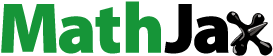
Abstract
Urban beekeeping has gained interest in recent years. Bees placed in cities, on the roofs of buildings, are exposed to many different sources of electromagnetic fields of different frequencies and intensities. Knowledge about the impact of electromagnetic fields on the physiology and behavior of insects (including honey bees) is limited. Hence, one of the first steps was to check how radiofrequency electromagnetic fields affect honey bees’ nutrition. The level of total proteins, glucose, triglycerides, and TAS activity in bee hemolymph was analyzed. This is because they indicate the honey bee’s nutrition level. The experiment involved 2-day-old Carniolan worker honey bees (Apis mellifera carnica L.). The bees were exposed to fields of 12 V/m, 28 V/m, and 61 V/m for 0.25, 1 and 3 h. Hemolymph was taken from 40 alive worker honey bees from each group immediately after exposure, by removing the antennae with sterile tweezers. The activity of biochemical markers (total proteins, TAS, and triglycerides) in the bee hemolymph in 12 V/m and 28 V/m groups showed statistically significant differences compared to the control group in most cases. According to our own research, radiofrequency electromagnetic fields disturb honey bee nutrition. The changes in the analyzed indicators may have long-term effects.
Introduction
Current radio communication systems use the electromagnetic field with frequencies ranges from approx. 180 kHz to 90 GHz, although bands up to 300 GHz are expected to be developed. The most popular of radio communication systems today – cellular systems – typically use the 800 MHz, 900 MHz, 1800 MHz, 2100 MHz, 2600 MHz bands and the latest 5 G systems − 3600 MHz (Ziegelberger Citation2009; Bakker et al. Citation2010). EM fields exposure levels limits for workplace and public environments are defined by appropriate environmental protection regulations. Best known are the ICNIRP guidelines, but local regulations had also been established (Ziegelberger Citation2009). The E-field limits for radio waves and microwaves are frequency-dependent and typically remains within the range of 6 V/m to 61 V/m. To initially estimate the far field E-field, the dependence of the E-field intensity on the distance from the source and the power emitted in the direction of maximum radiation can be used. For this reason, not only humans (Bakker et al. Citation2010) but also insects (Balmori Citation2021) (especially flying) are exposed to the threat posed by RF-EMF (Zmyślony et al. Citation2020)
Where: EIRP – equivalent isotropic radiated power through the antenna [W], r – distance from the antenna [m].
For typical cellular systems, the total EIRP for antennas can be assumed to be in the range of 2 kW to 20 kW. presents the maximal distance, calculated according to formula (1), up to which the exemplary field strengths on the direction of maximum antenna radiation are exceeded.
Table I. Exemplary E-field calculations in a public environment, taking as the source the transmitting antennas of typical cellular systems base station according to EquationEquation 1(1)
(1) .
It should also be noted that the environment is full of sources of higher (e.g. radars) or lower power (radio link) (Balmori Citation2021). But also insects (Zmyślony et al. Citation2020) (especially flying) are exposed to the threat posed by RF-EMF.
Urban beekeeping has gained interest in recent years (Salkin Citation2012). Bees are placed in cities where there are many different sources of electromagnetic fields. Placing beehives on the roofs of buildings in urban centers is becoming increasingly popular. In this way, they are exposed to electromagnetic fields of various frequencies and intensities (Gallai et al. Citation2009; Lorenz & Stark Citation2015; Migdał et al. Citation2020).
Worker bees penetrate the environment in search of nectar and pollen. They are the basis of a bee’s diet and a source of protein, carbohydrates, fats and minerals. Proteins and amino acids derived from food are involved in the formation of the cytoskeleton, enzyme production, tissue construction, and formation of immune proteins (Azevedo et al. Citation2016). In a honey bee’s body, the proteins concentration in the hemolymph ranges from 1% to 10%, hence proteins is considered one of its main components. The concentration of proteins in a honey bee’s hemolymph depends on the bee’s age, the availability of nutrient sources in the environment, and the degree of environmental pollution (Bounias & PARIS Citation1975; Domatskaya Citation1980; Weinberg & Madel Citation1985; Moritz & Crailsheim Citation1987).
During the day, the honey bee can cover a distance of up to 13.5 km searching for food (Beekman & Ratnieks Citation2000) which means it has a high energy requirement. Carbohydrates are the primary source of energy for honey bees (Thompson Citation2003). The main sugar found in hemolymph is glucose. It may come from fructose conversion in the metabolic pathway. It is stored in the form of trehalose or glycogen (Blatt & Roces Citation2001). Honey bees use fat (triglycerides) stored in the fat body as an energy reserve in periods of no flow or wintering. This fat also provides energy during a long flight and during bee colonies’ spring development (Hahn & Denlinger Citation2007; Chobotow & Strachecka Citation2013).
Environmental stress has a very large impact on the honey bee body’s proper functioning. One of the lines of defense against oxidative stress is the antioxidant system (Migdał et al. Citation2020). Oxidative stress is a condition in which the antioxidant defense system cannot counteract free radicals damage (Halliwell Citation2007). The lack of food in the environment as well as the lack of proper bee feeding in winter can cause oxidative stress (Tawfik et al. Citation2020). In studies on the impact of modulated radiofrequency electromagnetic fields (900 MHz) and intensity of 23 V/m for 2 h on honey bee larvae, it was shown that exposure had quite a significant effect on the antioxidant system activity. Glutathione S-transferase activity decreased while catalase activity significantly increased in honey bee larvae after exposure (Vilić et al. Citation2021). The influence of the radiofrequency electromagnetic field already at the level of the mobile phone frequency (900 MHz) reduced the hatching ratio but not the mating success (Odemer & Odemer Citation2019).
So far, studies on RF EMF have shown no effect on the reproductive capacity of small invertebrates using frequencies of 900 MHz (Vijver Citation2014). In a study on ants using 940 MHz, a high effect on their behavior was shown. Ant locomotion has changed significantly and orientation to their alarm pheromone has become poorer (Cammaerts et al. Citation2014). Knowledge about the impact of electromagnetic fields on the physiology and behavior of insects (including honey bees) is limited.
Exposure to an electromagnetic field with a frequency of 50 Hz showed a significant effect on nutritional and antioxidant indicators of the hemolymph of honey bees, such as, among others: total protein, glucose and triglycerides (Migdał et al. Citation2021). The effect on these indicators of radio frequency fields, including specific 900 MHz frequency intensities, has not yet been thoroughly investigated, but reports using cell-phone radiations indicate a possible impact of this factor on the nutritional parameters of honey bee hemolymph (Kumar et al. Citation2011) (Toribio et al. Citation2022). The level of total proteins, glucose, triglycerides and TAS activity in bee hemolymph were analyzed, because they indicate the degree of nutrition of the honey bee’s body.
Material and methods
Honey bee worker rearing
The Carniolan worker honey bees (Apis mellifera carnica L.) were used in the experiment. The bees were 2 days old. To obtain bees of the same age, in 10 randomly picked colonies, the queen originating from the same mother-queen colony and inseminated with the drone semen from the same father-queen colony was placed in an isolator with an empty comb (435 × 300 mm) for egg-laying. After 20 days of brood development, the combs with bee brood were transferred to an incubator (temp. 34.4°C ±0.5°C, relative humidity of 70% ± 5%) for the emerging of worker bees. After the emergence, bees were randomly placed in cages (20 × 15 × 8 cm), according Migdal et al. (Migdał et al. Citation2020).
Exposure of honey bees to radio frequency electromagnetic field
To perform the experiment, a 900 MHz electromagnetic field with narrowband FSK modulation was used. 900 MHz is a frequency representative for the bands used in, for example, mobile telephony. Use of modulation is intended to make the signal more similar to real radiocommunication signals. Under conditions of real exposure to electromagnetic fields, there are practically no unmodulated signals, so studies of the impact of a pure carrier wave can be considered unrepresentative.
In order to keep the exposure conditions as close as possible to real environmental exposure, in experiment was used exposure system with the EMF field emitted by the antenna in free space, and the test objects were placed in the far field of the antenna. The setup configuration ensured the absence of reflections and multipath propagation. Field strength uniformity and stability were controlled by measuring the field strength distribution in the whole exposure area before the start of the tests and by continuous monitoring the power delivered to the antenna in the experiment duration. The measurements were carried out by the accredited testing laboratory LWiMP AB-361 using a wideband NBM-520 S/N C-0062 meter with probe EF-1891 S/N A-0335 calibrated by an accredited calibration laboratory AP-078 (calibration certificate LWiMP/W/082/21).
The electromagnetic background in the measurement area (resultant field from other sources with the 900 MHz signal generator turned off) did not exceed 1 V/m in the 3 MHz-18 GHz band and 100 V/m for 50 Hz. The bees were exposed to EM fields of 12 V/m, 28 V/m and 61 V/m, for 0.25, 1 and 3 h using EMF test signal source. 25’ - short-term exposure to the electromagnetic field during a short reconnaissance flight of the worker bee. 1 h – the average time a worker bee stays in the environment in search of food – during a single flight. 3 h – the time a worker bee spends on bringing large and heavy portions of food from distant sources – during a single flight (Bradbear Citation2009). Field strength inhomogeneity over the whole exposure area not exceeding, respectively: ±5% for field strength of 12 V/m, ±7% for 28 V/m and ± 9% for 61 V/m. Fluctuations in field strength during the experiment did not exceed ± 3% ().
Figure 1. The exposure system consists of a 900MHz modulated signal generator, amplifier, power control system and antenna. Cages with bees were located in the far field of the antenna’s main beam-at distances from the antenna, where the desired field strengths of 12V/m, 28V/m and 61V/m were obtained.
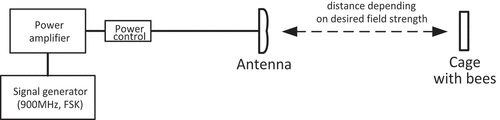
Exposure of bees to radiofrequency electromagnetic fields was carried out in laboratory conditions. The bees from the control group were placed in a separate laboratory in order to avoid the influence of the generated field.
Biochemical markers analysis
Hemolymph was taken from 40 alive worker honey bees from each group immediately after exposure to RF-field, by removing the antennae with sterile tweezers. Hemolymph was collected in a 20 μl glass capillary. After collection, samples were stored at −80°C according to the method of Migdał et al. (Citation2020). Total protein concentration, glucose concentration, triglycerides concentration and total antioxidant status were determined with ABX Pentra assay kit (HORIBA ABX Diagnostics, France) according to the manufacturer’s recommendation. For all analyses ABX Pentra 400 was used. For each analysis of total proteins concentration 2 µl of hemolymph was used, for analysis of glucose concentration 4 µl of hemolymph was used, for analysis of triglycerides concentration 3 µl of hemolymph was used and for analysis of Total Antioxidant Status 5 µl of hemolymph was used.
Data analysis
For all statistical analysis, R 4.1.2 with RStudio (R Core Team 2021) was used. In all tests, the level of significance was α = 0.05. Normality of data distribution was tested by Shapiro–Wilk test. Kruskal–Wallis test with Holm correction for multiple comparison was used to check differences between groups (package “agricolae”) with the significance level of α = 5%.
Results
The activity of biochemical markers (total proteins, TAS, and triglycerides) in the bee hemolymph in 12 V/m and 28 V/m groups showed statistically significant differences compared to the control group (C) in most cases. The 12 V/m group exposed for 1 h showed statistically significant differences for all biochemical markers (). In the group 61 V/m, fewer statistically significant differences can be noted.
Table II. Mean value of selected biochemical markers in the hemolymph of worker bees after exposure to a radio field with a frequency of 900 MHz.
Total proteins concentration
The highest number of statistically significant differences compared to the control group (C) can be seen in the protein content (). Only the 61 V/m group with exposure times of 0.25 h and 1 h did not show statistically significant differences with the control group (C). The protein content in all groups was lower than in the control group (C). The highest protein content in the bee hemolymph was recorded in the 61 V/m group with an exposure time of 0.25 h. This value was lower than the control group (C) by 15.5%. The lowest protein content in the bee hemolymph was recorded in the 12 V/m group with an exposure time of 1 h. This value was lower than the control group (C) by 59%.
Glucose concentration
The bees’ hemolymph glucose content compared to the control group (C) in the 12 V/m and 61 V/m groups was significantly higher after 0.25 h, then lower after 1 h, and again higher after 3 h of exposure (). The opposite situation can be seen in the 28 V/m group – the glucose content was first lower, then higher, and then lower again. The highest glucose content in the bee hemolymph was recorded in the 61 V/m group at the exposure time of 3 h. This value was higher than in the control group (C) by 60.9%. The lowest glucose content in the bee hemolymph was recorded in the group of 61 V/m with the exposure time of 1 h. This value was lower than in the control group (C) by 47.5%.
Triglycerides concentration
The content of triglycerides in the bee hemolymph after exposure to the radio field is lower than in the control group (C) in most cases (). Only in the 28 V/m group with exposure time of 1 h and 61 V/m group with exposure time of 1 h and 3 h, the content of triglycerides is higher than in the control group (C). The highest content of triglycerides in the bee hemolymph was recorded in the 28 V/m group with the exposure time of 1 h. This value was higher than in the control group (C) by 7.9%. The lowest content of triglycerides in the bee hemolymph was recorded in the 28 V/m group with the exposure time of 0.25 h. This value was lower than in the control group (C) by 48.7%.
Total antioxidant status (TAS) concentration
The activity of TAS in the bee hemolymph, compared to the control group (C), was lower in all groups except for the 61 V/m group at the exposure time of 0.25 h and 1 h where the activity was higher (). The highest TAS activity in the bee hemolymph was recorded in the 61 V/m group with the exposure time of 0.25 h. This value was higher than in the control group (C) by 8.3%. The lowest activity of TAS in the bee hemolymph was recorded in the 28 V/m group with the exposure time of 3 h. This value was lower than in the control group (C) by 44.2%.
Discussion
Taking into account the exposure time, only in the 12 V/m group it can be seen that the content of individual biochemical markers in the bee hemolymph first decreased and then increased to a greater or lesser extent. It can therefore be concluded that the correct interaction of the parameters with respect to each other has been preserved. Disturbances in physiological processes begin in the 28 V/m group and in the 61 V/m group. It could be concluded that the disturbance of these physiological changes could be affected by the time spent in the radiofrequency electromagnetic field at given intensities.
Total proteins concentration
Comparing the obtained results with the control group (C), the most statistically significant differences could be noticed in the protein content. Under the influence of the radiofrequency electromagnetic field, the level of protein in the bee hemolymph in the study groups was significantly lower than in the control group (C). In the 28 V/m group, only the protein level was different from the other research parameters, and both of these parameters differed in levels from each other. The level of total proteins in insect hemolymph may affect the antioxidant system activity. Hemolymph proteins plays a key role in insect physiology, including nutrient transport, waste removal, and immune defense. The proteins in the hemolymph may also act as antioxidants themselves, providing a first line of defense against ROS (Felton & Summers Citation1995).
A study conducted on the tobacco hornbill (Manduca sexta) showed that dietary proteins restriction resulted in a decrease in proteins levels in the hemolymph and a decrease in the activity of antioxidant enzymes. Scientists hypothesized that this was due to a decrease in the availability of amino acids, which are essential building blocks of proteins and enzymes, leading to a decrease in the synthesis of these molecules (Wilson et al. Citation2019). Another study on the desert locust (Schistocerca gregaria) showed that a high-protein diet led to an increase in the activity of antioxidant enzymes in the hemolymph. Researchers suggested that this was due to an increase in the amino acids availability, which may have stimulated the synthesis of these enzymes (Xiao et al. Citation2023).
Migdał et al. (Citation2021) researched the effect of an electromagnetic field with a frequency of 50 Hz on the concentration of protein in the honeybee’s hemolymph at different times and intensity of exposure. In the studies, it was noticed that under the influence of the electromagnetic field, the protein concentration in the bee hemolymph increased significantly, which indicated disturbances in its distribution in the body. The results of both studies indicate changes in protein concentration. These changes depend on the frequency to which the worker honeybees were exposed. Lower frequencies caused an increase in protein concentration, radio frequencies caused a decrease in its content in the hemolymph. Such a state may indicate various changes caused by these ranges of electromagnetic field in the body of the honey bee.
In general, total proteins levels in insect hemolymph may influence the activity of the antioxidant system. This is possibly through its role in providing amino acids for the synthesis of antioxidant enzymes. However, the specific mechanisms underlying this relationship may vary depending on the insect species and its diet.
Glucose concentration
In general, the level of glucose in insect hemolymph can have a significant impact on the antioxidant system activity, especially the glutathione system. It involves the production of glutathione, a tripeptide that scavenges free radicals and reduces ROS. The glutathione system activity may be influenced by the level of glucose in the insect’s hemolymph (Liu et al. Citation2021).
Studies have shown that when blood glucose levels are high, the glutathione system activity can be suppressed. This is because high sugar levels can lead to an increase in ROS production, which can overload the antioxidant system and lead to oxidative stress (Bajaj & Khan Citation2012; Papachristoforou et al. Citation2020).
On the other hand, when blood glucose levels are low, the glutathione system activity can be increased. This is because low glucose levels can lead to a reduction in the ROS production, which allows the antioxidant system to function more effectively (Papachristoforou et al. Citation2020).
Migdał et al. (Citation2021). researched the effect of a 50 Hz electromagnetic field on the glucose concentration in the honey bee’s hemolymph with varying duration and intensity of exposure. The studies noted that the glucose concentration after exposure to the E-field with a frequency of 50 Hz was statistically significantly lower in all groups than in the control group. Despite the differences in the frequencies studied, it was noticeable in our own research that most of the groups also showed a statistically significantly lower glucose concentration in the hemolymph than the control group.
Triglycerides concentration
The fewest statistically significant differences in comparison with the control group (C) occurred when examining the level of triglycerides.
The level of body fat and glucose in the hemolymph are not directly related. However, there are various factors that affect the level of fat in insects’ body, including the level of glucose in the hemolymph (Kaczmarek & Boguś Citation2021).
In insects’ diet, glucose is one of the main sources of energy, and glucose levels in the hemolymph are regulated by hormones and the nervous system. If glucose levels in the hemolymph is low, insects can break down stored fat for energy (Ziegler & Ibrahim Citation2001; Zhou et al. Citation2004).
Studies have shown that in some insect species (e.g., the fruit fly Drosophila melanogaster), high hemolymph glucose levels lead to increased fat synthesis and body fat storage, and low hemolymph glucose levels lead to fat mobilization and weight loss (Lee et al. Citation2008; Grönke et al. Citation2010).
Migdał et al. (Citation2021) studied the effect of an electromagnetic field with a frequency of 50 Hz on the concentration of triglycerides in the honey bee’s hemolymph, at different times and intensities of exposure. It was observed that the differences in the study groups after exposure to the EMF were statistically significant compared to the control group. Despite the differences in the frequencies studied, similar differences were also observed in our own research.
Currently, there are few studies on the effect of the level of fat in insects’ diet on the activity of their antioxidant system. A study on fruit flies (Drosophila melanogaster) showed that a high-fat diet caused an increase in reactive oxygen species (ROS) in the body, which triggered an increase in the activity of the antioxidant system (D’Antonio et al. Citation2021).
Total antioxidant status (TAS) concentration
By examining the functioning of the honey bees antioxidant system, we can obtain a lot of information about antioxidants in the body. The antioxidant system is one of the system that protects the body’s cells from damage caused by reactive oxygen species (ROS), which is formed during normal cellular processes or in response to stressors such as exposure to toxins, radiation or infection. The antioxidant system includes several enzymes, such as superoxide dismutase (SOD), catalase (CAD) and glutathione peroxidase (GPx), which together neutralize ROS (Harrison & Bonning Citation2010; Farjan et al. Citation2015; Sies Citation2020).
The influence of nutrition on the antioxidant system of insects is still under research, and there is no clear answer yet. It can be assumed that the antioxidant system is a very good indicator of proper nutrition of insects (including honeybees).
Skowronek and Strachecka (Skowronek & Strachecka Citation2023) investigated the effect of cannabidiol (CBD) on the honeybee’s antioxidant system. The results of the research showed that CBD supplementation can support the honeybee’s immune system by stimulating the antioxidant system.
Krishnan and Kordik (Krishnan & Kodrík Citation2006) fed two different diets to the Spodoptera littoralis caterpillar to see how they affected the antioxidant enzymes in the intestinal contents and tissues. The results confirmed that intestinal-derived antioxidant enzymes protect against endogenous and exogenous oxidative radicals.
Migdal et al. (Citation2023) conducted a study on the effect of the 900 MHz electromagnetic field on the level of enzymatic activity and the expression of stress-related genes. Enzymes such as AST, ALT, ALP and GGTP are involved in the body’s defense responses to oxidative stress. The results they obtained are quite similar to those obtained in their own research. The activity of the tested enzymes decreased after exposure. In our own research, a decrease in the activity of the oxidative system after exposure to the radio field can also be seen.
Conclusion
The results of the research confirmed that the radiofrequency electromagnetic field can cause disturbances in the proper nutrition of honey bees organism. The changes that have taken place in the analyzed indicators may have long-term effects. It is still difficult to describe the mechanism of how the radiofrequency electromagnetic field affects the body of insects. The experiment was carried out in the 900 MHz band, representing the lower frequency bands used in mobile phone systems. Further research work using higher frequencies is planned.
In further stages, it is also planned to extend the research with field research, which should provide more detailed information on the impact of RF-EMF on honeybees, as individual individuals may differ in sensitivity and degree of exposure to RF-EMF depending on sex, age.
Disclosure statement
No potential conflict of interest was reported by the author(s).
Data availability statement
The datasets used and/or analysed during the current study available from the corresponding author on reasonable request.
Additional information
Funding
References
- Azevedo DO, De Paula SO, Zanuncio JC, Martinez LC, Serrão JE. 2016. Juvenile hormone downregulates vitellogenin production in Ectatomma tuberculatum (Hymenoptera: Formicidae) sterile workers. Journal of Experimental Biology 219:103–108. DOI: 10.1242/jeb.127712.
- Bajaj S, Khan A. 2012. Antioxidants and diabetes. Indian Journal of Endocrinology and Metabolism 16(8):S267. DOI: 10.4103/2230-8210.104057.
- Bakker JF, Paulides MM, Christ A, Kuster N, Van Rhoon GC. 2010. Assessment of induced SAR in children exposed to electromagnetic plane waves between 10 MHz and 5.6 GHz. Physics in Medicine & Biology 55(11):3115. DOI: 10.1088/0031-9155/55/11/009.
- Balmori A. 2021. Electromagnetic radiation as an emerging driver factor for the decline of insects. Science of the Total Environment 767:144913. DOI: 10.1016/j.scitotenv.2020.144913.
- Beekman M, Ratnieks FLW. 2000. Long-range foraging by the honey-bee, Apis mellifera L. Functional Ecology 14(4):490–496. DOI: 10.1046/j.1365-2435.2000.00443.x.
- Blatt J, Roces F. 2001. Haemolymph sugar levels in foraging honeybees (Apis mellifera carnica): Dependence on metabolic rate and in vivo measurement of maximal rates of trehalose synthesis. Journal of Experimental Biology 204(15):2709–2716. DOI: 10.1242/jeb.204.15.2709.
- Bounias M, PARIS F. 1975. Les proteines de I’hemolymphe chez l’abeille Apis mellifica mellifica L. I - Larves, nymphes et adultes naissantes. Apidologie 6(3):219–232. DOI: 10.1051/apido:19750303.
- Bradbear N. 2009. Bees and their role in forest livelihoods. Food and Agricultural Organization 3:13–16.
- Cammaerts MC, Vandenbosch GAE, Volski V. 2014. Effect of short-term GSM radiation at representative levels in society on a biological model: The ant myrmica sabuleti. Journal of Insect Behavior 27(4):514–526. DOI: 10.1007/s10905-014-9446-4.
- Chobotow J, Strachecka A. 2013. Morphology and function of insect fat bodies taking into account Apis mellifera L. Medical Veterinary 69:712–771.
- D’Antonio V, Serafini M, Battista N. 2021. Dietary modulation of oxidative stress from edible insects: A mini-review. Frontiers in Nutrition 8:38. DOI: 10.3389/fnut.2021.642551.
- Domatskaya TF. 1980. Indices of hemolymph in the bees in varroatosis. Veterinaria 57:47–48.
- Farjan M, Dmitryjuk M, Lipiński Z, Biernat-Łopieńska E, Zółtowska K. 2015. Supplementation of the honey bee diet with vitamin C: The effect on the antioxidative system of Apis mellifera carnica brood at different stages. Journal of Apicultural Research 51(3):263–270. DOI: 10.3896/IBRA.1.51.3.07.
- Felton GW, Summers CB. 1995. Antioxidant systems in insects. Archives of Insect Biochemistry and Physiology 29(2):187–197. DOI: 10.1002/arch.940290208.
- Gallai N, Salles JM, Settele J, Vaissière BE. 2009. Economic valuation of the vulnerability of world agriculture confronted with pollinator decline. Ecological Economics 68(3):810–821. DOI: 10.1016/j.ecolecon.2008.06.014.
- Grönke S, Clarke DF, Broughton S, Andrews TD, Partridge L, Rulifson E. 2010. Molecular evolution and functional characterization of drosophila insulin-like peptides. PLoS Genetics 6(2):e1000857. DOI: 10.1371/journal.pgen.1000857.
- Hahn DA, Denlinger DL. 2007. Meeting the energetic demands of insect diapause: Nutrient storage and utilization. Journal of Insect Physiology 53(8):760–773. DOI: 10.1016/j.jinsphys.2007.03.018.
- Halliwell B. 2007. Oxidative stress and cancer: Have we moved forward? Biochemical Journal 401(1):1–11. DOI: 10.1042/BJ20061131.
- Harrison RL, Bonning BC. 2010. Proteases as insecticidal agents. Toxins 2(5):935–953. DOI: 10.3390/toxins2050935.
- Kaczmarek A, Boguś M. 2021. The metabolism and role of free fatty acids in key physiological processes in insects of medical, veterinary and forensic importance. PeerJ 9:e12563. DOI: 10.7717/peerj.12563.
- Krishnan N, Kodrík D. 2006. Antioxidant enzymes in Spodoptera littoralis (Boisduval): Are they enhanced to protect gut tissues during oxidative stress? Journal of Insect Physiology 52(1):11–20. DOI: 10.1016/j.jinsphys.2005.08.009.
- Kumar NR, Sangwan S, Badotra P. 2011. Exposure to cell phone radiations produces biochemical changes in worker honey bees. Toxicology International 18(1):70. DOI: 10.4103/0971-6580.75869.
- Lee KP, Simpson SJ, Clissold FJ, Brooks R, Ballard JWO, Taylor PW, Soran N, Raubenheimer D. 2008. Lifespan and reproduction in Drosophila: New insights from nutritional geometry. Proceedings of the National Academy of Sciences of the United States of America 105(7):2498–2503. DOI: 10.1073/pnas.0710787105.
- Liu Y, Zhu F, Shen Z, Moural TW, Liu L, Li Z, Liu X, Xu H. 2021. Glutaredoxins and thioredoxin peroxidase involved in defense of emamectin benzoate induced oxidative stress in Grapholita molesta. Pesticide Biochemistry and Physiology 176:104881. DOI: 10.1016/j.pestbp.2021.104881.
- Lorenz S, Stark K. 2015. Saving the honeybees in Berlin? A case study of the urban beekeeping boom. Environmental Sociology 1(2):116–126. DOI: 10.1080/23251042.2015.1008383.
- Migdal P, Bieńkowski P, Cebrat M, Berbeć E, Plotnik M, Murawska A, Sobkiewicz P, Łaszkiewicz A, Latarowski K. 2023. Exposure to a 900 MHz electromagnetic field induces a response of the honey bee organism on the level of enzyme activity and the expression of stress-related genes. PLoS One 18(5):e0285522. DOI: 10.1371/journal.pone.0285522.
- Migdał P, Murawska A, Bieńkowski P, Strachecka A, Roman A. 2021. Effect of E-field at frequency 50 Hz on protein, glucose, and triglycerides concentration in honeybee hemolymph. European Zoological Journal 88(1):1170–1176. DOI: 10.1080/24750263.2021.2004247.
- Migdał P, Murawska A, Roman A. 2020. A modified standardized method to extract and store insect hemolymph with use of a glass capillary. Journal of Apicultural Science 64(1):165–168. DOI: 10.2478/jas-2020-0004.
- Migdał P, Roman A, Strachecka A, Murawska A, Bieńkowski P. 2020. Changes of selected biochemical parameters of the honeybee under the influence of an electric field at 50 Hz and variable intensities. Apidologie 51(6):956–967. DOI: 10.1007/s13592-020-00774-1.
- Moritz B, Crailsheim K. 1987. Physiology of protein digestion in the midgut of the honeybee (Apis mellifera L.). Journal of Insect Physiology 33(12):923–931. DOI: 10.1016/0022-1910(87)90004-7.
- Odemer R, Odemer F. 2019. Effects of radiofrequency electromagnetic radiation (RF-EMF) on honey bee queen development and mating success. Science of the Total Environment 661:553–562. DOI: 10.1016/j.scitotenv.2019.01.154.
- Papachristoforou E, Lambadiari V, Maratou E, Makrilakis K, Sardu C. 2020. Association of glycemic indices (hyperglycemia, glucose variability, and hypoglycemia) with oxidative stress and diabetic complications. Journal of Diabetes Research 2020:1–17. DOI: 10.1155/2020/7489795.
- Salkin PEH. 2012. It’s all the buzz: Regulating neighborhood beehives. Boston College Environmental Affairs Law Review 39:55–70.
- Sies H. 2020. Oxidative stress: Concept and some practical aspects. Antioxidants 9(9):852. DOI: 10.3390/antiox9090852.
- Skowronek P, Strachecka A. 2023. Cannabidiol (CBD) supports the honeybee worker organism by activating the antioxidant system. Antioxidants 12(2):279. DOI: 10.3390/antiox12020279.
- Tawfik AI, Ahmed ZH, Abdel-Rahman MF, Moustafa AM. 2020. Influence of winter feeding on colony development and the antioxidant system of the honey bee, Apis mellifera. Journal of Apicultural Research 59(5):752–763. DOI: 10.1080/00218839.2020.1752456.
- Thompson SN. 2003. Trehalose—The insect ‘blood’sugar. Advances in Insect Physiology 205–285. https://books.google.pl/books?hl=pl&lr=&id=EogT_YtQREAC&oi=fnd&pg=PA205&dq=Thompson,+S.+N.+(2003).+Trehalose%E2%80%94the+insect+%E2%80%98blood%E2%80%99sugar.+Advances+in+insect+physiology,+31(Supplement+C),+205-285.&ots=svwPzeESeH&sig=AZtq10FbJns8yu0akO18HcouQtY&redir_esc=y#v=onepage&q&f=false
- Toribio D, Joseph W, Thielens A. 2022. Near field radio frequency electromagnetic field exposure of a Western Honey Bee. IEEE Transactions on Antennas & Propagation 70(2):1320–1327. DOI: 10.1109/TAP.2021.3111286.
- Vijver MG. 2014. Investigating short-term exposure to electromagnetic fields on reproductive capacity of invertebrates in the field situation. Electromagnetic Biology and Medicine 33(1):21–28. DOI: 10.3109/15368378.2013.783846
- Vilić M, Žura Žaja I, Tkalec M, Štambuk A, Šrut M, Klobučar G, Malarić K, Tucak P, Pašić S, Tlak Gajger I. 2021. Effects of a radio frequency electromagnetic field on honey bee larvae (Apis mellifera) differ in relation to the experimental study design. Veterinarski Arhiv 91(4):427–435. DOI: 10.24099/vet.arhiv.1321.
- Weinberg KP, Madel G. 1985. The influence of the mite Varroa jacobsoni Oud. On the protein concentration and the haemolymph volume of the brood of worker bees and drones of the honey bee Apis mellifera L. Apidologie 16(4):421–436. DOI: 10.1051/apido:19850407.
- Wilson JK, Ruiz L, Davidowitz G. 2019. Dietary protein and carbohydrates affect immune function and performance in a specialist herbivore insect (Manduca sexta)*. Physiological and Biochemical Zoology 92(1):58–70. DOI: 10.1086/701196.
- Xiao F, Wang J, Liu H, Zhuang M, Wen X, Zhao H, Wu K. 2023. Effects of dietary protein levels on growth, digestive enzyme activity, antioxidant capacity, and gene expression related to muscle growth and protein synthesis of juvenile greasyback shrimp (metapenaeus ensis). Animals 13:3886. DOI: 10.3390/ani13243886.
- Zhou G, Pennington JE, Wells MA. 2004. Utilization of pre-existing energy stores of female Aedes aegypti mosquitoes during the first gonotrophic cycle. Insect Biochemistry and Molecular Biology 34(9):919–925. DOI: 10.1016/j.ibmb.2004.05.009.
- Ziegelberger G. 2009. Guidelines on limits of exposure to static magnetic fields. Health Physics 96. DOI: 10.1097/01.HP.0000343164.27920.4a.
- Ziegler R, Ibrahim MM. 2001. Formation of lipid reserves in fat body and eggs of the yellow fever mosquito, Aedes aegypti. Journal of Insect Physiology 47(6):623–627. DOI: 10.1016/S0022-1910(00)00158-X.
- Zmyślony M, Bieńkowski P, Bortkiewicz A, Karpowicz J, Kieliszek J, Politański P, Rydzyński K. 2020. Protection of the population health from electromagnetic hazards − Challenges resulting from the implementation of the 5G network planned in Poland. Medycyna pracy 71(1):105–113. DOI: 10.13075/mp.5893.00867.