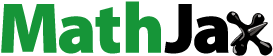
Abstract
Environmental changes related to global warming are both a threat to cold-water fishes and simultaneously create favourable conditions for the spread of eurythermic or warm-water species. In both cases, it is necessary to develop conservation strategies supported by precise ecological data, including thermal requirements. In this study, we determined the upper thermal tolerance thresholds and the critical maxima for three small, non-commercial Eurasian freshwater fish species; i.e. lake minnow Eupallasella (=Rhynchocypris) percnurus, sunbleak Leucaspius delineatus and topmouth gudgeon Pseudorasbora parva using the Critical Thermal Method at different acclimation temperatures, i.e. 18, 23, 28, and 33°C (highest treatment for topmouth gudgeon only). We hypothesized that lake minnow would have the lowest thermal tolerance and the topmouth gudgeon the highest. The response to temperature rise and the upper thermal limits were species-specific and correlated with the acclimation temperature, but not with fish length. Sunbleak showed the lowest thermal tolerance, though at 28°C both E. percnurus and L. delineatus reached a similar critical thermal limit. Topmouth gudgeon showed distinctly higher upper thresholds of thermal tolerance and at the highest acclimation temperature the critical upper limit for the species was close to 42°C. The results obtained for L. delineatus were surprising as we predicted that this leuciscid fish would be more tolerant of high temperatures than E. percnurus. We discuss the results in relation to the threat of extinction and the risk of species spreading beyond their natural range in the context of a warming environment.
Introduction
Among abiotic factors, temperature has a major impact on the life functions of aquatic organisms, especially ectothermic species. In fishes, development, growth rate, the onset and duration of the spawning period, as well as survival rates are strictly temperature-dependent (Fry Citation1971; Elliott Citation1981; Wieser Citation1991). Individual fish species have different thermal requirements and acknowledging these differences allows implementing both economic and protective measures to improve their living environment, breeding, or cultivation. This is particularly important in the face of increasing climatic instability, which potentially affects the thermal conditions of aquatic environments (Harrod Citation2016; Dokulil et al. Citation2021). Climate changes observed in recent years include, inter alia, deterioration of available habitats in the case of freshwater fishes, mostly as a result of disturbances to flow dynamics or increase in water temperatures. Consequently, the assemblage structure of freshwater fishes may undergo transformations, for example by a gradual disappearance of native, cold-water species and the expansion of alien, eurytopic ones (Britton et al. Citation2010; Jeppesen et al. Citation2010; Comte et al. Citation2013; Rolls et al. Citation2017). In Central European water bodies the spread of non-native species, such as gibel carp Carassius gibelio, Chinese sleeper Perccottus glenii and topmouth gudgeon Pseudorasbora parva, is clearly visible. Simultaneously, disappearance of native species such as lake minnow Eupallasella (=Rhynchocypris) percnurus and sunbleak Leucaspius delineatus is observed (Gozlan et al. Citation2005; Witkowski & Grabowska Citation2012; Wolnicki et al. Citation2022), which can partly be explained by changes in the environment related to the warming process.
Climate change has promoted a research interest in the thermal requirements of fishes. However, small-bodied fish species of limited commercial value, such as E. percnurus, L. delineatus or P. parva, do not attract as much research attention as species with greater economic significance, such as salmonids. Lake minnow is currently protected by national and European law as the priority species within the European Ecological Natura 2000 network, while natural populations of sunbleak are protected by the Bern Convention (Freyhof & Brooks Citation2011; Wolnicki et al. Citation2022). In contrast, the topmouth gudgeon is considered a serious biological threat to many species in European waters (Gozlan Citation2012; Baltazar-Soares et al. Citation2020). However, it could be concluded that all three species have an underestimated biological and economical potential, either for their conservation or as a biological threat when invasive (Zięba et al. Citation2010; Interesova Citation2012; Reshetnikov et al. Citation2017). Data on the thermal requirements of these species, particularly critical lethal or sublethal temperature thresholds, are sparse or imprecise. The exceptions are studies related to the optimal temperature for growth and egg development of lake minnow (Wolnicki et al. Citation2004; Kamiński et al. Citation2006). Nevertheless, the knowledge gap of these species’ thermal requirements limits the ability to develop successful conservation strategies, either in terms of protection against extinction or invasion.
Of the various methodologies for determining critical temperatures for fish, the critical thermal method (CTM) has the advantage of the possibility of establishing the temperature limits (CTmax or CTmin) without reaching a lethal endpoint, in contrast to an incipient lethal temperature (ILT) or chronic lethal temperature (CLT) (Fry Citation1971; Beitinger et al. Citation2000). Moreover, CTM is a rapid and convenient method which does not require any complicated apparatus or a large sample size (Currie et al. Citation1998; Rajaguru Citation2002; Mora & Maya Citation2006). It is also recommended for small-bodied fish species (e.g. Beitinger et al. Citation2000; Carveth et al. Citation2006; Mora & Maya Citation2006). The CTM, classed as a dynamic method, consists of raising (or lowering) the ambient temperature for fish at a constant, strictly defined rate. The critical limit of thermal tolerance is defined as a pre-death thermal point at which locomotory functions become disorganized, preventing fish from escaping conditions that result in death (Fry Citation1971; Becker & Genoway Citation1979; Beitinger et al. Citation2000). In this case, a precise definition of the sublethal endpoint is required, which is most often expressed as loss of equilibrium in fish (LOE). After the fish have reached the sublethal phase (heat coma) and have been transferred back to an acclimation temperature, the individuals undergo recovery of vital functions (Currie et al. Citation1998, Beitinger et al. Citation2000; Noyola et al. Citation2015). After undergoing this process, tested fish can be released back into their natural environment. Therefore, the method is recommended, especially for endangered and protected species (Beitinger et al. Citation2000; Carveth et al. Citation2006; Dalvi et al. Citation2009). The CTM allows the establishment of an upper tolerance threshold, similarly to lethal methods, but without killing test fish specimens (Fry Citation1971; Beitinger et al. Citation2000).
In this study, we aimed at identifying upper critical temperatures of three freshwater fishes, lake minnow (E. percnurus), sunbleak (L. delineatus), and topmouth gudgeon (P. parva), to assess their capacity to withstand thermal stress in their natural environment and predict their capability to populate new habitats. We hypothesized that E. percnurus would exhibit the lowest thermal tolerance, L. delineatus will be at an intermediate level, and P. parva will show the highest thermal tolerance. We discuss our results in the context of the potential outcomes for the studied species in a warming environment.
Material and methods
Fish collection and acclimation
The tested fish species were sampled between June and September 2019 in various types of water bodies located in northern Poland. Lake minnow specimens were collected in a natural isolated pond situated near the village of Egiertowo in the Kashubian Lakeland (54.228614 N, 18.196273 E). Sunbleaks were obtained from a small channel in the River Pasłęka system (Vistula Lagoon basin; 53.907250 N, 20.195778 E), while topmouth gudgeon was caught in the Struga Rychnowska stream, a tributary of the River Drwęca (lower River Vistula system; 53.095285 N, 18.826229 E). After capture, fish were transported to the laboratory and placed in aquaria connected with a recirculation aquaculture system (RAS). This system consisted of three independent units, each of 900 l capacity, with the ability to regulate water temperature. Total length of each fish specimen was measured to the nearest 0.1 cm and only adult specimens (TL > 4.0 cm) were used for further procedure. All individuals of fish species were randomly divided into separate groups for thermal acclimation in 40 l aquaria. For all three species, individuals were acclimated gradually (ca 1°C day−1) to reach the target temperatures of 18.0°C, 23.0°C, and 28.0°C (± 0.2°C). In addition, topmouth gudgeon was acclimated at 33.0°C. The highest acclimation temperatures were chosen on the basis of our own experience and the published literature, with the aim that the normal physiological functions of the fish would not be compromised. Previous attempts to acclimate fish at temperatures higher than those selected failed due to weight loss of the fish. A photoperiod of 16 h: 8 h light/dark was imposed. These conditions were maintained for two weeks prior to CTM testing. Fishes were fed with frozen chironomid larvae and commercial aquarium dry food, and were fasted for 24 hours before testing.
CTM testing procedure
After acclimation, CTmax tests were conducted in 10 l plastic heating chambers equipped with a 300 W submersion heater (Eliko, Poland). During experiments, water was lightly aerated through two finely perforated air stones, which permitted equalization of the temperature in the chamber, but did not force the fish to expend additional energy in swimming against water movement. Prior to each trial several fish (no more than five) were randomly selected from a particular acclimation temperature and placed in a small basket submerged in the heating chamber. Test fish were subsequently allowed to settle for 10–15 minutes. Water temperature was recorded using a calibrated mercury thermometer with an accuracy of 0.1°C, and oxygenation was measured with an oximeter (OxyGuard Handy Polaris ver.1.26) placed inside the basket. During the experiments the oxygen saturation of the water was maintained at 80–105%, to prevent the oxygen concentration from falling below 5 mg l−1.
For each acclimation temperature, experiments were carried out in three trials, except in the case of lake minnow acclimated at 23°C in which two trials were performed. Each fish was used only once for tests. The experiments were started at a given acclimation temperature, and the temperature rise was maintained at the recommended rate for small-bodied fish species of 0.3°C min −1 (Becker & Genoway Citation1979; Currie et al. Citation1998; Beitinger et al. Citation2000; Carveth et al. Citation2006). During tests, fish behaviour was carefully observed and any disturbance at a specific temperature was registered independently by two persons. The first sign of a loss of balance (failure to maintain dorso-ventral orientation) of a fish individual was considered as an initial loss of equilibrium (ILOE). However, for most individuals, this phase was only temporary and they quickly recovered normal buoyancy during temperature increase. Thus, the upper thermal limit (CTmax) was considered as a final loss of equilibrium (Becker & Genoway Citation1979; Carveth et al. Citation2006; Eme & Bennett Citation2009), and the moment a fish lost the ability to maintain dorso-ventral orientation for 15 second was defined as the critical endpoint. This criterion was established to represent the point at which the individual lost the capacity to escape unfavourable conditions while also avoiding the risk of reaching a lethal endpoint (Beitinger et al. Citation2000). This allowed us to release experimental fish back to their natural habitat after surveys, which is crucial in the case of lake minnow since this is an endangered and protected species. When an individual reached the CTmax endpoint, the temperature was precisely recorded and the individual was removed from the basket, measured (total length) and returned to an aquarium at its original acclimation temperature. Each trial was continued until the final loss of equilibrium was recorded in all tested individuals. ILOE and CTmax for all species were calculated as an arithmetic mean of the collective thermal points at which the given endpoint was reached. After the termination of the tests, all specimens were monitored until recovery. Lake minnow individuals were then released into the same water body in which they had been caught.
Statistical analysis
CTmax values were regressed on acclimation temperature and analysis of covariance (ANCOVA) was used to establish the differences in coefficients of regressions among species. The significance of observed variation was analysed with the LSD Fisher post-hoc test. To test the effects of body size on CTmax, total length (TL, in cm) was included in the Gaussian Generalized Model (GLM) as a covariate with fish species (i) and acclimation temperature Tacc. (j). The model was specified as:
The model was fitted using R version 4.1.2 (R Development Core Team Citation2021).
CTmax – ILOE differences were analysed using nested analysis of variance (ANOVA), where the temperature of acclimation was nested in species (Zar Citation2010). This difference is a function of time and determines the rate at which the sublethal response of the fish is achieved with a constant temperature increase. To meet assumptions of analyses, diagnostic plots of residuals against fitted values and QQ plot of residuals were used to assess the normality of residuals. Homoscedasticity was tested using the Levene test. If the results of ANOVA showed a significant effect, the LSD Fisher post-hoc test was used to identify which factors differed significantly. Probability values (p) < 0.05 were considered significant. Data are reported as mean ± standard deviation (SD). Statistical analyses were conducted using STATISTICA 13 (Dell Inc Citation2016).
Results
During the tests lake minnow and sunbleak frequently appeared stressed by the temperature increase, in contrast to the topmouth gudgeon which failed to express a behavioural response. The lowest ILOE value was observed for sunbleak acclimated at 18°C and occurred at temperatures slightly above 30.0°C, which was about two degrees below ILOE for lake minnow (). For topmouth gudgeon, ILOE values were highest, and the first symptoms of loss of balance were observed at a temperature exceeding 33.0°C. Acclimation temperatures of 18.0°C and 23.0°C in case of sunbleak resulted in the lowest upper thermal tolerance threshold (CTmax) among all tested species, whereas both the sunbleak and lake minnow acclimated at 28.0°C exhibited a similar upper critical temperature, which was in the range of 36.6–36.9°C ().
Table I. Characteristics of the tested fishes and values of upper temperature thresholds (initial loss of equilibrium – ILOE and critical thermal maximum – CTmax) at particular acclimation temperatures.
Among the three species, topmouth gudgeon showed the highest thermal tolerance. Fish acclimated to 28.0°C reached CTmax slightly above 40.0°C, i.e. over 3.0°C higher temperature than the other two species. In the case of the highest tested acclimation temperature (33.0°C), topmouth gudgeon had a threshold of critical temperature close to 42.0°C ().
CTmax of all species was positively correlated to acclimation temperature, and analysis of covariance revealed differences in slopes among species (F2,111 = 39.06, p < 0.001). Multiple comparisons (LSD Fisher post-hoc test) showed that all species differed significantly from one another (). The highest increase of CTmax in relation to acclimation temperature (b value in as an equivalent of acclimation response ratio, ARR) was recorded for sunbleak, and the lowest for lake minnow. Topmouth gudgeon increased its CTmax by 0.46 ± 0.01°C (mean ± SD) with each Celcius degree increase in acclimation temperature, whereas sunbleak and lake minnow increased their CTmax by 0.52 ± 0.03 and 0.28 ± 0.02, respectively. For topmouth gudgeon and sunbleak, the relationships were almost parallel (). The results are confirmed by the GLM, showing the effect of acclimation temperature on fish species, but no relation between fish size (TL) and CTmax was noted (). The overall model fit was highly significant (R2 = 0.952, df = 4.112, p < 0.001, residual standard error: 0.657) and the value of Akaike Information Criterion (AIC) was 240.5485.
Differences between CTmax and ILOE were significantly species-dependent (two-way nested ANOVA: F2,107 = 7.245, p < 0.001), as well as on the acclimation temperature nested in species (F7,107 = 39.404, p < 0.0001). In general, the differences between CTmax and ILOE decreased with temperature of acclimation, and therefore, for all species the largest differences were noted at 18.0°C (). The LSD Fisher post-hoc test revealed that at the lowest acclimation temperature top-mouth gudgeon had the highest value and there was no difference between sunbleak and lake minnow. In the case of the two latter species, the CTmax–ILOE difference was insignificant at acclimation temperatures of 23 and 28°C, though the lowest value was recorded for sunbleak at 28°C (). Surprisingly, lake minnow had a higher mean value of CTmax – ILOE differences at 28°C than at 23°C. No significant differences between mean values of the CTmax – ILOE difference were found for topmouth gudgeon at acclimation temperatures of 23°C, 28°C, and 33°C ().
Figure 1. The relationship between the upper thermal limit (CTmax) and fish acclimation temperature.
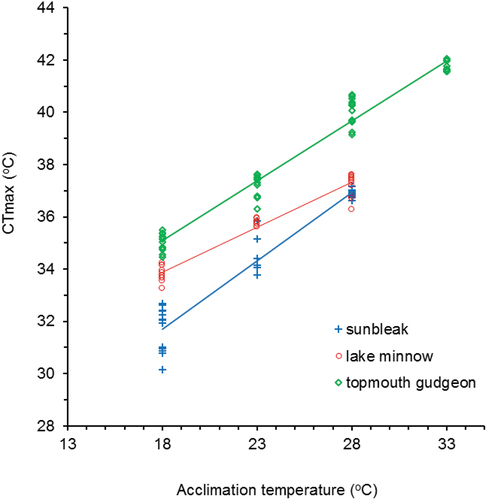
Table II. The upper thermal limit CTmax regression on the temperature of acclimation for the examined fish species. Intercept a and slope b are given with their standard errors. Various letters in superscripts denote groups that statistically differed (the LSD Fisher post hoc test).
Table III. Summary of a Gaussian GLM model of the upper thermal limit (CTmax) as a function of the temperature of acclimation (Tacc.) and fish length (TL) as a covariate trait across the tested fishes.
Discussion
The upper thermal tolerance thresholds in fishes differ at the levels of family, genus and species (Elliott Citation1981; Beitinger et al. Citation2000; Hasnain et al. Citation2013). Physiological plasticity and intra-specific variation in thermal tolerance is well established in the cyprinid group, often considered as a thermophilic or warm-water fish species group (Elliott Citation1981; Wieser Citation1991; Beitinger et al. Citation2000), and represented in our study by a leuciscid, L. delineatus, and a gobionid, P. parva. Many species in the genus Rhynchocypris (family: Leuciscidae), represented here by E. percnurus, are considered as cold-water species, which is related to their postglacial distribution and general ecological performance (Yu et al. Citation2014; Park et al. Citation2019). Our results revealed an unexpected response in CTmax values, which differs between lake minnow and sunbleak depending on acclimation conditions. However, the thermal tolerance of both species coincided at the highest acclimation temperature. It can be assumed that acclimation to warmer water is suboptimal in lake minnow as opposed to sunbleak due to a different physiological efficiency associated with long-term thermal stress. Physiological processes regulating acclimation to warmer temperature involve the metabolic rate, cardiovascular capacity, and oxygen consumption, and are typically species-specific (Fry Citation1971; Wieser Citation1991; Illing et al. Citation2020; McKenzie et al. Citation2021). Nevertheless, the sunbleak had a relatively low thermal tolerance of about 30°C in the case of the lowest acclimation temperatures. In contrast, the CTmax–ILOE difference values varied at the highest acclimation temperature and were higher for E. percnurus. For juvenile lake minnow, an optimum growth temperature (OGT) was determined as 22.0–25.0°C in laboratory conditions, though at a constantly maintained 28.0°C the fish grew slower (Wolnicki et al. Citation2004; Kamiński et al. Citation2011). In the wild, lake minnow suspended feeding activity over 29.0°C in contrast to its most common cohabitant of gibel or crucian carp Carassius carassius (Radtke Citation2011). Field surveys revealed that E. percnurus occupies habitats in which the maximum temperature does not exceed 30.0°C (Kamiński et al. Citation2011). There are no available data regarding the thermal optimum for sunbleak (e.g. OGT), but we can assume that our results correspond with the maximum temperatures occurring currently in small water bodies in central Europe (Kamiński et al. Citation2011; Radtke Citation2011).
The occurrence of sunbleak in Europe, like that of most cyprinid fishes, is a consequence of a postglacial expansion from the Ponto-Caspian refugee region. The region extends from the Volga basin in the East to the Rhine basin in the West, and to the River Rioni drainage basin in the South, as far as 42° latitude (Kottelat & Freyhof Citation2007). The sunbleak is relatively ubiquitous and more widespread than E. percnurus in Europe. Its natural habitats are stagnant isolated water bodies, or those connected to rivers during floods. In recent years, the species is known to be declining throughout its occurrence range (Gozlan et al. Citation2005; Andreou et al. Citation2011), but often spreads to waters connected with carp farms. Simultaneously, L. delineatus is considered as an invasive species in parts of Europe, such as Great Britain or the Asian part of Russia, where it was accidentally introduced with game fish (Zięba et al. Citation2010; Interesova Citation2012; Reshetnikov et al. Citation2017).
In contrast to the sunbleak, our predictions were supported in the case of P. parva. Topmouth gudgeon proved to be highly resistant to elevated temperatures (, ), and therefore can be classified as a warm-water or eurythermal species. Its native range is East Asia, as far to the south as the Zhujiang River in China (Kottelat & Freyhof Citation2007; Baltazar-Soares et al. Citation2020). The species has high reproductive, and exhibits feeding and growth plasticity (Onikura & Nakajima Citation2013; Záhorská et al. Citation2014; Davies & Britton Citation2015; Rolla et al. Citation2020). In Europe, the first specimens of P. parva were introduced to the Danube basin (Romania) in 1961. According to Gozlan (Citation2012), they came from the Cháng Jiang River (River Yangzi system) at Wùhàn, a large town on the east coast of China. The species quickly spread to carp farms and, as a consequence, also to open waters. In Europe, topmouth gudgeon is treated as a highly invasive species, which can affect the structure of natural fish communities (Britton et al. Citation2007; Charrier et al. Citation2016). The species is considered as an important parasite vector, and consequently poses a serious threat to native fish faunas at a global scale (Gozlan Citation2012; Spikmans et al. Citation2020).
Lake minnow occupies water bodies across a large area of the northern hemisphere (Kusznierz et al. Citation2017). According to the latest reports, in the European part of its distribution the most numerous populations currently exist in the Kashubian Lake District, in northern Poland (Wolnicki et al. Citation2022). In other regions of the country, the species has already become extinct or its populations are vestigial. In adjacent countries, e.g. Belarus, Ukraine, and Lithuania, where lake minnow sites were identified in the past, the species most likely survived exclusively at a few sites in the Lithuania (Tsaryk et al. Citation2002; Kaupinis Citation2006; Wolnicki et al. Citation2020). In the context of habitat loss, another symptom of contemporary change that is gaining importance in freshwaters is the appearance of alien fish species (Bernery et al. Citation2022). In Poland, lake minnow populations are often accompanied by alien invasive gibel carp or its hybrids with native crucian carp, less often with sunbleak (Radtke et al. Citation2011; Wolnicki et al. Citation2022). Thus, the emergence of a new invasive species might exert a decisive impact on the survival of E. percnurus or L. delineatus in a changing climate. Recent studies have demonstrated the impact of invasive topmouth gudgeon on native lake minnow populations in their eastern range (Ishiyama et al. Citation2020). Due to a high biological plasticity P. parva is probably the most opportunistic fish among the studied species. Like sunbleak, P. parva exhibits parental care, an additional competitive advantage and valuable trait for an invasive species (Pinder & Gozlan Citation2003). In consequence topmouth gudgeon are able to invade a wider range of different habitats and are currently reported from subtropical waters, such as Vietnam (Karabanov & Kodukhova Citation2013) and alpine streams on the Tibetan Plateau (Jia et al. Citation2019). Simultaneously, as mentioned above, the topmouth gudgeon can be a vector of parasites in newly inhabited areas, and consequently poses a particular threat to lake minnow or sunbleak populations (Andreou et al. Citation2011). Nevertheless, it should be noted that currently both P. parva and L. delineatus owe their success in expanding their range primarily to the aquaculture activities, including pond cultivation and stocking (Pinder & Gozlan Citation2003).
Thermal requirements have, or may have, a substantial impact on the future fate of particular species and the formation of inland fish assemblages in response to increasing water temperature due to climate change (Jeppesen et al. Citation2010; Pletterbauer et al. Citation2015; Lynch et al. Citation2016; Myers et al. Citation2017). The effect of temperature rising is relatively well described for tropical and marine fishes (e.g. Clark et al. Citation2017; Illing et al. Citation2020), but its impact on different life stages and body sizes is ambiguous and varies by species (Ospina & Mora Citation2004; McKenzie et al. Citation2021).
The smallest water bodies are strongly influenced by external environmental factors, which determine their trophic state and biota (Tonn et al. Citation1990; Jackson et al. Citation2001; Scheffer & van Nes Citation2007; Matthews Citation2010). Among other outcomes, water temperature determines both the likelihood of survival of local native fish and the possibility and range expansion of alien species (Comte et al. Citation2013; Rolls et al. Citation2017; Kärcher et al. Citation2019). Thermoregulatory behaviour as a physiological response to thermal stress enables fish to adapt to changing environmental conditions (Crawshaw Citation1977; Goyer et al. Citation2014; Haesemeyer Citation2020). However, in small, isolated water bodies, fish have a limited ability to exhibit thermoregulatory tactics, e.g. through emigration. According to our results risk of physiological disorders and direct fish survival in the face of a temperature increase to values above 30°C will create a significant threat to native populations of sunbleak and lake minnow, but will exert a much lower effect on invasive P. parva. Currently, such “overheating” scenarios for L. delineatus and E. percnurus habitats are likely (Lynch et al. Citation2016; Woolway et al. Citation2020; Dokulil et al. Citation2021). Moreover, rising temperatures as a result of climate change accelerate the eutrophication of shallow lakes in the temperate region (Jeppesen et al. Citation2014), as well as overgrowth, drying, and terrestrialisation of peat bogs and oxbow lakes. Resulting habitat loss might be a more important threat to both species (Sowińska-Świerkosz & Kolejko Citation2019; Wolnicki et al. Citation2022).
In conclusion, our results demonstrated that P. parva have the greatest resistance to temperature increase, while L. delineatus the lowest. The results obtained for the latter species were unexpected as we predicted that this leuciscid fish would be more tolerant of higher temperatures than E. percnurus. The following questions arise: (i) how to protect best the last E. percnurus habitats in Europe against P. parva invasions to avoid impacts already observed in the eastern part of lake minnow occurrence range (Ishiyama et al. Citation2020), and (ii) which species, L. delineatus or P. parva, will be more competitive in extending its range to the north, especially if they occur in sympatry. The present study was restricted to the upper thermal tolerance limits only, but the literature shows that P. parva can also adapt well to low temperatures, as was observed in the Tibetan Plateau (Jia et al. Citation2019). Our field observations indicate that occurrence of sunbleak in small, isolated ponds is often an ephemeral phenomenon. The maximum water temperatures in such water bodies in summer may be close to the upper tolerance threshold of this species, making temperature a key limiting factor. A similar explanation is probable in the case of some lake minnow extinction events. For this reason, the fate of the studied species is dependent on the degree of temperature change in the future. Since juvenile forms have not been tested in this work, further research is needed on the different ontogenic stages of the species shown.
Permissions
The authors obtained appropriate permits to catch fish, including protected species, and are authorized to carry out laboratory tests on fish.
Authors’ contributions
All authors make a significant contribution to the preparation of the manuscript. G.R., J.W., A.K. collected fish in the field, developed the design and performed the experiments. G.R., M.P., J.W., Z.K. analysed the data and drafted the manuscript.
Supplemental Material
Download Text (2.6 KB)Acknowledgements
We warmly thank Arkadiusz Duda, Grzegorz Wiszniewski and Waldemar Święcki from the Stanisław Sakowicz Inland Fisheries Institute in Olsztyn for their help with fish care and assistance during the laboratory tests. We are also grateful to Maciej Kamiński for help in statistical analysis, Łukasz Głowacki for English correction and two anonymous referees for constructive comments.
Disclosure statement
No potential conflict of interest was reported by the author(s).
Supplementary material
Supplemental data for this article can be accessed online at https://doi.org/10.1080/24750263.2022.2148763
Additional information
Funding
References
- Andreou D, Gozlan RE, Stone D, Martin P, Bateman K, Feist SW. 2011. Sphaerothecum destruens pathology in cyprinids. Diseases of Aquatic Organisms 95(2):145–151. DOI: 10.3354/dao02356.
- Baltazar-Soares M, Blanchet S, Cote J, Tarkan AS, Záhorská E, Gozlan RE, Eizaguirre C. 2020. Genomic footprints of a biological invasion: Introduction from Asia and dispersal in Europe of the topmouth gudgeon (Pseudorasbora parva). Molecular Ecology 29(1):71–85. DOI: 10.1111/mec.15313.
- Becker CD, Genoway RG. 1979. Evaluation of the critical thermal maximum for determining thermal tolerance of freshwater fish. Environmental Biology of Fishes 4(3):245–256. DOI: 10.1007/BF00005481.
- Beitinger TL, Bennett WA, McCauley RW. 2000. Temperature tolerances of North American freshwater fishes exposed to dynamic changes in temperature. Environmental Biology of Fishes 58(3):237–275. DOI: 10.1023/A:1007676325825.
- Bernery C, Bellard C, Courchamp F, Brosse S, Gozlan R, Jarić I, Teletchea F, Leroy B. 2022. Freshwater fish invasions: A comprehensive review. hal-03564824.
- Britton JR, Cucherousset J, Davies GD, Godard MJ, Copp GH. 2010. Non-native fishes and climate change: Predicting species responses to warming temperatures in a temperate region. Freshwater Biology 55(5):1130–1141. DOI: 10.1111/j.1365-2427.2010.02396.x.
- Britton JR, Davies GD, Brazier M, Pinder AC. 2007. A case study on the population ecology of a topmouth gudgeon (Pseudorasbora parva) population in the UK and the implications for native fish communities. Aquatic Conservation 17(7):749–759. DOI: 10.1002/aqc.809.
- Carveth CJ, Widmer AM, Bonar SA. 2006. Comparison of upper thermal tolerances of native and nonnative fish species in Arizona. Transactions of the American Fisheries Society 135(6):1433–1440. DOI: 10.1577/T05-025.1.
- Charrier A, Peudpiece M, Lesne M, Daniel P. 2016. First report of the intracellular fish parasite Sphaerothecum destruens associated with the invasive topmouth gudgeon (Pseudorasbora parva) in France. Knowledge & Management of Aquatic Ecosystems 417(417):44. DOI: 10.1051/kmae/2016031.
- Clark TD, Roche DG, Binning SA, Speers-Roesch B, Sundin J. 2017. Maximum thermal limits of coral reef damselfishes are size dependent and resilient to near-future ocean acidification. Journal of Experimental Biology 220(19):3519–3526. DOI: 10.1242/jeb.162529.
- Comte L, Buisson L, Daufresne M, Grenouillet G. 2013. Climate-induced changes in the distribution of freshwater fish: Observed and predicted trends. Freshwater Biology 58(4):625–639. DOI: 10.1111/fwb.12081.
- Crawshaw LI. 1977. Physiological and behavioral reactions of fishes to temperature change. Journal of the Fisheries Board of Canada 34(5):730–734. DOI: 10.1139/f77-113.
- Currie RJ, Bennett WA, Beitinger TL. 1998. Critical thermal minima and maxima of three freshwater game-fish species acclimated to constant temperatures. Environmental Biology of Fishes 51(2):187–200. DOI: 10.1023/A:1007447417546.
- Dalvi RS, Pal AK, Tiwari LR, Das T, Baruah K. 2009. Thermal tolerance and oxygen consumption rates of the catfish Horabagrus brachysoma (Günther) acclimated to different temperatures. Aquaculture 295(1–2):116–119. DOI: 10.1016/j.aquaculture.2009.06.034.
- Davies GD, Britton JR. 2015. Influences of population density, temperature and latitude on the growth of invasive topmouth gudgeon Pseudorasbora parva. Ecology of Freshwater Fish 24(1):91–98. DOI: 10.1111/eff.12128.
- Dell Inc. 2016. Dell Statistica (data analysis software system), version 13. Available: software.dell.com
- Dokulil MT, de Eyto E, Maberly SC, May L, Weyhenmeyer GA, Woolway RI. 2021. Increasing maximum lake surface temperature under climate change. Climatic Change 165(3):1–17. DOI: 10.1007/s10584-021-03085-1.
- Elliott JM. 1981. Some aspects of thermal stress on freshwater teleosts. In: Pickering AD, editor. Stress and Fish. London: Academic Press. pp. 209–245.
- Eme J, Bennett WA. 2009. Critical thermal tolerance polygons of tropical marine fishes from Sulawesi, Indonesia. Journal of Thermal Biology 34(5):220–225. DOI: 10.1016/j.jtherbio.2009.02.005.
- Freyhof J, Brooks E. 2011. European red list of freshwater fishes. Luxembourg: Publications Office of the European Union.
- Fry FEJ. 1971. The effect of environmental factors on the physiology of fish. In: Hoar WS, Randall DJ, editors. Fish Physiology. Vol. 6. London & New York: Academic Press. pp. 1–98.
- Goyer K, Bertolo A, Pépino M, Magnan P. 2014. Effects of lake warming on behavioural thermoregulatory tactics in a cold-water stenothermic fish. PLoS One 9(3):e92514. DOI: 10.1371/journal.pone.0092514.
- Gozlan RE. 2012. Pseudorasbora parva Temminck & Schlegel (topmouth gudgeon). In: Francis RA, editor. A handbook of global freshwater invasive species. Abingdon: Earthscan. pp. 275–285.
- Gozlan R, St-Hilaire S, Feist S, Martin P, Kent ML. 2005. Disease threat to European fish. Nature 435(7045):1046. DOI: 10.1038/4351046a.
- Haesemeyer M. 2020. Thermoregulation in fish. Molecular and Cellular Endocrinology 518:110986. DOI: 10.1016/j.mce.2020.110986.
- Harrod C. 2016. Climate change and freshwater fisheries. In: Craig JF, editor. Freshwater fisheries ecology. Chichester, UK: John Willey & Sons Ltd. pp. 641–694.
- Hasnain SS, Shuter BJ, Minns CK. 2013. Phylogeny influences the relationships linking key ecological thermal metrics for North American freshwater fish species. Canadian Journal of Fisheries and Aquatic Sciences 70(7):964–972. DOI: 10.1139/cjfas-2012-0217.
- Illing B, Downie AT, Beghin M, Rummer JL. 2020. Critical thermal maxima of early life stages of three tropical fishes: Effects of rearing temperature and experimental heating rate. Journal of Thermal Biology 90:102582. DOI: 10.1016/j.jtherbio.2020.102582.
- Interesova EA. 2012. Belica Leucaspius delineatus (Cyprinidae) in waterbodies of southwest Siberia. Journal of Ichthyology 52(5):356–361. DOI: 10.1134/S0032945212020051.
- Ishiyama N, Miura K, Yamanaka S, Negishi JN, Nakamura F. 2020. Contribution of small isolated habitats in creating refuges from biological invasions along a geomorphological gradient of floodplain waterbodies. Journal of Applied Ecology 57(3):548–558. DOI: 10.1111/1365-2664.13546.
- Jackson DA, Peres-Neto PR, Olden JD. 2001. What controls who is where in freshwater fish communities - the roles of biotic, abiotic, and spatial factors. Canadian Journal of Fisheries and Aquatic Sciences 58(1):157–170.
- Jeppesen E, Meerhoff M, Davidson TA, Trolle D, Søndergaard M, Lauridsen TL, Beklioğlu M, Brucet S, Volta P, González-Bergonzoni I, Nielsen A. 2014. Climate change impacts on lakes: An integrated ecological perspective based on a multi-faceted approach, with special focus on shallow lakes. Journal of Limnology 73(s1):88–111. DOI: 10.4081/jlimnol.2014.844.
- Jeppesen E, Meerhoff M, Holmgren K, González-Bergonzoni I, Teixeira-de Mello F, Declerck SA, De Meester L, Søndergaard M, Lauridsen TL, Bjerring R, Conde-Porcuna JM, Mazzeo N, Iglesias C, Reizenstein M, Malmquist HJ, Liu Z, Balayla D, Lazzaro X. 2010. Impacts of climate warming on lake fish community structure and potential effects on ecosystem function. Hydrobiologia 646(1):73–90. DOI: 10.1007/s10750-010-0171-5.
- Jia Y, Kennard MJ, Liu Y, Sui X, Chen Y, Li K, Wang G, Chen Y. 2019. Understanding invasion success of Pseudorasbora parva in the Qinghai-Tibetan Plateau: Insights from life-history and environmental filters. Science of the Total Environment 694:133739. DOI: 10.1016/j.scitotenv.2019.133739.
- Kamiński R, Kamler E, Korwin-Kossakowski M, Myszkowski L, Wolnicki J. 2006. Effects of different incubation temperatures on the yolk-feeding stage of Eupallasella percnurus (Pallas). Journal of Fish Biology 68(4):1077–1090. DOI: 10.1111/j.0022-1112.2006.01008.x.
- Kamiński R, Wolnicki J, Sikorska J. 2011. Physical and chemical water properties in water bodies inhabited by the endangered lake minnow, Eupallasella percnurus (Pall.), in central Poland. Fisheries & Aquatic Life 19(3):153–159.
- Karabanov DP, Kodukhova YV. 2013. Stone moroco Pseudorasbora parva (Cyprinidae): New species in the ichthyofauna of Vietnam. Journal of Ichthyology 53(3):235–239. DOI: 10.1134/S0032945213020057.
- Kärcher O, Hering D, Frank K, Markovic D. 2019. Freshwater species distributions along thermal gradients. Ecology and Evolution 9(1):111–124. DOI: 10.1002/ece3.4659.
- Kaupinis A. 2006. Lake minnow (Eupallasella percnurus (Pallas, 1814)) in Lithuanian inland waters. Acta Zoologica Lituanica 16(4):309–311. DOI: 10.1080/13921657.2006.10512746.
- Kottelat M, Freyhof J. 2007. Handbook of European freshwater fishes. Cornol, Switzerland: Publications Kottelat.
- Kusznierz J, Paśko Ł, Maślak R, Pietras-Lebioda A, Borczyk B, Tagayev D, Sergiel A, Wolnicki J. 2017. Broad-scale morphometric diversity in the lake minnow Eupallasella percnurus (Cyprinidae: Pisces). Annales Zoologici Fennici 54(5–6):357–371. DOI: 10.5735/086.054.0507.
- Lynch AJ, Myers BJ, Chu C, Eby LA, Falke JA, Kovach RP, Krabbenhoft TJ, Kwak TJ, Lyons J, Paukert CP, Whitney JE. 2016. Climate change effects on North American inland fish populations and assemblages. Fisheries 41(7):346–361. DOI: 10.1080/03632415.2016.1186016.
- Matthews J. 2010. Anthropogenic climate change impacts on ponds: A thermal mass perspective. BioRisk 5:193–209. DOi: 10.3897/biorisk.5.849.
- McKenzie DJ, Zhang Y, Eliason EJ, Schulte PM, Claireaux G, Blasco FR, Nati JJH, Farrell AP. 2021. Intraspecific variation in tolerance of warming in fishes. Journal of Fish Biology 98(6):1536–1555. DOI: 10.1111/jfb.14620.
- Mora C, Maya MF. 2006. Effect of the rate of temperature increase of the dynamic method on the heat tolerance of fishes. Journal of Thermal Biology 31(4):337–341. DOI: 10.1016/j.jtherbio.2006.01.005.
- Myers BJ, Lynch AJ, Bunnell DB, Chu C, Falke JA, Kovach RP, Krabbenhoft TJ, Kwak TJ, Paukert CP. 2017. Global synthesis of the documented and projected effects of climate change on inland fishes. Reviews in Fish Biology and Fisheries 27(2):339–361. DOI: 10.1007/s11160-017-9476-z.
- Noyola J, Mascaro M, Díaz F, Re AD, Sánchez-Zamora A, Caamal-Monsreal C, Rosas C. 2015. Thermal biology of prey (Melongena Corona bispinosa, Strombus pugilis, Callinectes similis, Libinia dubia) and predators (Ocyurus chrysurus, Centropomus undecimalis) of Octopus maya from the Yucatan Peninsula. Journal of Thermal Biology 53:151–161. DOI: 10.1016/j.jtherbio.2015.11.001.
- Onikura N, Nakajima J. 2013. Age, growth and habitat use of the topmouth gudgeon, Pseudorasbora parva in irrigation ditches on northwestern Kyushu Island, Japan. Journal of Applied Ichthyology 29(1):186–192.
- Ospina AF, Mora C. 2004. Effect of body size on reef fish tolerance to extreme low and high temperatures. Environmental Biology of Fishes 70(4):339–343. DOI: 10.1023/B:EBFI.0000035429.39129.34.
- Park J, Kim Y, Xi H. 2019. The complete mitochondrial genome sequence of Chinese minnow in Korea, Rhynchocypris oxycephalus (Sauvage and Dabry de Thiersant, 1874). Mitochondrial DNA Part B 4(1):662–663. DOI: 10.1080/23802359.2019.1572472.
- Pinder AC, Gozlan RE. 2003. Sunbleak and topmouth gudgeon: Two new additions to Britain’s freshwater fishes. British Wildlife 15(2):77–83.
- Pletterbauer F, Melcher AH, Ferreira T, Schmutz S. 2015. Impact of climate change on the structure of fish assemblages in European rivers. Hydrobiologia 744(1):235–254. DOI: 10.1007/s10750-014-2079-y.
- Radtke G. 2011. Comparison of seasonal activity of the lake minnow, Eupallasella percnurus (Pall.), and crucian carp, Carassius carassius (L.), in small water bodies in northern Poland. Archives of Polish Fisheries 19(3):175–182. DOI: 10.2478/v10086-011-0022-7.
- Radtke G, Wolnicki J, Kamiński R. 2011. Occurrence, threats and protection of the endangered lake minnow, Eupallasella percnurus (Pall.), in Pomorskie Voivodeship in Poland. Archives of Polish Fisheries 19(3):183–193. DOI: 10.2478/v10086-011-0023-6.
- Rajaguru S. 2002. Critical thermal maximum of seven estuarine fishes. Journal of Thermal Biology 27(2):125–128. DOI: 10.1016/S0306-4565(01)00026-2.
- R Development Core Team. 2021. R: A language and environment for statistical computing. Available: https://www.r-project.org.
- Reshetnikov AN, Golubtsov AS, Zhuravlev VB, Lomakin SL, Rezvyi AS. 2017. Range expansion of rotan Perccottus glenii, sunbleak Leucaspius delineatus, and bleak Alburnus alburnus in the Ob River basin. Contemporary Problems of Ecology 10(6):612–620. DOI: 10.1134/S1995425517060105.
- Rolla M, Consuegra S, Garcia de Leaniz C. 2020. Trophic plasticity of the highly invasive topmouth gudgeon (Pseudorasbora parva) inferred from stable isotope analysis. Frontiers in Ecology and Evolution 8:212. DOI: 10.3389/fevo.2020.00212.
- Rolls RJ, Hayden B, Kahilainen KK. 2017. Conceptualising the interactive effects of climate change and biological invasions on subarctic freshwater fish. Ecology and Evolution 7(12):4109–4128. DOI: 10.1002/ece3.2982.
- Scheffer M, van Nes EH. 2007. Shallow lakes theory revisited: Various alternative regimes driven by climate, nutrients, depth and lake size. Hydrobiologia 584(1):455–466. DOI: 10.1007/s10750-007-0616-7.
- Sowińska-Świerkosz B, Kolejko M. 2019. Extinction risk to lake minnow (Eupallasella percnurus) due to habitat loss: Eastern Poland case study. Environmental Monitoring and Assessment 191(9):1–17. DOI: 10.1007/s10661-019-7731-6.
- Spikmans F, Lemmers P, van Haren E, Kappen F, Blaakmeer A, van der Velde G, van Langevelde F, Leuven RSEW, van Alen TA. 2020. Impact of the invasive alien topmouth gudgeon (Pseudorasbora parva) and its associated parasite Sphaerothecum destruens on native fish species. Biological Invasions 22(2):587–601. DOI: 10.1007/s10530-019-02114-6.
- Tonn WM, Magnuson JJ, Rask M, Toivonen J. 1990. Intercontinental comparison of small-lake fish assemblages: The balance between local and regional processes. The American Naturalist 136(3):345–375. DOI: 10.1086/285102.
- Tsaryk J, Gorban I, Holovachov O, Shydlovskyy I, Gorban L, Dykyy I, Lesnik V, Matejchyk V, Pudlyk J, Senyk M. 2002. Ecological factors influencing biodiversity preservation in the Shatsk National Natural Park. Acta Agrophysica 67:275–285.
- Wieser W. 1991. Physiological energetics and ecophysiology. In: Winfield IJ, Nelson JS, editors. Cyprinid fishes: Systematics, biology and exploitation. London: Chapman & Hall. pp. 426–455.
- Witkowski A, Grabowska J. 2012. The non-indigenous freshwater fishes of Poland: Threats to the native ichthyofauna and consequences for the fishery: A review. Acta Ichthyologica et Piscatoria 42(2):77–87. DOI: 10.3750/AIP2011.42.2.01.
- Wolnicki J, Kamiński R, Korwin-Kossakowski M, Kusznierz J, Myszkowski L. 2004. The influence of water temperature on laboratory-reared lake minnow Eupallasella perenurus (Pallas) larvae and juveniles. Archives of Polish Fisheries 12(1):61–69.
- Wolnicki J, Rakauskas V, Juzumas L. 2020. Występowanie strzebli błotnej, Eupallasella percnurus (Pall.), w wodach południowej Litwy – Obecny stan wiedzy. [Occurrence of lake minnow, Eupallasella percnurus (Pall.), in the waters of southern Lithuania – Present state of knowledge]. Komunikaty Rybackie 3:24–26.
- Wolnicki J, Sikorska J, Radtke G. 2022. Protection of the endangered fish lake minnow, Eupallasella percnurus (Pallas, 1814), in the Natura 2000 network in Poland – Present state and perspectives. Fisheries & Aquatic Life 30(2):125–137. DOI: 10.2478/aopf-2022-0012.
- Woolway RI, Kraemer BM, Lenters JD, Merchant CM, O’Reilly CM, Sharma S. 2020. Global lake responses to climate change. Nature Reviews Earth & Environment 1(8):388–403. DOI: 10.1038/s43017-020-0067-5.
- Yu D, Chen M, Tang Q, Li X, Liu H. 2014. Geological events and Pliocene climate fluctuations explain the phylogeographical pattern of the cold water fish Rhynchocypris oxycephalus (Cypriniformes: Cyprinidae) in China. BMC Evolutionary Biology 14(1):1–12. DOI: 10.1186/s12862-014-0225-9.
- Záhorská E, Kováč V, Švolíková K, Kapusta A. 2014. Reproductive parameters of topmouth gudgeon (Pseudorasbora parva) from a heated Lake Licheńskie (Poland). Central European Journal of Biology 9(2):212–219.
- Zar JH. 2010. Biostatistical analysis. 5th ed. Upper Saddle River, NY, USA: Prentice-Hall/Pearson.
- Zięba G, Copp GH, Davies GD, Stebbing P, Wesley KJ, Britton JR. 2010. Recent releases and dispersal of non-native fishes in England and Wales, with emphasis on sunbleak Leucaspius delineatus (Heckel, 1843). Aquatic Invasions 5(2):155–161. DOI: 10.3391/ai.2010.5.2.04.