1997; Bouma & Soes Citation2010 ; Zheng et al. Citation2021; )), and both consist of 6 abdominal segments and telson (Gou et al. Citation1997). Although no literature is available on the sexual dimorphism of the carapace of this crab species, in this study, MANOVA was performed jointly with superimposed landmark coordinates recorded from male and female crabs to confirm whether there is a significant difference between male and female crabs to determine whether it is necessary to separate male and female crabs for analysis.
Table I. Body measurements for the E. sinensis populations (mean ± SD)
Figure 1. Schematic diagram of E. sinensis; Carapaces of a. male and b. female crabs; Abdomens of c. male and d. female crabs. e. Map of landmarks on the carapace of E. sinensis and schematic diagram of the scaling standard. A and B are the widest parts of the carapace. f. Map of landmarks on the carapace of E. sinensis.
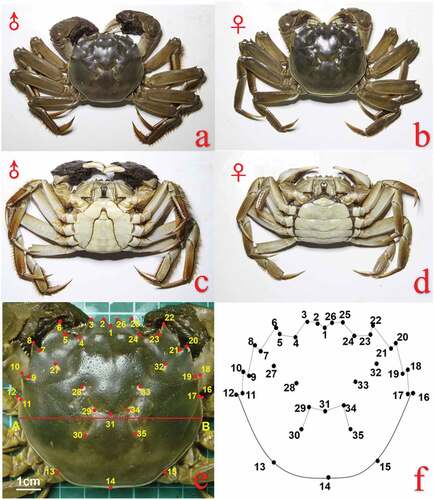
Each month during the study period, 20 crabs (10 females and 10 males) of similar size were sampled for morphological measurement, excluding February 2019, when only 17 crabs (7 females, 10 males) were sampled. The morphometric data for the sampled crabs are shown in .
2.2. Establishment and extraction of landmarks
Photographs of the samples were taken and standardized by scaling the carapace to a width of 10 cm ()). Then, a digital camera (Nikon COOLPIX P6000) was used to photograph the Yangcheng Lake crab samples. Subsequently, tpsDig2 version 2.12 (Rohlf Citation2008a) was used to establish homologous landmark points on the processed carapace photos ()), obtain their x and y coordinate values, and establish a data file of the surface points.
2.3 Analysis of average shape and spline
Using tpsSmall version 1.20 (Rohlf Citation2003), least-squares rule regression analysis was employed to test the validity of the landmark points. To calculate the centroid distance of the landmark points, tpsRelw version 1.46 (Rohlf Citation2008b) was used to perform Generalized Procrustes superimposition, accounting for the mean shape, relative distortion index matrix, and relative warp scores (Zheng et al. Citation2021). The relative contribution of each landmark was also calculated to analyze the differences in shape morphology using tpsRelw. A grid map of the crab carapace was obtained using tpsRegr version 1.36 (Rohlf Citation2009) to conduct a spline analysis, which allowed the morphological changes in the Yangcheng Lake E. sinensis carapace to be determined throughout ontogeny during the year-long culture period.
2.4 Statistical analysis
The program of Paleontological Statistics (PAST) (Hammer et al. Citation2001) was used to perform Multivariate analysis of variance (MANOVA) (Regmi et al. Citation2021). MANOVA was performed jointly with superimposed landmark coordinates recorded from male and female crabs to confirm whether there is a significant difference between male and female crabs. Additionally, PAST was used to perform discriminant analysis and principal component analysis (PCA) on the relative warp scores of each sample. Implementing PCA on the E. sinensis samples grouped by month allowed for a detailed visualization of how variables were loaded onto the carapaces (, b)). In addition, one-way ANOVA of the body measurements (body weight, body height, carapace length, carapace width) between males and females () was conducted using SPSS version 24.0 (IBM Corp., New York, USA).
Figure 2. Principal component analysis of the carapace morphology of E. sinensis from Yangcheng Lake; Principal component analysis scatter plots of a. male and b. female E. sinensis; Comparison of extreme value shapes from negative to positive for c. male and d. female E. sinensis (variation is enlarged by three times). The red line is negative extreme value shapes, while the black line is positive extreme value shapes.
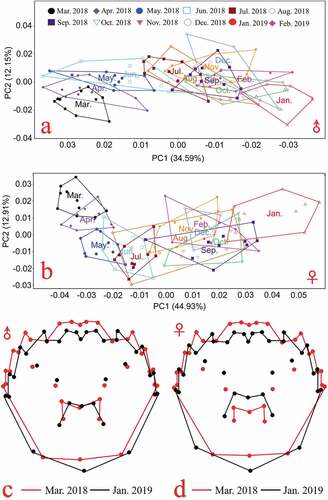
3. Results
3.1 Geometric morphometric analysis
Significant differences (P < 0.01) were particularly observed in the body weight, carapace length, and carapace width between males and females, especially after September. In contrast, there were almost no such significant differences in body height except for the data of April and September (). The MANOVA results showed that there were significant differences (P < 0.01) in the carapaces of male and female crabs in this study. Therefore, males and female crabs were separated for further multivariate analysis.
The regression coefficient of the tangent distance (y-axis) and the Procrustes distance (x-axis) of the landmark points on the male E. sinensis carapace was 0.997997, while that on the female crab carapace was 0.999710. As both of these coefficients were near to 1, the selected landmark points were considered effective and could be used for further geometric morphology research on this species.
In the analysis of landmarks on the male E. sinensis carapace, landmark points 11, 12, 16, and 17 had the highest contribution rates (13.53%, 11.50%, 16.62%, and 19.01%, respectively). These four points explained 60.66% of the differences in morphology of males over time. For female crabs, landmark points 11, 12, 16, and 17 also contributed the most of all the points, with contribution rates of 13.23%, 11.28%, 16.81%, and 19.16%, respectively. The four points explained 60.48% of the differences in female morphology over time.
To enhance the statistical power of analysis and compare the mesh deformation results (determined using tpsRegr software), all the results were magnified three times. The distribution in the monthly scatter plots of PCA scores for the crabs sampled from March 2018 to February 2019 indicated that the carapace shape of female crabs gradually changed from March to July (i.e., the monthly scatter plots gradually moved from the left to the right in turn in )). After August, the PC1 scores for crabs sampled between August 2018 to February 2019 did not differ, suggesting that the carapace shape stabilized in August. The grid map of the male E. sinensis carapace ()) shows that male crabs’ first (landmarks 5, 6, 22, 23) and second anterolateral teeth (i.e., spine) (landmarks 7, 8, 20, 21) shrank to the inner vertex. The fourth anterolateral teeth (landmarks 11, 12, 16, 17) extended downward, closer to the posterior edge, while the outer vertex of the fourth lateral tooth extended upward and outward. The three characteristic points of the posterior edge (landmarks 13, 14, 15) gradually expanded to the outside, while the M-shaped pattern (landmarks 29, 30, 34, 35) shrank towards the center, gradually occupying less space on the carapace. The remaining landmark points were not significantly different.
In the PCA ordination space of female E. sinensis from Yangcheng Lake, the distribution in the PC1 scatter plot for crabs sampled from March 2018 to February 2019 indicated that the female crab carapace shape gradually changed from March to July (i.e., the scatter plots gradually moved from right to left in )). After August, the scatter plots of monthly PCA scores for the crabs could not be distinguished, suggesting that the carapace shape stabilized, with the same trends as those in male crabs. The grid map of the female E. sinensis carapace throughout the year ()) revealed that the first (landmarks 5, 6, 22, 23) and second (landmarks 7, 8, 20, 21) anterolateral teeth of the female crab exhibited the same trends as those of the male crab, and they all shrank to the medial frontal edge. The lower apex of the shell length (landmark 14) had an obvious downward trend. The other two characteristic points (landmarks 13 and 15) on the rear edge extended to both sides, and the curvature at the rear edge became greater. The M-shaped pattern (landmarks 29, 30, 31, 34, and 35) extended to the outside and occupied a large area on the carapace. The remaining landmark points had no significant changes.
The visualizations of the differences in the carapaces of male and female E. sinensis obtained from Yangcheng Lake throughout the year (, d)) showed that the differences in the carapace are mainly located on the first, second, and fourth anterolateral teeth pairs, the M-shaped patterns, and the three landmark points on the rear edge, corresponding to the landmark points that had higher contribution rates.
3.2 Discriminant analysis
Based on the relative distortion scores of the male and female crabs collected from Yangcheng Lake throughout the year, discriminant analysis was conducted, and discriminant scatter diagrams describing the male and female crabs were obtained ().
Figure 3. Discriminant analysis scatter plot of Yangcheng Lake E. sinensis based on carapace morphology (95% confidence interval for ellipses); a. Male crab; b. Female crab.
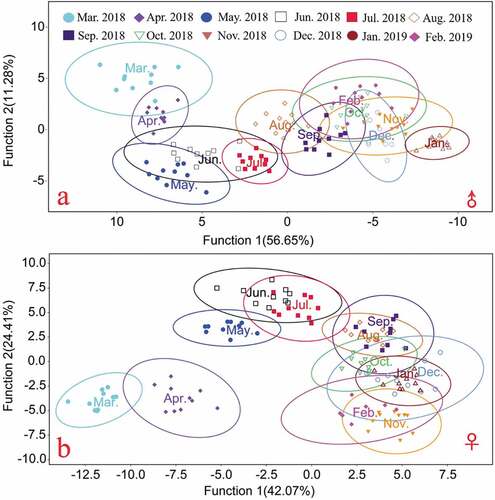
Discriminant analysis of the scatter plots of the male E. sinensis carapaces ()) showed clear morphological changes in the carapace from March to August 2018. Around September, the morphological changes in the carapace began to stabilize. After September, it was impossible to accurately identify male crab samples using discriminant analysis. Discriminant analysis of the female crab carapace ()) revealed the same trends as those in the male crab carapace.
4. Discussion
4.1 Morphological changes in Yangcheng Lake E. sinensis throughout ontogeny in the year-long culture period
Based on the analysis of morphological landmarks on the carapace of E. sinensis throughout the year, the landmark with the highest contribution rate was the fourth lateral tooth. In a previous analysis of the differences in carapace morphology between the Yangcheng Lake “bathed crab” and the Yangcheng Lake native crab, the landmark with the highest contribution rate was also the fourth lateral tooth (Xue et al. Citation2020). This result may be attributable to the special environment of Yangcheng Lake that has caused a significant change in morphology of the fourth lateral tooth. In the mesh deformation map analysis of the growth of the E. sinensis carapace throughout ontogeny in the year-long culture period, the landmark points that significantly changed, other than the fourth lateral tooth, included the M-shaped pattern and the back edge of the carapace. Similarly, in our previous study on crabs “bathed” for one month, the differences between the “bathed” and native Yangcheng Lake crabs were also concentrated on the fourth anterolateral teeth and M-shaped pattern (Xue et al. Citation2020). Zheng et al. (Citation2017) used grid visualization analysis to analyze landmark points on the carapaces of E. sinensis collected from eight different locations in the Yangtze River Basin and found the M-shaped pattern and the landmarks on the back edge of the carapace to be the most different parts. Almost no research has been conducted to explain the biological function of the fine-scale tooth/spine structures of crab carapaces and their development. Davis et al. (Citation2004) reported that the shift from long to short spines in hatchery-raised blue crabs (Callinectes sapidus), when compared to wild individuals, could be environmental plasticity related to the lack of exposure to predators, rather than hatchery-forced genetic drift. Zimmermann et al. (Citation2012) found a shape plasticity of carapace (e.g., thick vs. slender) and rostrum (e.g., short, robust, straight vs. thin long, orientated upward) in response to current speed in freshwater prawn Macrobrachium australe. Márquezab and Idaszkinbc (Citation2021) observed that the symmetric component (e.g., lateral teeth, cardiac and hepatopancreas sutures) of carapace shape variation in the crab Neoelice granulate responded to polluted and unpolluted habitats. Although the significance and mechanisms of monthly variations in certain fine structures of the carapace monitored by the landmarks are still unknown in the present study, it could be assumed that these shape changes might function more as an adaptation to biotic (e.g., predator presence) and abiotic (e.g., substrate type, water velocity, contaminant level, aquaculture operations) factors or pressures in the environments of Yangcheng Lake. Future further research will be necessary to confirm the validity of this assumption.
4.2 Analysis of the morphological characteristics in Yangcheng Lake
Analytical methods for determining the geographic origin of aquatic organisms include the bionic sensory technology (Rodríguez-Méndez et al. Citation2009; Zhang et al. Citation2012), element “fingerprint” analysis (Miyan et al. Citation2016; Avigliano et al. Citation2017; Luo et al. Citation2019), and isotope “fingerprint” analysis (Kim et al. Citation2015; Zhang et al. Citation2019). All these methods can accurately identify aquatic organisms of different varieties or from different origins. However, shape analysis is advantageous because it is non-lethal, fast, and has good application prospects. This study used geometric morphometrics to study the carapace morphology of Yangcheng Lake E. sinensis and provides theoretical support for the protection of crabs native to Yangcheng Lake. In a related study, Vignon (Citation2012) studied the otolith morphology of Lutjanus kasmira. In the early life stages, the otolith shape is determined by genetics, but after the aquatic environment changes, the otoliths of different individuals gradually differ in their growth direction and size. Sarà et al. (Citation1999) studied Diplodus puntazzo using the landmark method and found that, under different culture environments (monoculture in culture ponds, monoculture in offshore cages, and polyculture with Sparus aurata culture ponds), the shape of the fish adapts to the environment. Therefore, the landmark method can be used to distinguish organisms that grow under different aquaculture methods, as fish and crabs can change their original body shape after consistently living in a different habitat. Similarly, our previous studies indicated that regional differences in some of the morphological characteristics of Chinese mitten crab were associated with different culturing environments (Zheng et al. Citation2017, Citation2021; Xue et al. Citation2020). This means that the body shape gradually gains the characteristics of the production location. However, there are currently few studies on the time required to form the characteristics of each production location.
Based on the principal component scatter plots () and discriminant analysis (), the carapaces of both male and female crabs were observed to change significantly after they were transferred from the seed nursery pond in March to the Yangcheng Lake area for culture, indicating shape variation. The shape variation of the carapace of E. sinensis is believed to respond to biotic or abiotic environmental conditions and heterogeneities (such as the water current, depth, substrate type, predators, competitors, food availability, vegetation canopy, stone size; Zheng et al. (Citation2021)). The changes observed over the first three months are probably due to the substantial changes in the physical and chemical environment of the water body. Afterward, the rate of change slowed, but it did not immediately stabilize. Around September, it became impossible to distinguish E. sinensis crabs from different months through discriminant analysis or principal component analysis. It appears to coincide with the sexual maturity of the crab. In this study, the megalopae were obtained from a commercial E. sinensis farm in Rudong County, Nantong City, Jiangsu Province, and transported to an E. sinensis aquaculture base and cultured to coin-sized juveniles. The coin-sized crabs were then released to the purse seine culture area of Yangcheng Lake to culture according to the Chinese national standard GB/T 19957–2005 (AQSIQ & SAC (General Administration of Quality Supervision, Inspection and Quarantine of the People’s Republic of China & Standardization Administration of the People’s Republic of China) Citation2005) to allow crabs originating in Yangcheng Lake (i.e., genuine/native Yangcheng E. sinensis) to be designated. Typically, the crab would reach the terminal molt and sexual maturity in September (Cheng et al. Citation2018). We can infer that the carapace morphology of Yangcheng Lake E. sinensis tends to be stable, and it takes about six months to form the morphologies characteristic of the production location. For this reason, it was expected that one month of deliberately “bathing” crabs in Yangcheng Lake (Xue et al. Citation2020) was insufficient to converge the carapace shape profiles of the market-sized ecdemic E. sinensis crabs to those of the native crabs of the lake cultured according to the criterion of GB/T 19957–2005, as one month is insufficient for shape evolution. The results of this study provide a theoretical basis for distinguishing native crabs from “bathed crabs”, which cannot change their morphologies to look like native Yangcheng Lake crabs in such a short period of time. Furthermore, the six-month formation time of the morphologies that are characteristic of the place of origin is consistent with the definition of native “Yangcheng Lake Hairy Crabs” recognized by the GB/T 19957–2005. Therefore, our results provide a stronger theoretical basis for the definition of this geographical indication product.
5. Conclusions
Eriocheir sinensis cultured in Yangcheng Lake is a rare and precious aquatic product in China. The sale of counterfeit crabs claiming Yangcheng Lake origin has repeatedly been prohibited. Nevertheless, the selling of fake products such as “bathed crab” is common. This study is the first to use geometric morphometrics to analyze the temporal changes in the carapace shape of E. sinensis crabs native to Yangcheng Lake. The results indicate that it takes 6 months for the carapace morphology of the native crabs to stabilize and reflect their place of origin. This result is of great significance for the protection of the original habitat of the crab in Yangcheng. The results of the present study will be useful for demonstrating that the Yangcheng Lake E. sinensis morphologies that are characteristic of the production origin are representative, for contributing towards related research on the protection of crabs native to Yangcheng Lake, and for providing a reference for the protection of the locations of other products made famous based on their geographical origins. In future research, we plan to further compare crabs native to Yangcheng Lake across different years and from different areas of the lake.
Disclosure statement
No potential conflict of interest was reported by the author(s).
Additional information
Funding
References
- Adams DC, Rohlf FJ, Slice DE. 2004. Geometric morphometrics: Ten years of progress following the ‘revolution’. Italian Journal of Zoology 71(1):5–16. DOI:10.1080/11250000409356545.
- AQSIQ & SAC (General Administration of Quality Supervision, Inspection and Quarantine of the People’s Republic of China & Standardization Administration of the People’s Republic of China), 2005. GB/T19957-2005: Product of designations of origin or geographical indication of Yangchen Lake Chinese mitten crab. (in Chinese)
- Argolo LA, López-Fernández H, Batalha-Filho H, de Mello Affonso PRA. 2020. Unraveling the systematics and evolution of the ‘Geophagus’ brasiliensis (Cichliformes: Cichlidae) species complex. Molecular Phylogenetics and Evolution 150:106855. DOI: 10.1016/j.ympev.2020.106855.
- Avigliano E, Domanico A, Sánchez S, Volpedo AV. 2017. Otolith elemental fingerprint and scale and otolith morphometry in Prochilodus lineatus provide identification of natal nurseries. Fisheries Research 186:1–10. DOI: 10.1016/j.fishres.2016.07.026.
- Balazadeh K, Litvak MK. 2018. Using geometric morphometrics for sex determination on adult shortnose sturgeon (Acipenser brevirostrum). Aquaculture 487:89–96. DOI: 10.1016/j.aquaculture.2017.12.047.
- Bookstein FL. 1997. Morphometric tools for landmark data: Geometry and biology. Cambridge: Cambridge University Press.
- Bouma, S., and Soes, D.M., 2010. ”A risk analysis of the Chinese mitten crab in The Netherlands. Culemborg: Bureau Waardenburg bv (Consultants for environment & ecology)”. This literature is a research report published by Bureau Waardenburg bv in Culemborg of Netherlands
- Cheajesadagul P, Arnaudguilhem C, Shiowatana J, Siripinyanond A, Szpunar J. 2013. Discrimination of geographical origin of rice based on multi-element fingerprinting by high resolution inductively coupled plasma mass spectrometry. Food Chemistry 141(4):3504–3509. DOI:10.1016/j.foodchem.2013.06.060.
- Chen X, Wang J, Hou X, Yue W, Huang S, Wang C. 2019. Tissue expression profiles unveil the gene interaction of hepatopancreas, eyestalk, and ovary in the precocious female Chinese mitten crab, Eriocheir sinensis. BMC Genetics 20(1):2. DOI:10.1186/s12863-019-0716-1.
- Chen D-W, Zhang M, Shrestha S. 2007. Compositional characteristics and nutritional quality of Chinese mitten crab (Eriocheir sinensis). Food Chemistry 103(4):1343–1349. DOI:10.1016/j.foodchem.2006.10.047.
- Cheng Y, Wu X, Li J. 2018. Chinese mitten crab culture: Current status and recent progress towards sustainable development. In: Gui J, Tang Q, Li Z, Liu J, De Silva SS, editors. Aquaculture in China: Success stories and modern trends. Oxford: John Wiley & Sons Ltd. pp. 197–217.
- Cheng Y, Wu X, Yang X, Hines AH. 2008. Current trends in hatchery techniques and stock enhancement for Chinese mitten crab, Eriocheir japonica sinensis. Rev. Fish. Sci 16(1–3):377–384. DOI:10.1080/10641260701681698.
- Choo LQ, Bal TMP, Goetze E, Peijnenburg KTCA. 2021. Oceanic dispersal barriers in a holoplanktonic gastropod. Journal of Evolutionary Biology 34(1):224–240. DOI:10.1111/jeb.13735.
- Czerniejewski P, Wawrzyniak W, Pasewicz W, Bełdowska A. 2007. A comparative analysis of two allochthonous populations of the Chinese mitten crab (Eriocheir sinensis H. Milne-Edwards, 1853) from the Szczecin Lagoon (NW Poland) and San Francisco Bay (US west coast). Oceanologia 49(3):353–367.
- Davis JLD, Young-Williams AC, Aguilar R, Carswell BL, Goodison MR, Hines AH, Kramer MA, Zohar Y, Zmora O. 2004. Differences between hatchery-raised and wild blue crabs: Implications for stock enhancement potential.Transactions of the American Fisheries Society 133(1):1–14. DOI:10.1577/T03-004.
- Echeverry AM, Londoño-Cruz E, Benítez HA. 2020. Quantifying the geometric shell shape between populations of true limpets Lottia Mesoleuca (Mollusca: Lottidae) in Colombia. Animals 10(4):675. DOI:10.3390/ani10040675.
- Fernández-Montraveta C, Marugán-Lobón J. 2017. Geometric morphometrics reveals sex-differential shape allometry in a spider. PeerJ 5:e3617. DOI: 10.7717/peerj.3617.
- Gou JY, Ng NK, Dai A, Ng PKL. 1997. The taxonomy of three commercially important species of mitten crabs of the genus Eriocheir De Haan, 1835 (Crustacea: Decapoda: Brachyura: Grapsidae). Raffles B. Zool 45:445–476.
- Hammer Ø, Harper DAT, Ryan PD. 2001. PAST: Paleontological Statistics Software Package for Education and Data Analysis. Palaeontol. Electron 4(1):art. 4. http://palaeo-electronica.org/2001_1/past/issue1_01.htm.
- Hampton KR, Hopkins MJ, McNamara JC, Thurman CL. 2014. Intraspecific variation in carapace morphology among fiddler crabs (Genus Uca) from the Atlantic coast of Brazil. Aquatic Biology 20(1):53–67. DOI:10.3354/ab00545.
- Herborg LM, Rushton SP, Clare AS, Bentley MG. 2003. Spread of the Chinese mitten crab (Eriocheir sinensis H. Milne Edwards) in Continental Europe: Analysis of a historical data set. In: Jones MB, Ingólfsson A, Ólafsson E, Helgason GV, Gunnarsson K, Svavarsson J, editors. Migrations and Dispersal of Marine Organisms, Developments in Hydrobiology. vol. 174. Dordecht: Springer. pp. 21–28. DOI:10.1023/B:HYDR.0000008483.63314.3c.
- Jiang X, Wang H, Cheng Y, Wu X. 2020. Growth performance, gonad development and nutritional composition of Chinese mitten crab Eriocheir sinensis selected for growth and different maturity time. Aquaculture 523:735194. DOI: 10.1016/j.aquaculture.2020.735194.
- Kim H, Kumar KS, Shin K-H. 2015. Applicability of stable C and N isotope analysis in inferring the geographical origin and authentication of commercial fish (Mackerel, Yellow Croaker and Pollock). Food Chemistry 172:523–527. DOI: 10.1016/j.foodchem.2014.09.058.
- Klingenberg CP. 2010. Evolution and development of shape: Integrating quantitative approaches. Nature Reviews Genetics 11(9):623–635. DOI:10.1038/nrg2829.
- Li Y, Burridge CP, Lv Y, Peng Z. 2021. Morphometric and population genomic evidence for species divergence in the Chimarrichthys fish complex of the Tibetan Plateau. Molecular Phylogenetics and Evolution 159:107117. DOI: 10.1016/j.ympev.2021.107117.
- Luo R, Jiang T, Chen X, Zheng C, Liu H, Yang J. 2019. Determination of geographic origin of Chinese mitten crab (Eriocheir sinensis) using integrated stable isotope and multi-element analyses. Food Chemistry 274:1–7. DOI: 10.1016/j.foodchem.2018.08.104.
- Márquezab F, Idaszkinbc Y. 2021. Crab carapace shape as a biomarker of salt marsh metals pollution. Chemosphere 276:130195. DOI: 10.1016/j.chemosphere.2021.130195.
- Meng Y, Wang G, Xiong D, Liu H, Liu X, Wang L, Zhang J. 2018. Geometric morphometric analysis of the morphological variation among three lenoks of genus Brachymystax in China. Pak. Journal of Zoology 50(3):885–895. DOI:10.17582/journal.pjz/2018.50.3.809.815.
- Mitteroecker P, Gunz P, Windhager S, Schaefer K. 2013. A brief review of shape, form, and allometry in geometric morphometrics, with applications to human facial morphology. Hystrix 24(1):59–66. DOI:10.4404/hystrix-24.1-6369.
- Miyan K, Khan MA, Patel DK, Khan S, Ansari NG. 2016. Truss morphometry and otolith microchemistry reveal stock discrimination in Clarias batrachus (Linnaeus, 1758) inhabiting the Gangetic river system. Fisheries Research 173:294–302. DOI: 10.1016/j.fishres.2015.10.024.
- MOAC (Ministry of Agriculture, China), 2020. China Fisheries Yearbook, 2020. China Agriculture Publisher, Beijing. (in Chinese).
- Potortì AG, Di Bella G, Mottese AF, Bua GD, Fede MR, Sabatino G, Salvo A, Somma R, Dugo G, Lo Turco V. 2018. Traceability of Protected Geographical Indication (PGI) Interdonato lemon pulps by chemometric analysis of the mineral composition. Journal of Food Composition and Analysis 69:122–128. DOI: 10.1016/j.jfca.2018.03.001.
- Regmi B, Douglas MR, Edds DR, Douglas ME. 2021. Geometric morphometric analyses define riverine and lacustrine species flocks of Himalayan snowtrout (Cyprinidae: Schizothorax) in Nepal. Aquatic Biology 30:19–31. DOI: 10.3354/ab00737.
- Rodríguez-Méndez ML, Gay M, Apetrei C, De Saja JA. 2009. Biogenic amines and fish freshness assessment using a multisensor system based on voltammetric electrodes. Comparison between CPE and screen-printed electrodes. Electrochimica Acta 54(27):7033–7041. DOI:10.1016/j.electacta.2009.07.024.
- Rohlf FJ. 2003. TpsSamll (version 1.20). New York: Department of Ecology and Evolution, State University of New York at Stony Brook.
- Rohlf FJ. 2008a. TpsDig (version 2.12). New York: Department of Ecology and Evolution, State University of New York at Stony Brook.
- Rohlf FJ. 2008b. TpsRelw (version 1.46). New York: Department of Ecology and Evolution, State University of New York at Stony Brook.
- Rohlf FJ. 2009. TpsRegr (version 1.36). New York: Department of Ecology and Evolution, State University of New York at Stony Brook.
- Rudnick DA, Hieb K, Grimmer KF, Resh VH. 2003. Patterns and processes of biological invasion: The Chinese mitten crab in San Francisco Bay. Basic Appl. Ecol 4(3):249–262. DOI:10.1078/1439-1791-00152.
- Sanchez MLS, Gorospe JG, Gorospe JN, Torres MAJ, Demayo CG. 2013. Describing geographic differences in carapace shape in the blue swimming crab Portunus pelagicus from Mindanao Bays, Philippines. Aquac. Aquar. Cons. Legis 6(6):622–634. http://www.bioflux.com.ro/aacl.
- Sarà M, Favaloro E, Mazzola A. 1999. Comparative morphometrics of sharpsnout seabream (Diplodus puntazzo Cetti, 1777), reared in different conditions. Aquacultural Engineering 19(3):195–209. DOI:10.1016/S0144-8609(98)00052-1.
- Slice DE. 2001. Landmark coordinates aligned by procrustes analysis do not lie in Kendall’s shape space.Systematic Biology 50(1):141–149. DOI:10.1080/10635150119110.
- Sui L, Zhang F, Wang X, Bossier P, Sorgeloos P, Hänfling B. 2009. Genetic diversity and population structure of the Chinese mitten crab Eriocheir sinensis in its native range. Marine Biology 156(8):1573–1583. DOI:10.1007/s00227-009-1193-2.
- Vignon M. 2012. Ontogenetic trajectories of otolith shape during shift in habitat use: Interaction between otolith growth and environment. Journal of Experimental Marine Biology and Ecology 420-421:26–32. DOI: 10.1016/j.jembe.2012.03.021.
- Wang Q, Liu J, Zhang S, Lian Y, Ding H, Du X, Li Z, De Silva SS. 2016. Sustainable farming practices of the Chinese mitten crab (Eriocheir sinensis) around Hongze Lake, lower Yangtze River Basin, China. Ambio 45(3):361–373. DOI:10.1007/s13280-015-0722-0.
- Wang J, Xu P, Zhou G, Li X, Lu Q, Liu X, Zhou J, Wang C. 2018. Genetic improvement and breeding practices for Chinese mitten crab, Eriocheir sinensis. Journal of the World Aquaculture Society 49(2):292–301. DOI:10.1111/jwas.12500.
- Xue JR, Jiang T, Chen XB, Luo RJ, Liu HB, Yang J. 2020. Geometric morphometric analysis on the carapace difference between “bathed” and Yangcheng Lake originated Eriocheir sinensis. Acta Hydrobiol. Sin 44(3):587–594 10.7541/2020.072 (in Chinese with English abstract)
- Yang Z, Wei B, Liu Q, Cheng Y, Zhou J. 2018. Individual growth pattern of juvenile stages of the Chinese mitten crab (Eriocheir sinensis) reared under laboratory conditions. Aquaculture International 26(2):645–657. DOI:10.1007/s10499-018-0239-1.
- Zhang X, Cheng J, Han D, Zhao X, Chen X, Liu Y. 2019. Geographical origin traceability and species identification of three scallops (Patinopecten yessoensis, Chlamys farreri, and Argopecten irradians) using stable isotope analysis. Food Chemistry 299:125107. DOI: 10.1016/j.foodchem.2019.125107.
- Zhang C, Li Q, Wu X, Liu Q, Cheng Y. 2018. Genetic diversity and genetic structure of farmed and wild Chinese mitten crab (Eriocheir sinensis) populations from three major basins by mitochondrial DNA COI and Cyt b gene sequences. Mitochondrial DNA Part A 29(7):1081–1089. DOI:10.1080/24701394.2017.1404048.
- Zhang M, Wang X, Liu Y, Xu X, Zhou G. 2012. Species discrimination among three kinds of puffer fish using an electronic nose combined with olfactory sensory evaluation. Sensors 12(9):12562–12571. DOI:10.3390/s120912562.
- Zheng C, Jiang T, Luo R, Chen X, Liu H, Yang J. 2017. Landmark-based morphometric identification of different geographical origins for the Chinese mitten crab (Eriocheir sinensis). J. Fish. China 41(12):1896–1907 10.11964/jfc.20170210715 (in Chinese with English abstract)
- Zheng C, Jiang T, Luo R, Chen X, Liu H, Yang J. 2021. Geometric morphometric analysis of the Chinese mitten crab Eriocheir sinensis : A potential approach for geographical origin authentication. North American Journal of Fisheries Management 41(4):891–903. DOI:10.1002/nafm.10396.
- Zimmermann G, Bosc P, Valade P, Cornette R, Améziane N, Debat V. 2012. Geometric morphometrics of carapace of Macrobrachium australe (Crustacea: Palaemonidae) from Reunion Island. Acta Zoologica 93(4):492–500. DOI:10.1111/j.1463-6395.2011.00524.x.