Abstract
The effects of recent climate change on the phenology of avian breeding and migration, geographical range shifts, and decreasing migration distances are well documented. Only a few studies have focused on the long-term influence of these changes on the body condition of migrants, which is important as it is likely to affect the fitness of the whole population. Eurasian blackcaps have adapted their migratory behaviour to changing ecological factors. These changes seem to favour the species and its migratory populations have increased over the last decades in Europe. In the present study, we analysed the body condition index of juvenile blackcaps at their stop-over site in the southern Baltic region during autumn migration from 1967 to 2006. This index was distinctly higher since the 1990s than in the middle of the 1960s, when the number of migrating blackcaps was at least twofold lower. Moreover, there was a reduction in recapture rates of blackcaps from 14% to 6% during these periods which may imply that warblers were staying for a shorter time at the stop-over site as their body condition was higher. We hypothesized that higher berry crop availability resulted in the higher condition of migrating blackcaps. Body condition index also reflecting fuel load in migrants was higher in long-distance blackcaps compared to the short-distance ones. As the former are time-minimizing migrants, their higher body condition/fuel load may allow them to migrate faster to wintering grounds in sub-Saharan Africa.
Introduction
Climate is among the major factors determining key biological processes and ultimately influencing the abundance and distribution of plant and animal species. In birds, recent climate changes induced phenological changes in the timing of seasonal activities such as breeding and migration (Tryjanowski et al. Citation2002; Cotton Citation2003; Jenni & Kéry Citation2003; Marra et al. Citation2005; Hüppop & Winkel Citation2006; Tøttrup et al. Citation2006; Thorup et al. Citation2007; Remisiewicz & Underhill Citation2022), caused geographical range shifts, like the expansion of some species northwards (in Europe: Brommer et al. Citation2012), and decreasing migration distances (e.g., Visser et al. Citation2009; Ambrosini et al. Citation2016). Climate-change-induced shifts in the timing of vegetation development (Menzel & Fabian Citation1999), and consequently of invertebrate availability during the breeding season, lead to mistimed reproduction and/or influenced growth in birds (van Gils et al. Citation2016). Finally, elevated temperatures, an effect of global warming, may have a profound impact on birds due to their predominantly diurnal habits and generally small body sizes (McKechnie & Wolf Citation2010). The fitness costs of global climate change may be considerable and may result in changing avian population demographics (Both et al. Citation2006; du Plessis et al. Citation2012; van Gils et al. Citation2016). We have learnt how these global changes influence migratory preparations and the behaviour of migrants – not only adapting migration phenology but overall migratory/breeding schedules (Gill et al. Citation2014). Migrants adjust migration and migratory speed also in response to environmental conditions en route (Tøttrup et al. Citation2008), and the variation in food abundance in the staging areas may affect their fitness (Rakhimberdiev et al. Citation2018). Our knowledge of the influence of climate change on avian fitness and/or body condition over the last decades is growing (du Plessis et al. Citation2012; van Gils et al. Citation2016; Gardner et al. Citation2018; Rakhimberdiev et al. Citation2018). Yet so far only a few studies investigated changes over the last decades in migrants’ body condition at migratory stopover sites (e.g., Rakhimberdiev et al. Citation2018).
In the present study, we analysed the body condition of autumn migrating Eurasian blackcaps Sylvia atricapilla at their stop-over site in the southern Baltic region over 4 decades, from 1967 to 2006. The Eurasian blackcap is a highly adaptive species (Pulido & Berthold Citation2004) and may respond to changing ecological factors by changes in its migratory behaviour, e.g., establishing a novel wintering ground (Berthold & Terrill Citation1988; Busse Citation1992; Rolshausen et al. Citation2010; Kopiec & Ożarowska Citation2012) and/or shifting its migration phenology (e.g., Ożarowska & Zaniewicz Citation2015; Ożarowska et al. Citation2018). Current climate change seems to favour this species and its migratory populations have increased over the last decades (Berthold et al. Citation1999; Gyurácz & Bánhidi Citation2008; Zaniewicz & Busse Citation2010; Ożarowska et al. Citation2016; Gyurácz et al. Citation2021). So far, we have learnt that the Blackcap populations adapt their migratory behaviour to environmental changes, yet we do not know whether these changes also affect the body condition of migrating individuals. Such studies would be valuable as the body condition of individuals affects the fitness of the whole population, and when based on the species that exhibits enormous variation in its migratory behaviour, like the Eurasian blackcap (e.g., Delmore et al. Citation2020a, Citation2020b), would bring some more information on the climate change effect on short- and long-distance migrants.
The Eurasian blackcap populations either cover shorter distances and winter in the southern part of Europe or North Africa (short-distance migrants) or travel as far as sub-Saharan Africa (long-distance migrants) (Bakken et al. Citation2006; Bønløkke et al. Citation2006; Fransson & Hall-Karlsson Citation2008). We may therefore expect the difference in the body condition of migrants due to the distance they cover. The body condition of migrants reflects their actual physical condition, but most of all it shows their accumulated energy reserves enabling them to cover the distance between the stopover sites and/or cross large ecological barriers, like mountain ranges, seas (Baltic Sea, Mediterranean Sea), or deserts (Sahara).
During over 40 years rapidly growing numbers of juvenile blackcaps migrating through the southern Baltic region in autumn were paralleled by a remarkable decrease in wing length (Ożarowska et al. Citation2016). We suggest that these trends are due to the changing population composition of migrating birds and a distinct increase in the number of short-winged blackcaps, migrating short distances and not crossing the Sahara Desert (op cit.). Both short- and long-distance migrants probably benefit from improved conditions at their breeding grounds, but the shorter migration route and favourable conditions at wintering sites north of the Sahara may favour short-distance migrants over the longer-distance and longer-winged blackcaps (op. cit.). The long-winged blackcaps (long-distance migrants) compared to short-winged may be more vulnerable to environmental changes as they may be constrained in their response to climate change by rigid endogenous control and inherited circannual programs (Both & Visser Citation2001; Cotton Citation2003; Pulido & Widmer Citation2005). Still, there are examples suggesting that longer-distance migrants, like black-tailed godwits Limosa limosa, are not always more susceptible to climate change (Gill et al. Citation2014). In our previous studies, we also reported that long-distance blackcaps, like short-distance conspecifics, responded to changing climatic conditions and adapted their migration phenology, yet their response was delayed and/or less pronounced (Ożarowska & Zaniewicz Citation2015; Ożarowska et al. Citation2018). Considering all these facts we may also expect the difference in the body condition between these two groups. In this study, we aimed to determine if there were temporal trends in the body condition of migrating blackcaps during the long period covering some years preceding the time of documented increase in global temperature as well as its rapid increase since 1985 (Hansen et al. Citation2006), and whether there was any difference between the body condition of short- and long-winged blackcaps over this period.
Materials and methods
Field methods
Eurasian blackcaps migrating in the southern Baltic region in autumn, originate from different populations (Bakken et al. Citation2006; Bønløkke et al. Citation2006; Fransson & Hall-Karlsson Citation2008). These are local breeding birds and transients from Scandinavia (Norway, Sweden), and regions located to the east and north-east of Poland (Lithuania, Latvia, Estonia, Russia) (Bakken et al. Citation2006; Bønløkke et al. Citation2006; Fransson & Hall-Karlsson Citation2008). Therefore, we focused on a single station to sample a similar group of migrants every year. We analysed the data collected over 40 years (1967–2006) on migrating juvenile, i.e., first-year, blackcaps captured at Bukowo-Kopań bird ringing station, Poland (54°20′17.66′′N; 16°14′43.09′′E) on the southern coast of the Baltic Sea. We applied the standard Operation Baltic and SE European Bird Migration Network methodology, i.e., constant mist-netting from the mid of August to the end of November (Busse & Meissner Citation2015). This period covers 98.5% of autumn migration of the Eurasian blackcap in the region (Nowakowski Citation1999). During the entire migration season, nets were open 24 hours a day and were checked every hour from dawn to about one hour after dusk. Over 40 years the distribution of nets relative to habitats was stable and each year covered mature forests, young forest stands, shrubs, reed beds, and open habitats. Birds were sexed and aged according to the plumage characteristics following Svensson (Citation1992). First-year male and female blackcaps are about the same size (Holloway & Edwards Citation1989; Shirihai et al. Citation2001). Biometric measurements included wing length measured as a maximum chord with an accuracy of 1 mm using a ruler, fat score according to a nine-score scale (Busse & Meissner Citation2015), and weight with an accuracy of 0.1 g in 1967–1969 and since 1999 onwards, and 0.5 g in 1970 and 1984–1998.
Only full-grown juvenile individuals were measured, so the primary feathers of captured birds were carefully checked for any basal sheaths to ensure feather growth was complete. The measurements were taken by experienced ringers; each year the accuracy and repeatability of the measurements taken by different ringers were checked as described by Busse and Meissner (Citation2015) to ensure comparability across years.
Data analysis
Data were collected from 1967 to 2006. When analysing the body condition of autumn migrating juvenile blackcaps, we included data on individuals captured for the first time in the season with a complete set of biometric measurements. Since in 1971–1983 birds were not weighed, so we excluded those years from the analyses. The remaining data were grouped into six 4-year periods and the final period covered only 3 years: 2004–2006. Data were grouped due to the very low number of blackcaps captured in the 1980s. The median date of the Eurasian blackcap passage at Bukowo-Kopań bird ringing station was 16 September in 1967–2006 (Zaniewicz Citation2014). To exclude data on local birds a period from 1 September to 17 November was defined for data analysis. The beginning of this period was based on the preliminary analysis showing a clear increase in fat score of captured birds and suggesting the active passage of transient birds (authors’ unpublished data). The fat reserves of migrating blackcaps captured at the studied site were moderate, and it is typical for this region and stage of migration (Ożarowska Citation2015). Finally, we analysed data on 5,160 juvenile blackcaps, and out of this number, 330 individuals were captured more than once in the season (). The number of blackcaps varied in subsequent seasons. It was the lowest at the beginning of the 1980s, and then it distinctly increased. This increase was particularly clear in short-winged individuals ().
Table I. Number of short- and long-winged blackcaps captured and recaptured within the same season (numbers given in brackets) at Bukowo-Kopań bird ringing station, Poland during autumn migration on the southern coast of the Baltic Sea.
In migratory species, wing length reflects their flight performance and increases with increasing migration distance, which is also the case in various blackcap populations (Lo Valvo et al. Citation1988; Fiedler Citation2005; Ożarowska et al. Citation2021). As we aimed to check the possible difference in the body condition of migrants due to the distance they cover, then we used wing length as a proxy for the migratory behaviour. Birds were grouped as short-winged 70–74 mm (n = 1891), medium-winged 75–76 mm (n = 1999), or long-winged individuals 77–81 mm (n = 1270) based on lower and upper quartiles in the wing length distribution of captured blackcaps. Like in previous studies (Ożarowska & Zaniewicz Citation2015; Ożarowska et al. Citation2018), we assumed shorter-winged birds represent short-distance migrants and longer-winged individuals represent long-distance migrants (cf. Fiedler Citation2005; Bengtsson et al. Citation2009; Ożarowska et al. Citation2021) and focused on these two groups exclusively ().
Because body mass is size-dependent, we used residual body mass from regression of body mass of individuals a with fat score equal to zero (no subcutaneous fat deposits visible) captured for the first time in the season on wing length as an index of body condition at the individual level (Labocha & Hayes Citation2012; Stępniewska et al. Citation2020). In our data set, the relationship between body mass and wing length in blackcaps with no fat reserves was as follows: body mass = 9.97 + 0.10* wing length (F1,517 =11.26, p < 0.001; R2= 0.15). In this approach, an individual with a positive residual body mass was considered in better condition than the individual with a negative value.
To determine the relationship between body condition index of migrating blackcaps and factors that might have influenced this parameter we used a generalised linear model (GLM) (McCullagh & Nelder Citation1989) with an identity link function and normal error distribution with body condition index as the response variable, period, wing-length groups (short- vs. long-winged blackcaps), sex, and the day in the season as explanatory variables. The two-way interactions between categorical variables were included in the model. A criterion based on the Wald statistic (χ2) was applied to determine which variables had a significant influence on body condition index. In this paper, we fitted the full models only to learn the influence of all factors considered. For simplicity, non-significant interactions between categorical variables were not reported.
In this study, we analysed body condition index of an individual at its first capture. Still, we are aware that its actual arrival date at the study site was unknown. As the variation in body condition of migrants may be due to the time they spent refuelling at a given stopover site, we also analysed the recapture rate of juvenile blackcaps and their minimum stopover duration during the studied periods. Due to the low number of recaptures in some periods (), we only included data from 1967 to 1979 and three periods from 1996 onwards. The recapture rate was the percentage of individuals ringed and recaptured later at Bukowo-Kopań station during the same season (e.g., Stępniewska et al. Citation2020). We used a generalised linear model with a logit link function and binomial error distribution to test if the recapture rate of juvenile blackcaps (response variable) differed among the studied periods, groups of blackcaps that differed by wing-length (short- vs. long-winged blackcaps) and sex categories (males and females). The two-way interactions between categorical variables were included. Finally, we analysed minimum stopover duration estimated as the difference between the date of the last capture of an individual, and the date it was captured for the first time at the study site in a particular season, i.e., it was 2 days for an individual that was recaptured on the next day of its first capture. This index is commonly used to estimate the minimum stopover duration of passerine and non-passerine species (Kaiser Citation1999; Minias et al. Citation2010; Bayly et al. Citation2012; Stępniewska et al. Citation2020). We used a generalised linear model with a log link function and normal error distribution to test if minimum stopover duration (response variable) differed in 1967–1979 and three periods since 1996 onwards, among groups of blackcaps that differed by wing-length (short- vs. long-winged blackcaps), and whether such factors as body condition at the first and last capture, and the day in the season might have influenced this parameter. The two-way interactions between categorical variables were included in this model. The analyses were performed using the STATISTICA 12 software (StatSoft Citation2014).
Results
Body condition indices of migrating juvenile blackcaps significantly increased over 40 years of the study (; ). Long-winged blackcaps had higher body condition indices when compared to short-winged individuals in all study periods (GLM, Wald χ2 = 15.63, p < 0.001; ; sample sizes are given in ), and females showed higher body condition indices than males (GLM, Wald χ2 = 5.37, p = 0.020; ; nF = 1718, nM = 2078). Neither the day in the season nor all analysed interactions influenced body condition indices of juvenile blackcaps migrating in autumn in the southern Baltic region ().
Figure 1. Long-term changes in body condition indices of long- and short-winged juvenile blackcaps migrating in autumn in the southern Baltic region as shown by the GLM (vertical lines: 95% confidence intervals). No data available for the period 1971–1983.
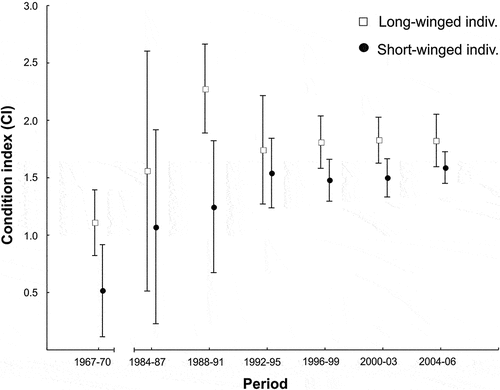
Table II. Effects of period, wing-length group, and sex on body condition index of juvenile blackcaps during autumn migration in the southern Baltic region according to the GLM model. For simplicity, non-significant interactions between categorised variables were not shown. The estimate is set to zero for the baseline category of the first period, i.e., 1967–1970, group of short-winged birds and female blackcaps. Sample sizes are given in .
The recapture rate of juvenile blackcaps at the study site did not differ between male and female blackcaps (GLM, Wald χ2 = 0.84, p = 0.360; ; nF = 1718, nM = 2078) or long- and short-winged individuals (GLM, Wald χ2 = 1.85, p = 0.174; ; sample sizes are given in ). The recapture rate was low (), and only 6% of birds recaptured over all study years. This proportion varied significantly between the beginning and the end of the studies, i.e., 14% in 1967–1970 vs 6% in 2004–2006 (GLM, Wald χ2 = 4.97, p = 0.026; ; ), yet it tended to be lower already since 1996–1999.
Figure 2. Recapture rates of juvenile blackcaps migrating in autumn in the southern Baltic region as shown by the GLM (vertical lines: 95% confidence intervals). Due to the low number of recaptures in some periods, data from 1967–1979 and three periods from 1996 onwards were analysed.
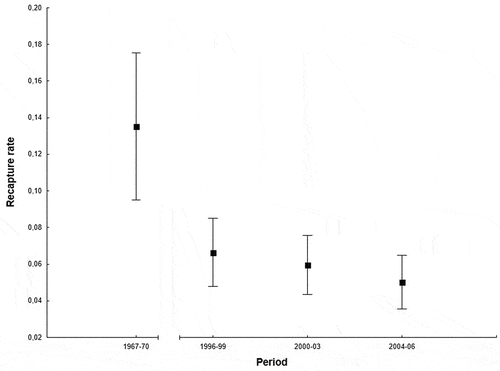
Table III. Effects of period, wing-length group, and sex on recapture rate of juvenile blackcaps during autumn migration in the southern Baltic region according to the GLM model. For simplicity, non-significant interactions between categorised variables were not shown. The estimate is set to zero for the baseline category of the first period, i.e., 1967–1970, group of short-winged birds and female blackcaps. Sample sizes are given in .
Most recaptured blackcaps (56%) stayed at the study site for 1 and 2 days (39% and 17%, respectively). Minimum stopover duration did not differ between long- and short-winged blackcaps or four analysed periods (). Only body condition of an individual at its first capture had a significant influence on its minimum stopover duration, i.e., the lower its body condition index, the longer that individual stayed at the study site (GLM, Wald χ2 = 4.53, p = 0.033; ; sample sizes are given in ), while the day when the individual was captured in the season or its body condition at the last capture did not affect this parameter ().
Table IV. Effects of period, wing-length group, sex, day in the season, body condition of an individual at the first and at the last capture on minimum stopover duration of juvenile blackcaps during autumn migration in the southern Baltic region according to the GLM model. For simplicity, non-significant interactions between categorised variables were not shown. The estimate is set to zero for the baseline category of the first period, i.e., 1967–1970, group of short-winged birds and female blackcaps. Sample sizes are given in .
Discussion
The body condition of blackcaps migrating in the southern Baltic region was distinctly higher since the 1990s compared to the second half of the 1960s, i.e., when the number of these migrants was at least twofold lower, and then continued to decline for more than a decade (Ożarowska et al. Citation2016). There was no change in the stopover length at the study site between 1967 and 1970 and since 1996 onwards, and most birds stayed there for only 1 or 2 days. Yet there was a clear reduction in recapture rates of blackcaps from 14% in the middle of the 1960s to 6% since the 1990s. This reduction may imply that birds were staying for a shorter time at Bukowo-Kopań as their body condition was higher, and therefore were not recaptured. The analysis of recaptures also showed that the lower the initial body condition of an individual the longer that bird stayed at the stopover site. And indeed, the stopover duration of recaptured blackcaps did not change over 40 years of the study. Birds arriving with low energetic reserves need more time for refuelling to be able to continue their migration and stay longer than those that arrived at the stopover site with larger reserves (e.g., Biebach et al. Citation1986; Ellegren Citation1991).
Blackcaps advanced autumn migration start (Ożarowska & Zaniewicz Citation2015), and we hypothesize that this allowed them to match its phenology with the food resources availability during the pre-migratory fattening and/or en route. It is plausible as this species and the closely related Garden Warbler Sylvia borin show a distinct seasonal shift in food selection at this time of the year (Bairlein Citation1998, Citation2002; Eggers Citation2000). Fruits compose about 90% of the juvenile blackcap diet during both pre-migration and autumn migration periods (Eggers Citation2000). Such a diet is advantageous as fruits have a high content of fatty acids necessary for building up lipid depots, which are the main energy source for long-distance flights of migrating birds (Bairlein Citation2002). In addition, flavonoids may play a beneficial role in immunology for avian wild species (Catoni et al. Citation2008) and may protect against the potentially damaging effects of oxidative stress caused by long-distance flight (Bolser et al. Citation2013). Moreover, fruits are conspicuous and often abundant locally, then birds may minimise the time spent searching for food. That is particularly important for juvenile individuals as they lack experience with environmental conditions en route, including food resources and habitat structures (Eggers Citation2000).
Several studies have demonstrated a lengthening of the plant growing season and significant advancements in spring phenophases of plants, i.e., the timing of recurring life history events of species, across the northern hemisphere (Menzel et al. Citation2006; Schwartz et al. Citation2006; Schleip et al. Citation2009; Szabó et al. Citation2016). This also applies to different fruiting shrubs, like the black elder Sambucus nigra. This species is widespread all over Europe (Atkinson & Atkinson Citation2002) and its berries compose an important part of the diet of migrating blackcaps (Eggers Citation2000; Bairlein Citation2002). Its flowering onset in Europe has advanced on average 2.8 days per decade over the 1959–1996 period (Menzel Citation2000), and consequently the first ripe fruits are available earlier (Chmielewski et al. Citation2005). Most shifts in the timing of vegetation development occurred in the late 1980s and early 1990s (Menzel Citation2000). This coincides with the shift in autumn migration phenology of blackcaps towards earlier dates (i.e., 2.2 and 1.2 days per decade in the short- and long-winged blackcaps, respectively; Ożarowska & Zaniewicz Citation2015), the significant increase in their number (Ożarowska et al. Citation2016), and the distinct increase in blackcaps’ body condition index reported in our study since the 1990s. Migration phenology of short- and longed-winged blackcaps is complex (Zaniewicz Citation2014) and a future study could measure if these migrants match their migration phenology with the black elder berries availability. Diet, abundance, and the predictability of food resources directly influence fuel deposition rates in migratory species (Schaub & Jenni Citation2000), then food resources availability during the pre-migratory period and en route is of the utmost importance. The black elder has become also more abundant in Europe and the reason is most probably climate change. There are also other factors, like an increase in nitrogen availability to this nitrophilous species resulting from the development of industry, the manufacture of agricultural fertilisers, and the growth of the urban population in recent decades that favoured this species (Tamis et al. Citation2005; Hédl et al. Citation2010; Heinrichs et al. Citation2012; Buriánek et al. Citation2013). All these changes could result in increasing food availability for blackcaps migrating in Europe, either short- or long-distance individuals, and their higher body condition.
Body condition index in migrants reflects their fuel loads, i.e., fat stores and wet protein, which in most passerine migrants may reach 10–30% of their lean body mass at departure (e.g., Alerstam & Lindström Citation1990; Schaub et al. Citation2008). Body condition indices were higher in long-winged individuals (long-distance migrants) compared to short-winged blackcaps (short-distance migrants). Higher body condition of long-distance blackcaps may allow them to migrate faster. These blackcaps winter in sub-Saharan Africa and are most probably under selective pressure to minimize the time spent on migration (cf. time minimization strategy in Hedenström & Alerstam Citation1997). Indeed, blackcaps arrive in the eastern Mediterranean with high fat stores (Ożarowska Citation2015), which are much larger than in spring (Zduniak et al. Citation2013; Wojciechowski et al. Citation2014) and they stopover in this region only for 1–3 days (Yosef & Wineman Citation2010). High energy reserves and short stopovers governing their fast tempo of migration while heading to the wintering grounds maximize the probability of encountering rich stopover sites in the Sahel at the end of the rainy season before continuing migration further south (Schaub & Jenni Citation2001; Jenni & Kéry Citation2003; Gordo Citation2007).
In the present study, we found that short- and long-winged females had higher body condition than males at the stopover site in the southern Baltic region but did not differ in the recapture rate or stopover duration. This may indicate that they are differential distance migrants. A significantly larger proportion of females in blackcaps wintering in Africa (Catry et al. Citation2006) may support these findings. In Hungary, during the post-fledging period, males had lower body mass than females but there was no difference in fat scores and average stopover length between both sexes. Authors hypothesized that males and females might have been avoiding intraspecific resource competition by foraging in different microhabitats at stopover sites (Gyurácz et al. Citation2017). All these facts indicate differential migratory behaviour of males and females at least in some blackcap populations (Catry et al. Citation2006; Gyurácz et al. Citation2017; this study). Still, further studies are needed as juvenile blackcaps are size-monomorphic (Holloway & Edwards Citation1989; Shirihai et al. Citation2001; Pérez-Tris & Tellería Citation2002; Ożarowska et al. Citation2021) and at European wintering grounds, there is no geographical segregation between sexes (Pérez-Tris & Tellería Citation2002; Catry et al. Citation2006).
Conclusions
The advancement in autumn migration phenology that allowed Eurasian blackcaps matching with seasonal berry crop availability during the pre-migratory period and en route, as well as the higher abundance of fruiting shrubs due to climate change in recent decades, may result in the higher body condition of autumn migrating blackcaps, either short- or long-distance individuals, and presumably higher survival rate. Coupled with earlier spring arrival allowing for higher breeding success, the number of migratory populations increased significantly since the end of the 1980s and the beginning of the 1990s. It seems that both, short- and long-distance migrating blackcaps take advantage of changing environmental conditions and, so far, their adaptive response seems sufficient to match these changes.
Compliance with ethical standards
All applicable international, national and/or institutional guidelines for the care and use of animals were followed. Bird ringing and data collection were conducted by experienced, professional ringers having valid licenses and permissions of the National Ringing Centres in Poland. All the fieldwork was done according to the ethical standards recommended by this institution.
Geolocation information
Central Europe.
Acknowledgments
We are grateful to Prof. Pshem Busse and dr. Magdalena Remisiewicz, retired and present Heads of the Operation Baltic team for assistance, and to all ringers and volunteers for their help during field work. We are also grateful to anonymous reviewers and the editors for their detailed and thoughtful critiques of the earlier draft of the manuscript, which has been greatly improved.
Data availability statement
The data analysed in this study are available from the corresponding author upon reasonable request.
Disclosure statement
No potential conflict of interest was reported by the author(s).
Additional information
Funding
References
- Alerstam T, Lindström A. 1990. Optimal bird migration: The relative importance of time, energy, and safety. In: Gwinner E, editor Bird migration: Physiology and ecophysiology. Berlin Heidelberg New York: Springer. pp. 331–351.
- Ambrosini R, Cuervo JJ, du Feu C, Fiedler W, Musitelli F, Rubolini D, Sicurella B, Spina F, Saino N, Pape Møller AP. 2016. Migratory connectivity and effects of winter temperatures on migratory behaviour of the European robin Erithacus rubecula: A continent-wide analysis. Journal of Animal Ecology 85:749–760. DOI: 10.1111/1365-2656.12497.
- Atkinson MD, Atkinson E. 2002. Sambucus nigra L. Journal of Ecology 90(5):895–923. DOI: 10.1046/j.1365-2745.2002.00698.x.
- Bairlein F. 1998. The effect of diet composition on migratory fuelling in garden warblers Sylvia borin. Journal of Avian Biology 29(4):546–551. DOI: 10.2307/3677174.
- Bairlein F. 2002. How to get fat: Nutritional mechanisms of seasonal fat accumulation in migratory songbirds. Naturwissenschaften 89:1–10. DOI: 10.1007/s00114-001-0279-6.
- Bakken V, Runde O, Tjørve E. 2006. Norsk Ringmerkingsatlas. In: Stavange M, editor Norwegian bird ringing atlas, Vol. 2. Stavanger: Stavanger Museum. pp. 239–242.
- Bayly NJ, Gómez C, Hobson KA, González AM, Rosenberg KV. 2012. Fall migration of the veery (Catharus fuscescens) in northern Colombia: Determining the energetic importance of a stopover site. The Auk: Ornithological Advances 129:449–459. DOI: 10.1525/auk.2012.11188.
- Bengtsson D, Fransson T, Røer JE. 2009. Occurrence of continental Blackcaps Sylvia atricapilla in northern Europe. Ornis Svecica 19(1):41–49. DOI: 10.34080/os.v19.22660.
- Berthold P, Fiedler W, Schlenker R, Querner U. 1999. Bestandsveränderungen mitteleuropäischer Kleinvögel: Abschlußbericht zum MRI-Programm. Vogelwarte 40:1–10.
- Berthold P, Terrill SB. 1988. Migratory behaviour and population growth of Blackcaps wintering in Britain and Ireland: Some hypotheses. Ringing & Migration 9:153–159. DOI: 10.1080/03078698.1988.9673939.
- Biebach H, Friedrich W, Heine G. 1986. Interaction of bodymass, fat, foraging and stopover period in trans-Sahara migrating passerine birds. Oecologia 69:370–379. DOI: 10.1007/BF00377059.
- Bolser JA, Alan RR, Smith AD, Li L, Seeram NP, McWilliams SR. 2013. Birds select fruits with more anthocyanins and phenolic compounds during autumn migration. Wilson Journal of Ornithology 125(1):97–108. DOI: 10.1676/12-057.1.
- Bønløkke J, Madsen JJ, Thorup K, Pedersen KT, Bjernum M, Rahbek C. 2006. Dansk Trækfugleatlas. The Danish bird migration atlas. Københavns Universitet: Forlaget Rhodos A/S & Zoologisk Museum.
- Both C, Bouwhuis S, Lessells CM, Visser ME. 2006. Climate change and population declines in a long-distance migratory bird. Nature 441:81–83. DOI: 10.1038/nature04539.
- Both C, Visser ME. 2001. Adjustment to climate change is constrained by arrival date in a long-distance migrant bird. Nature 411:296–298. DOI: 10.1038/35077063.
- Brommer JE, Lehikoinen A, Valkama J, Clarke RH. 2012. The breeding ranges of Central European and Arctic bird species move poleward. PloS ONE 7(9):e43648. DOI: 10.1371/journal.pone.0043648.
- Buriánek V, Novotný R, Hellebrandová K, Šrámek V. 2013. Ground vegetation as an important factor in the biodiversity of forest ecosystems and its evaluation in regard to nitrogen deposition. Journal of Forest Science 59(6):238–252. DOI: 10.17221/16/2013-JFS.
- Busse P. 1992. Migratory behaviour of Blackcaps (Sylvia atricapilla) wintering in Britain and Ireland. Contradictory hypotheses. Ring 14:51–75.
- Busse P, Meissner W. 2015. Bird ringing station manual. Warsaw/Berlin: De Gruyter Open Ltd.
- Catoni C, Schaefer HM, Peters A. 2008. Fruit for health: The effect of flavonoids on humoral immune response and food selection in a frugivorous bird. Functional Ecology 22(4):649–654. DOI: 10.1111/j.1365-2435.2008.01400.x.
- Catry P, Lecoq M, Conway G, Felgueiras M, King JMB, Hamidi S. 2006. Are blackcaps Sylvia atricapilla differential distance migrants by sex? Ardeola 53:31–38.
- Chmielewski FM, Müller A, Küchler W. 2005. Possible impacts of climate change on natural vegetation in Saxony (Germany). International Journal of Biometeorology 50:96–104. DOI: 10.1007/s00484-005-0275-1.
- Cotton PA. 2003. Avian migration phenology and global climate change. Proceedings of the National Academy of Sciences of the United States of America 100:12219–12222. DOI: 10.1073/pnas.1930548100.
- Delmore K, Illera JC, Pérez-Tris J, Segelbacher G, Lugo Ramos JS, Durieux G, Ishigohoka J, Liedvogel M. 2020a. The evolutionary history and genomics of European blackcap migration. eLife 9:e54462. DOI: 10.7554/eLife.54462.
- Delmore KE, Van Doren BM, Conway GJ, Curk T, Garrido-Garduño T, Germain RR, Hasselmann T, Hiemer D, Vander Jeugd HP, Justen H, Lugo Ramos JS, Maggini I, Meyer BS, Phillips RJ, Remisiewicz M, Roberts GCM, Sheldon BC, Vogl W, Liedvogel M. 2020b. Individual variability and versatility in an eco-evolutionary model of avian migration. Proceedings of the Royal Society B 287:20201339. DOI: 10.1098/rspb.2020.1339.
- du Plessis KL, Martin RO, Hockey PAR, Cunningham SJ, Ridley AR. 2012. The costs of keeping cool in a warming world: Implications of high temperatures for foraging, thermoregulation and body condition of an arid-zone bird. Global Change Biology 18:3063–3070. DOI: 10.1111/j.1365-2486.2012.02778.x.
- Eggers S. 2000. Compensatory frugivory in migratory Sylvia warblers: Geographical responses to season length. Journal of Avian Biology 31:63–74. DOI: 10.1034/j.1600-048X.2000.310109.x.
- Ellegren H. 1991. Stopover ecology of autumn migrating Bluethroats Luscinia s. svecica in relation to age and sex. Ornis Scandinavica 22:340–348. DOI: 10.2307/3676506.
- Fiedler W. 2005. Ecomorphology of the external flight apparatus of Blackcaps (Sylvia atricapilla) with different migration behaviour. Annals New York Academy of Sciences 1046:253–263. DOI: 10.1196/annals.1343.022.
- Fransson T, Hall-Karlsson S. 2008. Swedish bird ringing atlas. Vol. 3, Passerines. Stockholm: Swedish Museum of Natural History.
- Gardner JL, Rowley E, de Rebeira P, de Rebeira A, Brouwer L. 2018. Associations between changing climate and body condition over decades in two southern hemisphere passerine birds. Climate Change Responses 5:2. DOI: 10.1186/s40665-018-0038-y.
- Gill JA, Alves JA, Sutherland WJ, Appleton GF, Potts PM, Gunnarsson TG. 2014. Why is timing of bird migration advancing when individuals are not? Proceedings of the Royal Society B 281:20132161. DOI: 10.1098/rspb.2013.2161.
- Gordo O. 2007. Why are bird migration dates shifting? A review of weather and climate effects on avian migratory phenology. Climate Research 35:37–58. DOI: 10.3354/cr00713.
- Gyurácz J, Bánhidi P. 2008. Dynamics and spatial distribution of migratory birds. Results of bird ringing project of Tömörd bird observatory 1998–2007. Szombathely, Hungary: University of West Hungary.
- Gyurácz J, Bánhidi P, Góczán J, Illés P, Kalmár S, Lukács Z, Molnár P, Németh C, Varga L. 2021. Temporal changes in wing length, fat reserves and body mass of migrating Eurasian Blackcaps Sylvia atricapilla at a west Hungarian stopover site. Acta Ornithologica 56(1):51–58. DOI: 10.3161/00016454AO2021.56.1.005.
- Gyurácz J, Bánhidi P, Góczán J, Illés P, Kalmár S, Lukács Z, Németh C, Molnár P, Varga L. 2017. Stopover strategies of Eurasian Blackcaps (Sylvia atricapilla) during the post-fledging period in western Hungary. Ornis Fennica 94(4):180–193. DOI: 10.51812/of.133923.
- Hansen J, Sato M, Ruedy R, Lo K, Lea DW, Medina-Elizade M. 2006. Global temperature change. Proceedings of the National Academy of Sciences 103(39):14288–14293. DOI: 10.1073/pnas.0606291103.
- Hedenström A, Alerstam T. 1997. Optimum fuel loads in migratory birds: Distinguishing between time and energy minimization. Journal of Theoretical Biology 189(3):227–234. DOI: 10.1006/jtbi.1997.0505.
- Hédl R, Kopecký M, Komárek J. 2010. Half a century of succession in a temperate oakwood: From species-rich community to mesic forest. Diversity and Distributions 16:267–276. DOI: 10.1111/j.1472-4642.2010.00637.x.
- Heinrichs S, Winterhoff W, Schmidt W. 2012. Vegetation dynamics of beech forests on limestone in central Germany over half a century – effects of climate change, forest management, eutrophication or game browsing? In: Dengler J, Oldeland J, Jansen F, Chytrý M, Ewald J, Finckh M, Glöckler F, Lopez-Gonzalez G, Peet R, Schaminée J, editors. Vegetation databases for the 21st century, Biodiversity & Ecology 4. pp. 49–61. DOI: 10.7809/b-e.00059.
- Holloway GJ, Edwards PJ. 1989. Biometrics, capture date and sex ratio in relation to migration in Blackcaps. Ringing & Migration 10:108–112. DOI: 10.1080/03078698.1989.9673948.
- Hüppop O, Winkel W. 2006. Climate change and timing of spring migration in the long-distance migrant Ficedula hypoleuca in central Europe: The role of spatially different temperature changes along migration routes. Journal of Ornithology 147:344–353. DOI: 10.1007/s10336-005-0049-x.
- Jenni L, Kéry M. 2003. Timing of autumn bird migration under climate change: Advances in long-distance migrants, delays in short-distance migrants. Proceedings of the Royal Society B 270:1467–1471. DOI: 10.1098/rspb.2003.2394.
- Kaiser A. 1999. Stopover strategies in birds: A review of methods for estimating stopover length. Bird Study 46:S299–S308. DOI: 10.1080/00063659909477257.
- Kopiec K, Ożarowska A. 2012. The origin of Blackcaps Sylvia atricapilla wintering on the British Isles. Ornis Fennica 89(4):254–263. DOI: 10.51812/of.133813.
- Labocha MK, Hayes JP. 2012. Morphometric indices of body condition in birds: A review. Journal of Ornithology 153(1):1–22. DOI: 10.1007/s10336-011-0706-1.
- Lo Valvo F, Lo Verde G, Lo Valvo M. 1988. Relationships among wing length, wing shape and migration in Blackcap Sylvia atricapilla populations. Ringing & Migration 9(1):51–54. DOI: 10.1080/03078698.1988.9673923.
- Marra PP, Francis CM, Mulvihill RS, Moore FR. 2005. The influence of climate on the timing and rate of spring bird migration. Oecologia 142:307–315. DOI: 10.1007/s00442-004-1725-x.
- McCullagh P, Nelder JA. 1989. Generalized linear models. London: Chapman and Hall.
- McKechnie AE, Wolf BO. 2010. Climate change increases the likelihood of catastrophic avian mortality events during extreme heat waves. Biology Letters 6:253–256. DOI: 10.1098/rsbl.2009.0702.
- Menzel A. 2000. Trends in phenological phases in Europe between 1951 and 1996. International Journal of Biometeorology 44:76–81. DOI: 10.1007/s004840000054.
- Menzel A, Fabian P. 1999. Growing season extended in Europe. Nature 397:659. DOI: 10.1038/17709.
- Menzel A, Sparks TH, Estrella N, Roy DB. 2006. Altered geographic and temporal variability in phenology in response to climate change. Global Ecology and Biogeography 15:498–504. DOI: 10.1111/j.1466-822X.2006.00247.x.
- Minias P, Kaczmarek K, Włodarczyk R, Janiszewski T. 2010. Variation in autumn migration strategy in the first-year wood sandpipers Tringa glareola. Acta Ornithologica 45:131–138. DOI: 10.3161/000164510x551273.
- Nowakowski JK. 1999. Terms of autumn migration of the genus Sylvia in central Poland. Ring 21(2):3–13.
- Ożarowska A. 2015. Contrasting fattening strategies in related migratory species: The blackcap, garden warbler, common whitethroat and lesser whitethroat. Annales Zoologici Fennici 52:115–127. DOI: 10.5735/086.052.0210.
- Ożarowska A, Zaniewicz G. 2015. Temporal trends in the timing of autumn migration of short- and long-distance migrating Blackcaps (Sylvia atricapilla). Ornis Fennica 92(3):144–152. DOI: 10.51812/of.133875.
- Ożarowska A, Zaniewicz G, Meissner W. 2016. Blackcaps Sylvia atricapilla on migration: A link between long-term population trends and migratory behaviour revealed by the changes in wing length. Acta Ornithologica 51:211–219. DOI: 10.3161/00016454AO2016.51.2.007.
- Ożarowska A, Zaniewicz G, Meissner W. 2018. Spring arrival timing differs between the groups of blackcaps (Sylvia atricapilla) wintering in distinct regions. Annales Zoologici Fennici 55:45–54. DOI: 10.5735/086.055.0105.
- Ożarowska A, Zaniewicz G, Meissner W. 2021. Sex and age-specific differences in wing pointedness and wing length in blackcaps Sylvia atricapilla migrating through the southern Baltic coast. Current Zoology 67:271–277. DOI: 10.1093/cz/zoaa065.
- Pérez-Tris J, Tellería JL. 2002. Migratory and sedentary blackcaps in sympatric non-breeding grounds: Implications for the evolution of avian migration. Journal of Animal Ecology 71:211–224. DOI: 10.1046/j.1365-2656.2002.00590.x.
- Pulido F, Berthold P. 2004. Microevolutionary response to climatic change. Adv Ecol Res 35:151–183. DOI: 10.1016/S0065-2504(04)35008-7.
- Pulido F, Widmer M. 2005. Are long-distance migrants constrained in their evolutionary response to environmental change? Causes of variation in the timing of autumn migration in a Blackcap (S. atricapilla) and two garden warbler (Sylvia borin) populations. Annals of the New York Academy of Sciences 1046:228–241. DOI: 10.1196/annals.1343.020.
- Rakhimberdiev E, Duijns S, Karagicheva J, Camphuysen CJ, Castricum VRS, Dekinga A, Dekker R, Gavrilov A, Ten Horn J, Jukema J, Saveliev A, Soloviev M, Tibbitts TL, van Gils JA, Piersma T. 2018. Fuelling conditions at staging sites can mitigate Arctic warming effects in a migratory bird. Nature Communications 9:4263. DOI: 10.1038/s41467-018-06673-5. Erratum in: Nature Communications 9:5189.
- Remisiewicz M, Underhill LG. 2022. Large-scale climatic patterns have stronger carry-over effects than local temperatures on spring phenology of long-distance passerine migrants between Europe and Africa. Animals 12:1732. DOI: 10.3390/ani12131732.
- Rolshausen G, Hobson KA, Schaefer HM. 2010. Spring arrival along a migratory divide of sympatric Blackcaps (Sylvia atricapilla). Oecologia 162:175–183. DOI: 10.1007/s00442-009-1445-3.
- Schaub M, Jenni L. 2000. Fuel deposition of three passerine bird species along the migration route. Oecologia 122(3):306–317. DOI: 10.1007/s004420050036.
- Schaub M, Jenni L. 2001. Stopover durations of three warbler species along their autumn migration route. Oecologia 128:217–227. DOI: 10.1007/s004420100654.
- Schaub M, Jenni L, Bairlein F. 2008. Fuel stores, fuel accumulation, and the decision to depart from a migration stopover site. Behavioral Ecology 19(3):657–666. DOI: 10.1093/beheco/arn023.
- Schleip C, Sparks TH, Estrella N, Menzel A. 2009. Spatial variation in onset dates and trends in phenology across Europe. Climate Research 39:249–260. DOI: 10.3354/cr00830.
- Schwartz MD, Ahas R, Aasa A. 2006. Onset of spring starting earlier across the northern hemisphere. Global Change Biology 12:343–351. DOI: 10.1111/j.1365-2486.2005.01097.x.
- Shirihai H, Gargallo G, Helbig A. 2001. Sylvia warblers: Identification, taxonomy and phylogeny of the genus Sylvia. London: Christopher Helm Publishers Ltd.
- StatSoft Inc. 2014. STATISTICA (data analysis software system), version 12. Available: www.statsoft.com.
- Stępniewska K, Ożarowska A, Busse P, Bobrek R, Zehtindjiev P, Ilieva M, Meissner W. 2020. Autumn migration strategy of juvenile great reed warblers Acrocephalus arundinaceus on the eastern European flyway: A spatiotemporal pattern of accumulation and utilisation of energy stores. European Zoological Journal 87(1):537–551. DOI: 10.1080/24750263.2020.1814882.
- Svensson L. 1992. Identification guide to European passerines. Stockholm: Svensson.
- Szabó B, Vincze E, Czúcz B. 2016. Flowering phenological changes in relation to climate change in Hungary. International Journal of Biometeorology 60:1347–1356. DOI: 10.1007/s00484-015-1128-1.
- Tamis WLM, Van’t Zelfde M, Van Der Meijden R, Udo De Haes HA. 2005. Changes in vascular plant biodiversity in the Netherlands in the 20th century explained by their climatic and other environmental characteristics. Climatic Change 72:37–56. DOI: 10.1007/s10584-005-5287-7.
- Thorup K, Tøttrup AP, Rahbek C. 2007. Patterns of phenological changes in migratory birds. Oecologia 151:697–703. DOI: 10.1007/s00442-006-0608-8.
- Tøttrup AP, Thorup K, Rahbek C. 2006. Changes in timing of autumn migration in North European songbird populations. Ardea 94:527–536.
- Tøttrup AP, Thorup K, Rainio K, Yosef R, Lehikoinen E, Rahbek C. 2008. Avian migrants adjust migration in response to environmental conditions en route. Biology Letters 4:685–688. DOI: 10.1098/rsbl.2008.0290.
- Tryjanowski P, Kuźniak S, Sparks TH. 2002. Earlier arrival of some farmland migrants in western Poland. Ibis 144:62–68. DOI: 10.1046/j.0019-1019.2001.00022.x.
- van Gils JA, Lisovski S, Lok T, Meissner W, Ożarowska A, de Fouw J, Rakhimberdiev E, Soloviev MY, Piersma T, Klaassen M. 2016. Body shrinkage due to Arctic warming reduces red knot fitness in tropical wintering range. Science 352(6287):819–821. DOI: 10.1126/science.aad6351.
- Visser ME, Perdeck AC, van Balen JH, Both C. 2009. Climate change leads to decreasing bird migration distances. Global Change Biology 15:1859–1865. DOI: 10.1111/j.1365-2486.2009.01865.x.
- Wojciechowski MS, Yosef R, Pinshow B. 2014. Body composition of north and southbound migratory blackcaps is influenced by the lay-of-the-land ahead. Journal of Avian Biology 45:264–272. DOI: 10.1111/j.1600-048X.2013.00345.x.
- Yosef R, Wineman A. 2010. Differential stopover of Blackcap (Sylvia atricapilla) by sex and age at Eilat, Israel. Journal of Arid Environments 74:360–367. DOI: 10.1016/j.jaridenv.2009.09.004.
- Zaniewicz G 2014. Differentiation in migration strategy and biometrics of blackcaps (Sylvia atricapilla) captured at the Polish Baltic coast in autumn [ Dissertation]. University of Gdańsk. [In Polish].
- Zaniewicz G, Busse P. 2010. Like a phoenix from the ashes. Ring 32:17–30. DOI: 10.2478/v10050-010-0002-0.
- Zduniak P, Yosef R, Meyrom K. 2013. A comparison of passerine migration in southern and northern Israel. Journal of Arid Environments 90:22–28. DOI: 10.1016/j.jaridenv.2012.09.016.