Abstract
The warming climate is giving migratory birds new opportunities to extend their breeding ranges. These expansions may have a significant impact on other local bird communities. Here, we investigate the nest site selection of Mute Swan Cygnus olor, and the expanding Whooper Swan Cygnus cygnus. The study aims to assess the differences in habitat use by these two swan species in a lowland anthropogenic landscape. It examines the number of breeding pairs and the surface areas of nesting zones in different habitat types. The field procedures involved the use of unmanned aerial vehicles (UAVs) to survey and locate nests, with geotagged photographs taken to map nest locations. Our results show that Mute Swans primarily occupy oxbows, while Whooper Swans predominantly nest in pond complexes. Moreover, GLM models indicate that Mute Swans tend to choose open areas within a radius of 200 m around the nests, whereas Whooper Swans avoid built-up areas, often choosing forested sites within a radius of 500 m of their nests. These findings suggest that the expansion of the Whooper Swan population has led to changes in the breeding behaviour and habitat use of sympatric Mute Swan populations. The territorial behaviour and aggression of Whooper Swan may be driving Mute Swan away from fishpond habitats. We conclude that interactions between the two swan species are dynamic and have not yet stabilized, so this is an issue that needs to be closely monitored. Understanding these dynamics is essential for the effective conservation and management of wetland habitats in the face of ongoing climate change and shifting breeding ranges of birds.
Key policy highlights
Species range shifts: climate change causes shifts in bird breeding ranges, impacting species interactions. The expanding Whooper Swan’s competition with Mute Swan exemplifies this process.
Selection of new habitat types: as it expands, the Whooper Swan competes with the Mute Swan for attractive breeding habitat.
Differences in habitat choice: Whooper Swan most frequently chooses fishponds surrounded by forests, whereas Mute Swan nests within more open area, closer to human settlements, frequently on oxbows.
Introduction
Climate change is causing the breeding ranges of multiple species to shift (Devictor et al. Citation2008; Gillings et al. Citation2015; Hu et al. Citation2020) – towards higher elevations or polewards (Kearney et al. Citation2010). The most obvious direction within the Northern Hemisphere is northwards, as most species try to track their optimal conditions (Martinez-Meyer Citation2005; Hitch & Leberg Citation2007; McDonald et al. Citation2012; Lehikoinen et al. Citation2013). However, for migratory birds, the pattern of range shift may have a species-specific character (Gillings et al. Citation2015; Marjakangas et al. Citation2023) and may impact the phenology and distances of annual migrations (Lehikoinen et al. Citation2006; Lehikoinen & Jaatinen Citation2012; Potvin et al. Citation2016). The case of a species shifting its breeding range in another direction, especially southwards, is atypical (Kopij Citation2011; Ławicki et al. Citation2013). Such a situation may occur if a migratory species finds convenient wintering conditions at higher latitudes than before the climate changed, with the result that the migration distances become shorter (Dudzik et al. Citation2015).
The climate in east-central Europe has warmed up in recent years – winters are mild with few frosty days (Owczarek & Filipiak Citation2016) – and this trend is set to continue (Kundzewicz & Matczak Citation2012). This, in turn, will offer migratory species an opportunity to avoid energy-consuming seasonal migrations, since the new local climate conditions will allow some of them to both breed and overwinter. One such species is the Whooper Swan Cygnus cygnus. Individuals that used to breed only in northern latitudes spent the winters in distant regions of temperate climate zones (Luigujõe et al. Citation2002; Brazil Citation2003). In recent decades, however, the migratory behaviour of these birds has changed. Nowadays, subpopulations of these swans have sprung up in new breeding areas, where they undertake only local seasonal migrations (Dudzik et al. Citation2015). Climate change is thus a possible reason why the Whooper Swans’ breeding range is shifting south. This situation must be having an impact on local water bird communities, as the Whooper Swan is considered to be an aggressive and highly territorial species, which can even kill the juveniles of other species (Wood et al. Citation2020). It shares a common type of nesting habitat with the Mute Swan Cygnus olor (Butkauskas et al. Citation2012), which has been breeding in central-eastern Europe for far longer than its new competitor.
At present, Whooper Swan and Mute Swan should be regarded as sympatric species. This is a situation that has evolved during the last few decades, ever since the Whooper Swan began to establish new breeding populations. Before that, these two species were considered to be allopatric: the Whooper Swan was originally associated with the boreal zone in northern Asia, northern Europe and Iceland (Cramp & Simmons Citation1977), whereas the Mute Swan inhabited the temperate zone of Europe. Their breeding ranges were thus quite separate (Butkauskas et al. Citation2012). Nevertheless, the current relationships between these species have become highly dynamic: the Mute Swan’s population is stable, whereas that of the Whooper Swan is continuing to increase rapidly. This may mean that both species will have to adapt to new types of habitat and compete for the most optimal ones, because densities and levels of competition change from one year to another (Morris Citation2011). Even though both swans are considered to be non-selective breeding species (Brazil Citation1981; Gayet et al. Citation2011), they appear to compete for specific habitats, especially in man-made ones like fishpond complexes (Dudzik et al. Citation2014). Such ponds are regarded as important habitats for waterbirds, especially in anthropogenic landscapes, where there are not enough natural wetlands (Sebastián-González et al. Citation2010). Moreover, it has been demonstrated that the range expansion of some bird species also involves a widening of habitat options, which in turn increases the pace of colonization (Thomas et al. Citation2001; Parmesan & Yohe Citation2003)
The aim of our study is to assess the differences in current habitat use by Whooper Swan and Mute Swan in the lowlands of east-central Poland. We formulated two hypotheses:
1) there are differences in the numbers of breeding pairs of both species inhabiting ponds and oxbows, the most important habitat types available in the study area.
In this case, we have assumed that the Whooper Swan would inhabit mainly fishponds, where this increasing in number species has frequently reproduced over the last decade (Wieloch et al. Citation2014).
2) the surface areas of habitat components differ in the nesting areas. We expected that the Whooper Swan inhabits rather remote and secretive places surrounded by forest, according to its native habit (Brazil Citation2003), while the Mute Swan does not show such demands.
Materials and methods
Study area
The survey was conducted in east-central Poland and covered an area of approximately 6,000 square kilometers (22.09071 E, 52.52924 N). Within this area, the research focused on two types of swan nesting sites: oxbows (94 monitored water objects with an area of 1 345 ha) and pond complexes (69 monitored facilities with an area of 5 019 ha). The first type was represented by the valley of the River Bug between the town of Siemiatycze and its confluence with the River Narew. The whole of that area is protected as a Natura 2000 Special Protection Area (SPA), the Birds Directive SPA of Dolina Dolnego Bugu PLB140001, i.e. the Lower Bug Valley. There are various types of land cover in the vicinity of the river banks, but the dominant ones are meadows and pastures (41%), farmland (25%) and forests (20%). The riverbed is not regulated – it meanders strongly – and the fluvial terrace has persisted in an almost natural form. These features imply a complex structure, the existence of a floodplain where surface water levels may fluctuate considerably, and also oxbow lakes. The largest of these has an area of c. 36 ha. Their shorelines and alluvial sediments are usually bare or support willow and poplar woodland or scrub. There are many patches of reeds and sedges. The second type of nesting area consists of pond complexes, all situated in the provinces of Mazovia and Podlasie. The ponds are mostly given over to fish farming or utilized in other ways. Most of the fish bred in the ponds are carp Cyprius carpio, so the water is seasonally drained from selected ponds in connection with spawning and the upkeep of the vegetation. The vegetation cover varied among the pond complexes and also within particular ones. The most common species were reed Phragmites australis, reedmace Typha sp. and sedge Carex sp.
Field procedures
The field survey was conducted from the 1st of April to the 2nd of June 2022. Both swan species live in a wide range of habitats (Gayet et al. Citation2011; Boiko et al. Citation2014), so the study area covered a wide diversity of wetland habitats, including natural and artificial ones. During the nest search, we focused on the two most important habitat types: oxbows and fishponds. The database containing previous records (for the last five years preceding this research), especially of confirmed nesting, was used to predict areas where swans’ nests were likely to occur. The entire study area was meticulously searched for breeding pairs of swans during the breeding season of both species, which in this region usually lasts from early April to late May. During the first phase of the field survey, from 1st to 8th April, we observed early territorial behaviour of swans such as mating of individuals into pairs, displays or ruffled posture of males. At that time, we noted the locations of pairs exhibiting such behaviours, expecting them to start breeding soon. We began searching for nests on 16th April. We first visited the sites where the pairs mentioned were spotted and then searched the entire remaining study area. This phase was completed on 10th May. To verify the appearance of new nests, a second check of the entire study area took place between 15th May and 2nd June. We visited some uncertain territories (pairs seen but not yet nesting) more frequently until the presence or absence of an occupied nest was confirmed. If neither eggs nor an incubating individual was observed, only the non-breeding pair was noted (not included further in the database). We carried out the search using UAVs (Unmanned Aerial Vehicles). This method has already proven useful and highly efficient in bird surveys, as it enables large or inaccessible areas to be scanned in a short time by just a few observers (Sikora & Marchowski Citation2023). It is also considered to be as accurate or even more so than a standard ground-based survey (Hodgson et al. Citation2018; Valle & Scarton Citation2021). Usually, UAVs do not disturb the birds so much, as long as they are used properly (Borrelle & Fletcher Citation2017; Marchowski Citation2021; Sikora & Marchowski Citation2023). A recent study assessed the reactions of swans to drones: Mute Swans did not react at all, but some Whooper Swans were frightened when a drone flew within 40 m (Marchowski Citation2021), although panicked flight reactions were still infrequent in the latter species (Sikora & Marchowski Citation2023). Based on this knowledge, we were able to determine the appropriate altitude range of our flights so as not to disturb the birds. We used three types of UAVs (DJI Phantom 3, Phantom 4 and Mavic Mini), flying them at heights of 50–80 m AGL (Above Ground Level), depending on current local conditions and the type and height of the vegetation.
In open terrain, the drone was flown higher in order to cover a large area with a small number of flights, as the size and colour of swans makes them easily visible (also to the camera) from a distance. Each time when an individual was detected, the drone hovered above it to take a picture. For each nesting pair, the location of their nest was mapped with the use of geotagged photographs taken exactly above the nesting platform. This is the easiest way to obtain the precise coordinates without disturbing the birds, while retaining a high spatial precision. If the observer could not see the pair with binoculars, the drone was lowered in order to identify the species of swan. The average distance between neighboring nests was 10.4 km (SD = 9.56) for the Whooper Swan and 1.5 km (SD = 2.61) for the Mute Swan.
The map of the nest locations as well as the further geoprocessing operations were performed in QGIS software, which we found to be suitable for this task. All the geotagged nest photos were imported and converted into point layers for both Mute Swan and Whooper Swan. For each nest, circular buffers 200 and 500 m in radius were generated as polygon layers. These two zones were adopted due to the wide variation in the territories of the two species (see Cramp & Simmons Citation1977; Brazil Citation2003; Włodarczyk & Minias Citation2016). It was assumed that an area with a radius of 200 m (12.5 hectares) could mostly guarantee a suitable breeding area for both swan species during the first nesting period (nest building and egg incubation). An area with a radius of 500 m around the nest (78.5 ha) on the other hand was designated for the second nesting period, during which swan families move greater distances from the nest. Within these zones, each type of ground cover, such as reedbeds, woodland or water surface, was drawn manually on the basis of an orthophotograph generated from satellite images taken during spring 2022, thus representing the land cover at the time of the study. We mainly used Sentinel-2 (Sentinel Playground) satellite images taken on May 9th 2022. We also used images from Landsat 8 satellite (Google Earth) as supporting images. The scale of the map was 1:2,000, and the minimum size of the mapped habitat was 100 m2. For each nest, the area of each mentioned land cover type was calculated within each of the two generated nest buffer zones (200 and 500 m) as a set of output geoprocessing polygon layers. The accuracy of such computerized geoprocessing operations is very high, and there is no ultimate loss of precision. The following parameters were obtained: 1) area of open terrain; 2) areas of forest and groups of trees; 3) area of built-up land; 4) area of aquatic vegetation and 5) surface area of open water ().
Table I. Factors employed for analysing differences between Mute Swan and Whooper Swan nest sites.
Statistical analyses
The difference in the number of Mute Swan and Whooper Swan nests located in oxbow lakes or fishponds were evaluated using a chi2 test. A general linear model (GLM) with logit link function and binomial error variance was used to compare the species’ macro-habitat preferences. The dependent variable was the species pair being compared (binomial variable: 0 = Whooper Swan, 1 = Mute Swan). The models were constructed using the glm function in the lme4 package for R (Bates et al. Citation2015). The VIF procedure was applied and predictors with high and moderate multicollinearity were identified. A variable with a high VIF value (>5) was noted for both models. After removing one predictor, which was aquatic vegetation area, the remaining four predictors had the lowest VIF value (<2.8) and were included in the models. Model selection was performed using the information-theoretic approach (AIC) (Burnham & Anderson Citation2002). All possible combinations of the global model were analysed using the dredge function in the MuMln package for R (Bartoń Citation2016). Only the models with AIC ≤ 2 are discussed, because they are treated as being equally supported (Burnham & Anderson, Citation2002). Multiple competing models were assessed with regard to their fit to the data using AIC as the leading criterion, and the models with the lowest AIC value were selected as the best fitting models. All the data were analysed in the R environment (R Core Team Citation2019). The values reported are the mean ±1 SE. Only those results with a probability of α ≤ 0.05 were assumed to be statistically significant.
Results
In the area of oxbow habitats, we confirmed 68 Mute Swan nests (5.06 pair/km2) but only 2 Whooper Swan nests (0.15 pair/km2). The situation was different within the pond complexes, where we located 21 Whooper Swan nests (0.42 pair/km2) and 22 Mute Swan nests (0.44 pair/km2). The difference in the number of nests between the two habitats was significant (chi2 = 31.96, p < 0.001, df = 1). Nesting of both species was found in only 4 fishponds.
Models containing five macrohabitat predictors were created separately for the two radii around the swans’ nests on the basis of Akaike’s information criterion (). For the 200 m radius, the best model describing the differences between the two swan species contained just one factor – the area of open terrain – so the other four macrohabitat parameters were omitted from the next step in the analysis. For the 500 m radius, however, the best model included two habitat factors, i.e. the area of built-up land and the area of forests. The best GLM model (R-square = 0.12) created for smaller areas showed that the differences between territories in open terrain was significant (). Mute Swans tended to choose larger open habitats than Whooper Swans within a radius of 200 m around their nests (). In the best model for the 500 m radius (R-square = 0.54), the area of built-up land area and the area of woodland were significant (). Whooper Swan breeding territories contained a larger area of forest but a smaller area of built-up land than Mute Swan territories ().
Figure 1. Mean area and 95% confidence limits (whiskers) of open terrain within a radius of 200 m (open200), built-up land within a radius of 500 m (build500) and forest within a radius of 500 m (forest500) around Whooper Swan and Mute Swan nests.
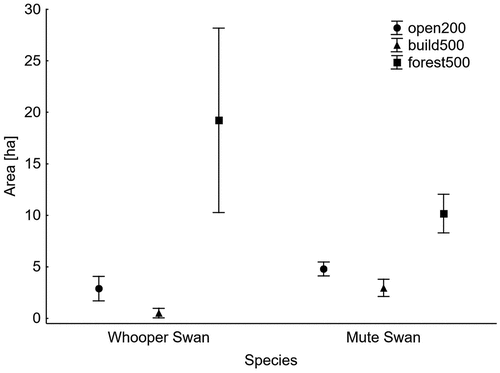
Table II. Results of models describing the differences in macrohabitat parameters between the two swan species. Degrees of freedom (df), model log-likelihood (LL), corrected AIC criterion (AICc), difference between the model and the best model in the data set (Δ AICc), and weight for the model (AICcwt) are shown.
Table III. Estimated model coefficients for the best binomial general linear models comparing activity parameters among the macrohabitat parameters between the two swan species.
Discussion
The results of our research indicate that Mute Swans mostly occupy oxbows in river valleys. A strong increase in the abundance of Mute Swan in this habitat type and an increase in the number of breeding pairs of Whooper Swan in the fishpond have been observed in the study area over the past two decades (Kasprzykowski et al. Citation2017; Goławski Citation2022). Our present findings may be an illustration of the changing behavioural ecology of both swan species, now breeding sympatrically. We found Whooper Swan nests almost exclusively within fishpond complexes. This could indicate the possibility of fierce competition for habitat between the two species, with the Whooper Swan displacing the Mute Swan from ponds where the latter has readily nested in the past (Dudzik et al. Citation2014, own observ.). Whooper Swans are known to defend larger territories than Mute Swans (Cramp & Simmons Citation1977). The former are also more aggressive and can expel their competitors. On the other hand, mixed pairs of both species have been observed, and in a few cases have even bred successfully (Kampe-Persson & Boiko Citation2011). Hence, another possible consequence of such proximity is hybridization.
Our results could be interpreted as evidence that Mute Swans are more flexible in terms of breeding habitat selection than Whooper Swans, as the former are more often present in both types of habitat. However, this feature may be due to the Whooper Swan’s aggression towards its competitor and ability to take over its breeding territories (Kampe-Persson & Boiko Citation2011; Dudzik et al. Citation2014). Nevertheless, a division has begun to emerge, with the Whooper Swan selecting and occupying mainly ponds, probably the most attractive habitat. At the same time, most breeding pairs of the Mute Swan were observed on oxbows and fishponds not occupied by the Whooper Swan. In regions without fishpond complexes, Whooper Swan range expansion is facilitated by the presence of beaver ponds (Sikora et al. Citation2022). The reason for such a difference in habitat choice of Whooper Swans in our study area compared to other new breeding areas in north-eastern Poland is habitat availability: beaver ponds are a much more common habitat type in that region. However, in our study area there are no natural waterbodies such as glacial lakes or large beaver ponds. Therefore, the only type of standing water is the relatively numerous oxbow lakes and artificial ponds.
Nest-site selection by waterbirds often involves nest concealment and water depth as key features influencing breeding success (Polak et al. Citation2008; Athamnia et al. Citation2015; Zaremba et al. Citation2020). Studies have shown that predation rates for wetland nesters decreased with increasing water depth (Purger & Mészáros Citation2006). Therefore, it is very likely that oxbows do not provide stable conditions for swans during the entire breeding season because their water levels may vary (own observ.). This could turn out to be significant as the lack of snow melt in spring and regular early droughts destabilize river valley environments somewhat (Bradley Citation2002). This implies that a nest, inaccessible to predators in early spring, may become easily reachable by the time of hatching. On the other hand, fishponds may provide more stable and safe conditions for nestlings as the water level is man-managed. Moreover, swans use fish feed as an additional food source. In general, fish farms with high coverage of sporadically mowed emergent vegetation are known to exhibit bird-friendly management in the study area (Kasprzykowski & Polak Citation2013). In fact, the productivity of Mute Swans was found to be lower in river valley than fishpond complexes (Włodarczyk & Wojciechowski Citation2001). This explains why fishponds appear to be the preferred type of habitat for nesting swans of both species and hence the object of competition.
We confirmed the expected differences in habitat variables of the nesting areas. These differences are statistically significant, but not yet extreme, since neither species is regarded as strongly selective in terms of habitat choice (Brazil Citation2003). Our study shows that in comparison to Mute Swans, Whooper Swans choose locations with less built-up land and more forest cover. Therefore, sites chosen by the latter seem to be more remote and peaceful, thus corresponding to their nesting habitats within their original breeding range in northern and north-eastern Europe where Whooper Swan nests are situated mostly on small waterbodies, usually surrounded by forest (Boiko & Kampe-Persson Citation2013). The human population density of these territories is also much lower, thus Whooper Swans may not yet have become accustomed to human habitations. On the other hand, Mute Swans breed regularly in the highly populated regions of east-central Europe, where it was introduced long ago (Wieloch et al. Citation2004). In contrast to the Whooper Swan, this species used to breed primarily in the steppes of Asia, which is a non-forested, open country (Brazil Citation2003; Wieloch et al. Citation2004). Our study suggests that the Mute Swan prefers more open areas and avoids more thickly wooded areas in the vicinity of the nest.
Developing a habitat selection strategy is a dynamic and very complex process, influenced by such factors as population density, inter- and intraspecific competition, and also breeding strategy and adaptability (Morris Citation2011). In the case of breeding swans of both species, this process has recently become disrupted. The Whooper Swan expands its range by conquering new territories where the available habitat types are different from those it chose before, and in the process its population density increases. Such expansion therefore demands a high level of plasticity from this species (Gayet et al. Citation2011). Our results suggest that Whooper Swans tended to nest in more thickly wooded areas, but this could also be a temporary behaviour that might change at a later stage of this process. On the other hand, Mute Swan nests were situated in more open areas, moreover, in habitats prone to desiccation, perhaps rendering the nests more accessible to predators. This is another factor which may impact the Mute Swan’s inferior position in the competition between the two species.
Conclusion
This study showed that the Whooper Swan, which is expanding its breeding range, chooses habitats that suit its requirements in its native, northern latitudes, which are mostly ponds surrounded by woodland. The Mute Swan, on the other hand, tends to nest closer to human settlements, often in a river valley. This difference may indicate a division in habitat type choice between the two species, that is now beginning to emerge. As the Whooper Swan population continues to grow, different scenarios of habitat competition are possible. Regardless of the final result, this is a highly topical issue and further changes are to be expected.
Acknowledgments
We are grateful to Peter Senn for the English language editing and Przemysław Obłoza for help in statistical analyses.
Disclosure statement
No potential conflict of interest was reported by the author(s).
Data availability statement
Dataset supporting reported results can be found at the Mendeley Data repository: https://doi.org/10.17632/jwv3prnfh4.1.
Additional information
Funding
References
- Athamnia M, Samraoui F, Kelailia B, Rouabah A, Alfarhan AH, Samraoui B. 2015. Nest-site selection and reproductive success of the little grebe Tachybaptus ruficollis in northeast Algeria. Ardeola 62(1):113–124. DOI: 10.13157/arla.62.1.2015.113.
- Bartoń K. 2016. MuMln: Multi-Model Inference. R Package Version 1.15.6. Available: https://cran.r-project.org/web/packages/MuMIn. Accessed Dec 2019 15.
- Bates D, Maechler M, Bolker B, Walker S. 2015. Fitting linear mixed-effects models using lme4. Journal of Statistical Software 67:1–48.
- Boiko D, Kampe-Persson H. 2013. Breeding whooper swans Cygnus cygnus in Latvia, 1973–2009. Wildfowl 60(60):168–177.
- Boiko D, Kampe-Persson H, Morkunas J. 2014. Breeding whooper swans Cygnus cygnus in the Baltic states, 1973-2013: Result of a re-colonisation. Wildfowl 64:207–216.
- Borrelle SB, Fletcher AT. 2017. Will drones reduce investigator disturbance to surface-nesting seabirds? Marine Ornithology 45:89–94.
- Bradley C. 2002. Simulation of the annual water table dynamics of a floodplain wetland, Narborough Bog, UK. Canadian Journal of Fisheries and Aquatic Sciences 261(1–4):150–172. DOI: 10.1016/S0022-1694(02)00012-4.
- Brazil M 1981. The Behavioural Ecology of the Whooper Swan, Doctoral Thesis.
- Brazil M. 2003. The Whooper Swan. London, UK: T.& A.D. Poyser.
- Burnham KP, Anderson DR. 2002. Model selection and multimodel inference: A practical information-theoretic approach. New York, NY: Springer. pp. 1–454.
- Butkauskas D, Švažas S, Tubelytė V, Morkūnas J, Sruoga A, Boiko D, Paulauskas A, Stanevičius V, Baublys V. 2012. Coexistence and population genetic structure of the whooper swan Cygnus cygnus and mute swan Cygnus olor in Lithuania and Latvia. Open Life Sciences 7(5):886–894. DOI: 10.2478/s11535-012-0065-9.
- Cramp S, Simmons KEL. 1977. The birds of the Western palearctic. Vol. 1. Oxford: Oxford Univ. Press. pp. 1–722.
- Devictor V, Julliard R, Couvet D, Jiguet F. 2008. Birds are tracking climate warming, but not fast enough. Proceedings of the Royal Society B: Biological Sciences 275(1652):2743–2748. DOI: 10.1098/rspb.2008.0878.
- Dudzik K, Polakowski M, Wilniewczyc P, Kaczorowski G, Sułek J, Dobosz R. 2014. Population of the Whooper Swan Cygnus cygnus in the Świętokrzyskie region (SE Poland) at the beginning of the 21 st century. Chrońmy Przyrodę Ojczystą 70:32–48. (In Polish with English summary).
- Dudzik K, Włodarczyk R, Czyż S, Polakowski M. 2015. Unusual migratory behavior in a newly established subpopulation of Whooper Swan (Cygnus cygnus) breeding in the highlands of Poland. Ornis Fennica 92(4). DOI: 10.51812/of.133880.
- Gayet G, Eraud C, Benmergui M, Broyer J, Mesleard F, Fritz H, Guillemain M. 2011. Breeding Mute Swan habitat selection when accounting for detectability: A plastic behaviour consistent with rapidly expanding populations. European Journal of Wildlife Research 57(5):1051–1056. DOI: 10.1007/s10344-011-0518-x.
- Gillings S, Balmer DE, Fuller RJ. 2015. Directionality of recent bird distribution shifts and climate change in Great Britain. Global Change Biology 21(6):2155–2168. DOI: 10.1111/gcb.12823.
- Goławski A. 2022. Changes in the abundance of selected breeding species at the fishponds of Siedlce in 1997–2022. Ornis Polonica 63:383–390. (In Polish with English summary).
- Hitch AT, Leberg PL. 2007. Breeding distributions of North American bird species moving north as a result of climate change. Conservation Biology 21(2):534–539.
- Hodgson JC, Mott R, Baylis SM, Pham TT, Wotherspoon S, Kilpatrick AD, Raja Segaran R, Reid I, Terauds A, Koh LP 2018. Drones count wildlife more accurately and precisely than humans. Methods in Ecology and Evolution 9(5):1160–1167. DOI: 10.1111/2041-210X.12974.
- Hu R, Gu Y, Luo M, Lu Z, Wei M, Zhong J, Acharya BK. 2020. Shifts in bird ranges and conservation priorities in China under climate change. PLoS One 15(10):e0240225. DOI: 10.1371/journal.pone.0240225.
- Kampe-Persson H, Boiko D. 2011. Occurrence of swan hybrids around the Baltic Sea—An outcome of range expansions? Ornis Svecica 21(1):45–54. DOI: 10.34080/os.v21.22609.
- Kasprzykowski Z, Dmoch A, Goławski A, Kozik R, Mitrus C. 2017. Changes in the abundance of waterbirds in the Narew and the Bug Valleys. Ornis Polonica 58:1–11. (In Polish with English summary).
- Kasprzykowski Z, Polak M. 2013. Habitat quality and breeding parameters in relation to female mating status in the polygynous Eurasian Bittern Botaurus stellaris. Journal of Ornithology 154(2):403–409. DOI: 10.1007/s10336-012-0904-5.
- Kearney MR, Wintle B, Porter WP. 2010. Correlative and mechanistic models of species distribution provide congruent forecasts under climate change. Conservation Letters 3(3):203–213. DOI: 10.1111/j.1755-263X.2010.00097.x.
- Kopij G. 2011. Population and range expansion of forest boreal owls (Glaucidium passerinum, Aegolius funereus, Strix uralensis, Strix nebulosa) in East-Central Europe. Vogelwelt 132(4):207–214.
- Kundzewicz ZW, Matczak P. 2012. Climate change regional review: Poland. Wiley Interdisciplinary Reviews: Climate Change 3(4):297–311. DOI: 10.1002/wcc.175.
- Ławicki Ł, Abramcuk AV, Domashevsky SV, Paal U, Solheim R, Chodkiewicz T, Woźniak B. 2013. Range extension of Great Grey Owl in Europe. Dutch Birding 35:145–154.
- Lehikoinen A, Jaatinen K. 2012. Delayed autumn migration in Northern European waterfowl. Journal of Ornithology 153(2):563–570. DOI: 10.1007/s10336-011-0777-z.
- Lehikoinen A, Jaatinen K, Vähätalo AV, Clausen P, Crowe O, Deceuninck B, Hearn R, Holt CA, Hornman M, Keller V, Nilsson L. 2013. Rapid climate driven shifts in wintering distributions of three common waterbird species. Global Change Biology 19(7):2071–2081. DOI: 10.1111/gcb.12200.
- Lehikoinen A, Kilpi M, Ost M. 2006. Winter climate affects subsequent breeding success of common eiders. Global Change Biology 12(7):1355–1365. DOI: 10.1111/j.1365-2486.2006.01162.x.
- Luigujõe L, Kuresoo A, Leivits A. 2002. Numbers and distribution of Whooper Swans breeding, wintering and on migration in Estonia, 1990–2000. Waterbirds 25:61–66.
- Marchowski D. 2021. Drones, automatic counting tools, and artificial neural networks in wildlife population censusing. Ecology and Evolution 11(22):16214. DOI: 10.1002/ece3.8302.
- Marjakangas EL, Bosco L, Versluijs M, Xu Y, Santangeli A, Holopainen S, Mäkeläinen S, Herrando S, Keller V, Voříšek P, Brotons L. 2023. Ecological barriers mediate spatiotemporal shifts of bird communities at a continental scale. Proceedings of the National Academy of Sciences 120(23):e2213330120. DOI: 10.1073/pnas.2213330120.
- Martinez-Meyer E. 2005. Climate change and biodiversity: Some considerations in forecasting shifts in species potential distributions. Biodiversity Informatics 2:42–55. DOI: 10.17161/bi.v2i0.8.
- McDonald KW, McClure CJ, Rolek BW, Hill GE. 2012. Diversity of birds in eastern North America shifts north with global warming. Ecology and Evolution 2(12):3052–3060. DOI: 10.1002/ece3.410.
- Morris DW. 2011. Adaptation and habitat selection in the eco-evolutionary process. Proceedings of the Royal Society B: Biological Sciences 278(1717):2401–2411. DOI: 10.1098/rspb.2011.0604.
- Owczarek M, Filipiak J. 2016. Contemporary changes of thermal conditions in Poland, 1951–2015. Bulletin of Geography Physical Geography Series (10):31–50. DOI: 10.1515/bgeo-2016-0003.
- Parmesan C, Yohe G. 2003. A globally coherent fingerprint of climate change impacts across natural systems. Nature 421(6918):37–42. DOI: 10.1038/nature01286.
- Polak M, Kasprzykowski Z, Kucharczyk M. 2008. Micro-habitat nest preferences of the great bittern, Botaurus stellaris, on fishponds in central-eastern Poland. Annales Zoologici Fennici 45(2):102–108. DOI: 10.5735/086.045.0202.
- Potvin DA, Välimäki K, Lehikoinen A. 2016. Differences in shifts of wintering and breeding ranges lead to changing migration distances in European birds. Journal of Avian Biology 47(5):619–628. DOI: 10.1111/jav.00941.
- Purger JJ, Mészáros LA. 2006. Possible effects of nest predation on the breeding success of Ferruginous Ducks Aythya nyroca. Bird Conservation International 16(4):309–316. DOI: 10.1017/S0959270906000451.
- R Core Team. 2019. R: A language and environment for statistical computing. Vienna, Austria: R Foundation for Statistical Computing.
- Sebastián-González E, Sánchez-Zapata JA, Botella F. 2010. Agricultural ponds as alternative habitat for waterbirds: Spatial and temporal patterns of abundance and management strategies. European Journal of Wildlife Research 56(1):11–20. DOI: 10.1007/s10344-009-0288-x.
- Sikora A, Marchowski D. 2023. The use of drones to study the breeding productivity of Whooper Swan Cygnus cygnus. European Zoological Journal 90(1):193–200. DOI: 10.1080/24750263.2023.2181414.
- Sikora A, Marchowski D, Półtorak W. 2022. Use of beaver ponds by breeding Whooper Swans Cygnus cygnus and Mute Swans Cygnus olor at Górowo Heights (north-eastern Poland). Ornis Polonica 63:199–214.
- Thomas C, Bodsworth E, Wilson R, Simmons AD, Davies ZG, Musche M, Conradt L. 2001. Ecological and evolutionary processes at expanding range margins. Nature 411(6837):577–581. DOI: 10.1038/35079066.
- Valle RG, Scarton F. 2021. Monitoring the hatching success of gulls laridae and terns sternidae: A comparison of ground and drone methods. Acta Ornithologica 56(2):241–254. DOI: 10.3161/00016454AO2021.56.2.010.
- Wieloch M, Sikora A, Rohde Z, Neubauer G 2014. Expansion of breeding population of the Whooper Swan in Poland. 5th International Swan Symposium, February, Easton, Maryland. pp. 2–6.
- Wieloch M, Włodarczyk R, Czapulak A. 2004. Cygnus olor Mute Swan. BWP Update 6(1/2):1–27.
- Włodarczyk R, Minias P. 2016. Non-adaptive territory selection by a bird with exceptionally long parental care. PeerJ 4:e1852. DOI: 10.7717/peerj.1852.
- Włodarczyk R, Wojciechowski Z. 2001. The breeding ecology of the Mute Swan Cygnus olor in central Poland. Wildfowl 52(52):157–168.
- Wood KA, Ham P, Scales J, Wyeth E, Rose PE. 2020. Aggressive behavioral interactions between swans (Cygnus spp.) and other water birds during winter: a webcam-based study. Avian Research 11:1–16. DOI: 10.1186/s40657-020-00216-7.
- Zaremba U, Kasprzykowski Z, Golawski A. 2020. Effect of nest age and habitat variables on nest survival in Marsh Harrier (Circus aeruginosus) in a fishpond habitat. PeerJ 8:e9929. DOI: 10.7717/peerj.9929.