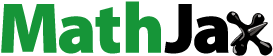
Abstract
Undoped and Al-doped ZnO nanoparticles are synthesized in supercritical isopropanol using zinc acetate di-hydrate, aluminum nitrates and methanol as precursor, dopant source and solvent, respectively. The atomic ratio [Al]/[Zn] is fixed to 3%. X-ray diffraction (XRD) results indicate that the synthesized ZnO nanoparticles possess hexagonal wurtzite structure. Al-doping of ZnO aerogel leads to the improvement of the crystalline quality due to the occupation of Zn2+ sites by Al3+ ions. The crystallites size is found to be 32 nm in pure ZnO and 50 nm in the doped aerogel. Fourier-transform infrared spectroscopy spectra reveal that the intensity of the absorption bands in ZnO decreases and the Zn-O vibration band position shifts towards higher wave numbers upon Al-doping. UV–Visible measurements show a reduction in the band gap and a shift towards long wavelength side of the absorbance with Al-doping. Scanning electron microscopy images reveal that aerogel grains are quasi-spherical in pure ZnO and have different and random forms of morphologies in the doped sample. Room temperature photoluminescence (PL) studies put into evidence that the PL emission peaks are influenced by the Al-doping, in particular, a decrease in the green emission at 535 nm is observed.
1. Introduction
Zinc oxide (ZnO) is a semiconductor material that has, at room temperature, a wide band gap (3.37 eV) and a large excitonic bonding energy (60 meV). ZnO nanostructures are used in various components intended for applications in optoelectronics, environment, purification, separation, photocatalysis and light emitting diodes (Alaeddine et al., Citation2009; Chang et al., Citation2004; Djouadi, Aksas, & Chelouche, Citation2010; Duan et al., Citation2015; Farhat, Halim, Abdullah, Ali, & Allam, Citation2015; Hasnidawani et al., Citation2016; Neves et al., Citation2012; Ridhuan, AbdulRazak, Lockman, & Abdul Aziz, 2012). The most thermodynamically stable crystallographic structure of ZnO is the hexagonal wurtzite form. The various properties of this material are highly dependent on the crystalline structure, size and morphology of the particles (Farhat et al., Citation2015). Various methods are used for ZnO nanoparticles synthesis and the most widespread are the sol-gel process (Hasnidawani et al., Citation2016), soft chemistry (Ridhuan et al., Citation2012) and chemical vapor phase synthesis (CVD) (Chang et al., Citation2004). In some cases, the drying process is carried out under supercritical conditions of solvents for better control of crystalline quality and size uniformity of nanoparticles (Ben Ayadi, El Mir, Djessas, & Alaya, Citation2007; Djouadi et al., Citation2010; Meddouri, Djouadi, Chelouche, Touam, & Chergui, Citation2014).The advantage of supercritical drying process lies in the use of environmentally friendly solvents and without any negative impact on the synthesized product, particularly in the pharmaceutical and biotechnology sectors. ZnO particles elaborated in supercritical solvents present best crystalline quality and lower size compared to others methods (Ben Ayadi et al., Citation2007; Meddouri et al., Citation2014). Also, this synthesis process leads to obtain aerogels consisting of nanoparticles building blocks networked together to form an open and highly porous structure (Gao, Sisk, & Hope-Weeks, Citation2007). When the properties of a semiconductor do not meet the requirements of the desired applications, doping with appropriate elements is one of the best ways to improve the performance of these materials. Doping of ZnO with transition elements such as aluminum (Ahmad et al., Citation2013; Ben Ayadi et al., Citation2007), gallium (Phan, Farag, Yakuphanoglu, & Chung, Citation2012), cadmium (Khayyat et al., Citation2012), copper (Singhal, Kaur, Namgyal, & Sharma, Citation2012) has been widely investigated to improve significantly its optical, electronic and structural properties. It has been demonstrated that Al-doping can also improve sensor sensitivity to gas molecules and decreases the thermal conductivity of ZnO material. In the majority of studies, it has been found that the ideal level of doping (Al/Zn atomic ratio) varies from 2% to 3% and constitutes an optimized balance between electrical (low resistivity), structural (without secondary phase) and optical (good transparency) properties of ZnO (Echresh & Shoushtari, Citation2013). However, it was reported that the doping disadvantage of nanostructures synthesized in supercritical drying conditions is the segregation of extrinsic atoms on the particles surface after the solvent evaporation (Sperling & Parak, Citation2010). Particularly, in Al-doped ZnO, the Zn2+ ions located in the wurtzite structure can be substituted by Al3+ ions and this leads to an exchange interaction between itinerant sp-band electrons or holes and the d-electron spins (Ghosh, Das, & Chattopadhyay, Citation2007).
In the literature, several works have demonstrated the dependence of the properties of Al-doped ZnO aerogels on the nature of the used supercritical fluid (Ahmad et al., Citation2013; Meddouri et al., Citation2014). Moreover, in a previous work (Mouzaia, Djouadi, Chelouche, & Hammiche, Citation2018), we have found that the crystal quality of Al-doped ZnO aerogels synthesized in supercritical ethanol deteriorates after the introduction of Al3+ ions in ZnO lattice. In this context, the present investigation is intended to enrich our understanding of the effects of Al-doping on the optical and structural properties of ZnO aerogels synthesized in others supercritical alcohols, particularly the isopropanol. So far, there have been only a few research reports on the study of the effects of Al-doping on the physical and chemical properties of ZnO aerogels synthesized in supercritical isopropanol.
Therefore, in this work, a sol-gel process followed by drying in supercritical conditions of isopropanol is used to elaborate pure and Al-doped (3 at. %) ZnO nanostructures. The effects of Al doping on structural, morphological and optical properties of the aerogels nanostructures are studied.
2. Experimental procedures
Undoped and Al-doped ZnO nanoparticles were synthesized using, in appropriate masses and volume, zinc acetate dihydrate (Zn (C2H3O2)2, 2H2O) as precursor, methanol as solution solvent and aluminum nitrates (Al(NO3)3, 9H2O) as doping element source. The mixture was kept under constant magnetic stirring for 15 min until a whitish solution is obtained. Then, the stable and homogeneous solutions underwent a drying at supercritical conditions of isopropanol (254 °C, 54 bars). For the doped solution, the [Al]/[Zn] atomic ratio was fixed to 3%. Both solutions are obtained under the same conditions. After reaching the supercritical conditions, the solvent was removed immediately and the autoclave was allowed to cool spontaneously to room temperature. The as-obtained powders were then characterized without additional treatments.
The crystal structure of the products was studied using a diffractometer type PanAlytical where the X-rays are produced from a radiation source CuKα (λ = 0.154 nm), with an acceleration voltage of 40 kV and a current of 30 mA. Fourier-transform infrared spectroscopy (FTIR) spectra were recorded with an Agilent 630-IR spectrometer. Scanning electron microscopy (SEM) micrographs were performed using a FEI Quanta 650 scanning electron microscope. A thermo-scientific evolution 201 UV–Vis spectrophotometer was used to investigate the optical properties. The photoluminescence (PL) spectra were recorded at room temperature with LabSolutions RF 6000 spectrophotometer using an excitation wavelength of 340 nm.
3. Results and discussion
The X-ray diffraction (XRD) patterns of pure and Al-doped ZnO aerogels synthesized in supercritical isopropanol are shown in . All recorded diffraction peaks can be indexed in the P63mc space group and are significant for hexagonal wurtzite structure. All the diffraction peaks were indexed and found to match with the JCPDS standard (No. 36-1451, where a0 = 3.249 Å and c0 = 5.206 Å) data of hexagonal wurtzite polycrystalline phase structure of pure ZnO nanoparticles. Peaks are quite sharp and intense, indicating high crystal quality of the samples. No additional peaks corresponding to metallic Al or other aluminum compound are detected in the XRD patterns, which suggest that Al3+ ions are incorporated in ZnO lattice. The intensity of all peaks increases after Al-doping. This observation indicates that the presence of Al atoms in ZnO lattice increases the crystal quality. Also, this improvement in crystalline quality maybe due to the fact that the majority of Zn2+ sites are occupied by Al3+ ions in ZnO lattice during the elaboration process. This result seems to be interesting since it was reported in literature that the crystal quality of ZnO nanoparticles is generally deteriorated after Al-doping (Neves et al., Citation2012; Phan et al., Citation2012). However, some authors have also observed the improvement of ZnO crystal quality by doping (Salam, Islam, & Akram, Citation2013; Song, Lee, Seo, Kim, & Yang, Citation2015; Zhai et al., Citation2016).To investigate the Al-doping effects on structural parameters of ZnO, the three most pronounced XRD peaks are shown in the inset of . It can be seen from the inset that the diffraction peaks of the doped aerogel are slightly shifted towards higher diffraction angles compared to the undoped ZnO sample, indicating a decrease in the lattice parameters. The lattice constants a and c for both aerogels are calculated, respectively, from the positions of (100) and (002) using the formulas:
(1)
(1)
(2)
(2)
where
is the diffraction angle and
is the X-rays wavelength (0.154 nm).
Figure 1. XRD patterns of pure and Al-doped ZnO aerogels synthesized in supercritical isopropanol. The inset shows XRD patterns of the three most important peaks.
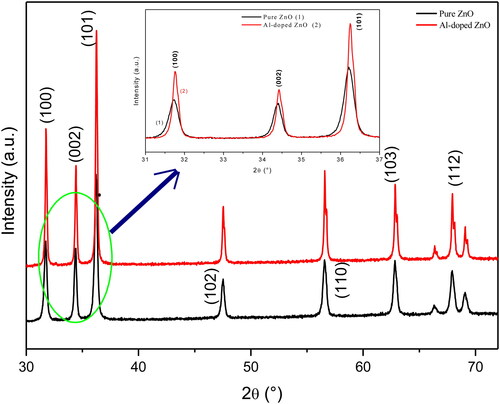
The average crystallites size of pure and Al-doped ZnO powders is calculated using Scherrer formula:
(3)
(3)
where is the full-width at half maximum (in radians) of the most pronounced XRD peaks. D1, D2 and D3 are the average size of the crystallites calculated from the positions of the (100), (002) and (101) peaks, respectively, and Da is the average value. The length of Zn-O bond L is calculated for both aerogels using the formula (Barret & Massalski, Citation1980; Singhal et al., Citation2012):
(4)
(4)
Where, in wurtzite crystallographic structure, the parameter is given by:
(5)
(5)
The degree of crystallinity is calculated using the equation (Ren, Xin, Ge, & Leng, Citation2009):
(6)
(6)
where β002 is the full width at half maximum (in degrees) of the peak (002) .
The induced strain is calculated using the equation (Anandhi et al., Citation2018):
(7)
(7)
The microstrain along the c-axis () is calculated using the following formula (Belkhaoui, Mzabi, Smaoui, & Daniel, Citation2019):
(8)
(8)
where
and
(5.206 Å) are the calculated and standard lattice constant parameters, respectively.
The dislocation density (δ), which is the number of dislocation lines per unit volume present in the as-synthesized aerogels, is calculated for both samples by the following formula (Srinivasulu, Saritha, & Ramakrishna Reddy, Citation2017):
(9)
(9)
where
is the average crystallites size.
All the calculated parameters and the average crystallite sizes of both aerogels are summarized in .
Table 1. The structural parameters and crystallites size of the pure and Al-doped ZnO aerogels.
The values of a and c for pure ZnO are found to be 3.2531 Å and 5.2101 Å, respectively. For the doped sample, these values decrease to 3.2486 Å and 5.2033 Å. The shrinkage of the lattice parameters could be due to smaller ionic radius of Al3+ (0.53 Å) substituted in place of Zn2+ (0.74 Å) site in the ZnO lattice. Moreover, this decrease leads to reduction in the unit cell volume and the length of Zn-O bond (L). The L value is found to be 1.9795 Å in pure ZnO and 1.9766 Å in the doped sample. The enhancement of ZnO crystal quality after Al-doping is confirmed by the increase of Xc value from 1.32 to 3.05, which clearly indicates that the majority of Al3+ occupy Zn2+ sites. The degree of crystallinity Xc, also like the average crystallites size Da, is directly related to the full width at half maximum of the (002) peak. The crystallites size of ZnO increases after the introduction of Al atoms in ZnO lattice. This increase may be attributed to the segregation of dopant atoms on the surface of ZnO nanoparticles and to the improvement of the crystalline quality (Belkhaoui et al., Citation2019; Sayari & El Mir, Citation2015). Furthermore, it is noted that there is a reduction in the lattice strain after Al-doping, which causes a local distortion of the crystal structure due to the difference in the ionic radii of Zn2+ and Al3+ (Nafees, Liaqut, Ali, & Shafique, Citation2013; Sayari & El Mir, Citation2015).
Also, it is remarkable (from the ) that the microstrain along c-axis is found to be compressive for Al-doped ZnO aerogel and tensile for the pure one. This may be due to the fact the incorporated Al3+ ions are initially trapped in positions of non-equilibrium and, for this concentration (3 at. %), they could have shifted to equilibrium positions, which could release the tensile strain. The decrease in lattice strain (
) and dislocations density (
) after Al-doping indicates the presence of fewer defects in the doped aerogel and formation of high crystal quality at this doping concentration (Belkhaoui et al., Citation2019; Srinivasulu et al., Citation2017).
SEM micrographs of pure and Al-doped ZnO are shown in . It can be observed that pure ZnO demonstrates quasi-spherical grains with practically uniform size. These grains are formed by uniformly small ZnO nanoparticles agglomeration. However, the grains of the doped aerogel formed by randomly agglomerated crystallites are with different sizes. Only, a few of these grains appear slightly bigger compared to those of the pure ZnO sample. These changes in grains size after Al-doping are probably due to the concentration of Al atoms on the surface of ZnO crystallites, which is directly related to the number of small crystallites attaching to larger crystallites. The presence of Al atoms in the aerogel plays an important role in the agglomeration process of the crystallites.
Figure 2. SEM images (with two different magnifications) of undoped and Al-doped ZnO aerogels synthesized in supercritical isopropanol.
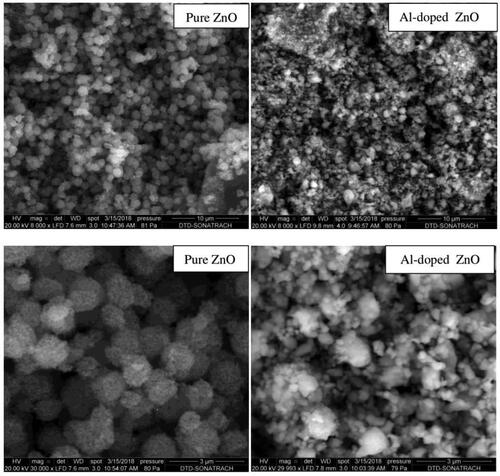
shows FTIR spectra of pure and Al-doped ZnO nanoparticles. Both the samples have a peak at 3450 cm−1 which is assigned to the stretching and bending vibration of surface -OH groups on ZnO particles. The peak at 1405 cm−1 is attributed to bending vibrations (deformation) of hydroxyl groups C-OH groups (Lee et al., Citation2010), while the band at 1620 cm−1 is ascribed to C = O stretching vibrations of the – COOH groups (Spyrou et al., Citation2014). The very low intensity band located at 2350 cm−1 is produced by atmospheric CO2. The peaks observed at 466 and 507 cm−1 for pure and doped ZnO, respectively, are attributed to the stretching vibration of the Zn-O bond. The shift of the IR peak to higher wavenumber with Al-doping could be due to the local strain acting on ZnO nanocrystallites and their size. Sowri Babu, Ramachandra Reddy, Sujatha, Venugopal Reddy, and Mallika (Citation2013) have reported the same behaviour of the position of Zn-O absorption band in ZnO microcrystal. The small intensities of absorption bands related to organic species testify the high chemical purity of the elaborated aerogels and the improvement of the crystal quality upon Al-doping. The decrease in intensity of the infrared absorption band of Zn-O bond vibration of the Al doped sample is probably due to the diminution of Zn-O bond length. Indeed, this result confirms the occupation of Zn 2+ sites by Al3+ ions in ZnO lattice. One can also note a shift of the Zn-O bond position towards high wavenumbers side from 488 cm−1 to 520 cm−1 with Al-doping. This shift maybe attributed to the Al-O bond observed in Al- doped ZnO between 490 cm−1 and 590 cm−1 (Shahid et al., Citation2016).
Figure 3. FTIR spectra of undoped and Al-doped ZnO aerogels synthesized in supercritical isopropanol.
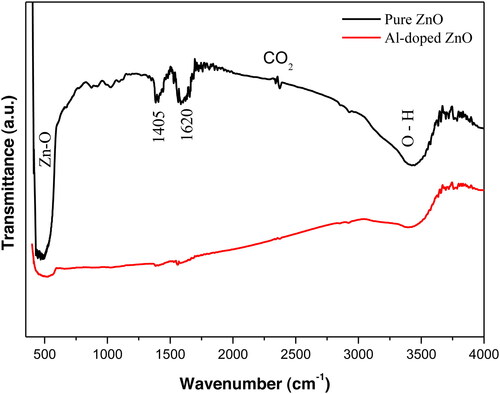
shows optical absorption spectra of pure and Al-doped ZnO aerogels. Pure ZnO exhibits higher absorbance in the UV region compared to the doped sample. The increase in absorbance in the visible region for the Al-doped ZnO may be due to different factors including particle size and defects in crystal structure (Mir & Batoo, Citation2016). It is well known that Al atoms located on ZnO particles surface exhibit surface plasmon resonance and can show an intense absorption in the visible region (Chitra, Uthayarani, Rajasekaran, & Girija, Citation2013). The optical band gap (Eg), determined from the absorbance spectrum which is equal to the difference between the HOMO and LUMO energy levels, corresponds to the first optical transition of lower energy that can be absorbed by the material [Z]. The estimated value f the optical bandgap is given by the formula:
(10 )
(10 )
where λ0 is determined by the intersection of the linear region of the absorbance and the constant visible absorbance as shown in . The estimated values of the band gap are found to be 3.13 eV in the doped aerogel and 3.18 eV for pure ZnO. The decrease in the band gap energy of the doped sample may be attributed to the increase in the crystallites size and the enhancement of the crystal quality as confirmed by XRD measurements. This decrease in optical bandgap indicates the weakening of the quantum confinement effect in ZnO aerogel upon Al-doping. The obtained result is in well agreement with some reported works in literature (Ahmad et al., Citation2013; Echresh & Shoushtari, Citation2013; Krstulovic et al., Citation2018) where it was pointed out that Al-doping creates some energy donor levels in the band gap that leads to reduce the optical band gap of ZnO nanoparticles.
Figure 4. Optical absorption spectra of undoped and Al-doped ZnO aerogels synthesized in supercritical isopropanol.
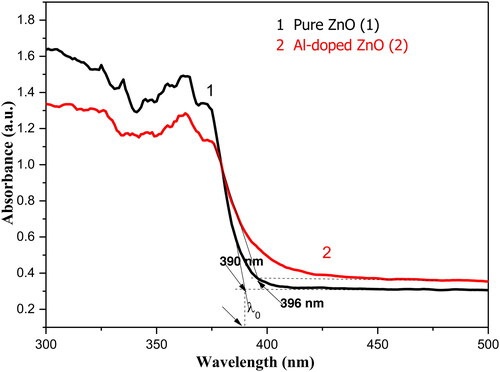
Similar behavior of the optical gap of the Al-doped ZnO has been reported in the literature and the reasons of these red shifts are different. Some authors attributed the decrease of the optical gap in Al-doped ZnO to the quantum confinement effect (Wang, Yang, & Yang, Citation2011). Robles-Águila, Luna-López, Hernández de la Luz, Martínez-Juárez, and Rabana have observed that transition metal ions might additionally introduce d–d transitions into the UV–Vis spectra if the samples are present in a suitable oxidation state, and as a result, the substitution of Zn2+ by Al3+ in Al-doped ZnO exhibits the formation of oxygen vacancies and increases free electrons, hence, generating conductivity in the semiconductor (Robles Alguila et al., 2018).
The band gap narrowing may be also due to the doping induced band edge bending (Ahmad et al., Citation2013) and also to the increase in the average crystallites size (El Yamny & Abdel Rafea, Citation2012). Sharma and Jha have reported that the decrease in optical band gap can be interpreted as stronger sp-d exchange interaction between the band electrons of ZnO (in valence and conduction bands) and the localized d electrons of the Al3+ ions substituting for Zn2+ ions (2017). The strong interaction (s-d and p-d) results into a positive and negative correction to the valence and conduction band edges, respectively. Dabir, Esfahani, Bakhtiargonbadi, and Khodadadi have suggested that the decrease in optical band gap of ZnO films after Al-doping may be due to the decrease of electron-hole recombination rate (2020). This is the possible causes of shrinkage of the band gap in our Al-doped sample.
depicts room temperature PL spectra of pure and doped ZnO samples. The PL spectrum of the doped aerogel is found to be more intense in the investigated wavelength range. Both PL spectra consist of a sharp and intense UV emission at 380 nm (3.26 eV), a low blue emission band located at 466 nm (2.66 eV) and a very weak green emission at 535 nm (3.32 eV). The UV emission at 380 nm is followed by a shoulder at 390 nm, which is a near band edge emission with higher intensity compared to other bands occurring in the visible region. The UV emission is assigned to band-to-band transitions and the shoulder is due to excitons. The blue emission centered at 466 nm is originated from the intrinsic defects and/or Al impurities (Djurišić et al., Citation2006). Zn interstitial (Zni) and oxygen vacancy (VO) are main donor defects while Zn vacancy (VZn) and oxygen interstitial (Oi) are main acceptor defects in intrinsic ZnO. Xia, Hu, Hu, Ping, and Wang (Citation2011) have reported that the 466 nm blue emission is due to the transition of electrons from the level of interstitial Zn (Zni) to zinc vacancy (VZn). They suggested that some of Al3+ ions partially substitute Zn2+ ions in ZnO crystal lattice, and meanwhile, the Zn2+ ions released their sites must have entered into the volume of the lattice to form the interstitial zinc (Zni) defects. Various deep level defect states originated from the zinc interstitials (Zni) and/or oxygen vacancies (VO) in ZnO lattice lead to the appearance of the green emission centered at 535 nm (Zhang, Wei, & Zunge, Citation2001). Thandavan, Gani, Wong, and Nor (Citation2015) have reported a green emission around 540 nm in pure and Al-doped ZnO nanostructures prepared by thermal chemical vapor deposition and have attributed this emission to the radial recombination of photo-generated hole with electron of singly ionized charged particles in the VO. Some others works reported that oxygen vacancies VO slightly contribute to the formation of green emission compared to VZn2- (Patterson, Citation2006; Zhang et al., Citation2001). The VZn2- defects are found to be most energetically favorable and stable in the ZnO nanostructures (Wang et al., Citation2007). As can be seen in , the green emission of pure ZnO is more intense compared to the doped sample. It is believed that this emission is due to oxygen vacancies as reported in the literature (Djouadi et al., Citation2010; Kim & Kang, Citation2011). These later defects may be favored by the introduction of Al atoms in ZnO structures during the formation process.
4. Conclusion
Pure and Al-doped ZnO (3 at. %) aerogels have been synthesized using zinc acetate dihydrtate, aluminum nitrates and methanol as precursor, dopant source and solvent, respectively. The obtained homogeneous solutions have been dried at supercritical conditions of isopropanol. SEM image of pure ZnO nanostructures reveals spherical grains morphology and uniform size, while that of the doped aerogel discloses grains with random forms and different sizes. XRD results have shown a unique phase of ZnO with hexagonal wurtzite structure, an enhancement of crystal quality and a decrease in the structural parameters of ZnO lattice after Al-doping. FTIR results have revealed that the presence of Al atoms leads to bands with a decreasing intensity and a shift of the Zn-O bond band towards higher wave numbers. UV–Visible measurements put into evidence that both absorption and band gap are found to decreasein Al-doped ZnO. Room temperature PL spectra have demonstrated that the PL emission is affected by the Al-doping, especially, a decrease in the green emission at 535 nm is observed.
Disclosure statement
No potential conflict of interest was reported by the authors.
References
- Ahmad, M., Ahmad, E., Zhang, Y., Khalid, N. R., Xu, J., Ullah, M., & Hong, Z. (2013). Preparation of highly efficient Al-doped ZnO photocatalyst by combustion synthesis. Current Applied Physics, 13, 697–704. doi:10.1016/j.cap.2012.11.008
- Alaeddine, A., Rachidi, I., Bahsoun, F., Mohanna, Y., Bazzi, O., & El Haj Hassan, F. (2009). Influence of Al dopant on the optical and electrical properties of zinc oxide thin films by spray pyrolysis. Journal of Applied Sciences, 9, 1588–1592. doi:10.3923/jas.2009.1588.1592
- Anandhi, B., Shankar Ganesh, A., Thangarasu, R., Sakthivel, R., Kannusamy, R., & Tamilselvan, K. (2018). Structural, morphological and optical properties of aluminium doped ZnO thin film by dip-coating method. Oriental Journal of Chemistry, 34, 1619–1624. doi:10.13005/ojc/340356
- Barret, C. S., & Massalski, T. B. (1980). Structures of metals: Crystallographic methods, principles and data. Oxford, UK: Pergamon Press.
- Belkhaoui, C., Mzabi, N., Smaoui, H., & Daniel, P. (2019). Enhancing the structural, optical and electrical properties of ZnO nanopowders through (Al + Mn) doping. Results in Physics, 12, 1686–1696. doi:10.1016/j.rinp.2019.01.085
- Ben Ayadi, Z., El Mir, L., Djessas, K., & Alaya, S. (2007). Electrical and optical properties of aluminum-doped zinc oxide sputtered from an aerogel nanopowder target. Nanotechnology, 18, 445702–445706. doi:10.1088/0957-4484/18/44/445702
- Chang, P. C., Fan, Z., Wang, D., Tseng, W. Y., Chiou, W. A., Hong, J., & Lu, J. G. (2004). ZnO nanowires synthesized by vapor trapping CVD method. Chemistry of Materials, 16, 5133–5137. doi:10.1021/cm049182c
- Chitra, M., Uthayarani, K., Rajasekaran, N., & Girija, E. K. (2013). Preparation and Characterisation of Al doped ZnO Nanopowders. Physics Procedia, 49, 177–182. doi:10.1016/j.phpro.2013.10.024
- Dabir, F., Esfahani, H., Bakhtiargonbadi, F., & Khodadadi, Z. (2020). Study on microstructural and electro-optical properties of sol–gel derived pure and Al/Cu-doped ZnO thin films. Journal of Sol-Gel Science and Technology, 2020, 1–10. doi:10.1007/s10971-020-05269-0
- Djouadi, D., Aksas, A., & Chelouche, A. (2010). Elaboration et caractérisations structurales et optique de nanocristallites toriques de ZnO. Annales de Chimie Science Des Matériaux, 35, 255–260. doi:10.3166/acsm.35.255-260
- Djurišić, A. B., Leung, Y. H., Tam, K. H., Ding, L., Ge, W. K., Chen, H. Y., & Gwo, S. (2006). Green, yellow, and orange defect emission from ZnO nanostructures: Influence of excitation wavelength. Applied Physics Letters, 88, 103103–103107. doi:10.1063/1.2182096
- Duan, L., Zhao, X., Zheng, Z., Wang, Y., Geng, W., & Zhang, F. (2015). Structural, optical and photocatalytic properties of (Mg, Al)-codoped -ZnO powders prepared by sol–gel method. Journal of Physics and Chemistry of Solids, 76, 88–93. doi:10.1016/j.jpcs.2014.07.003
- Echresh, A., & Shoushtari, M. Z. (2013). Synthesis of Al-doping ZnO nanoparticles via mechanochemical method and investigation of their structural and optical properties. Materials Letters, 109, 88–91. doi:10.1016/j.matlet.2013.07.059
- El Yamny, S., & Abdel Rafe, M. (2012). Preparation and characterization of ZnO: In transparent conductor by low cost dip coating technique. Journal of Modern Physics, 03, 1060–1069. doi:10.4236/jmp.2012.39140
- Farhat, O. F., Halim, M. M., Abdullah, M. J., Ali, M. K. M., & Allam, N. K. (2015). Morphological and structural characterization of single-crystal ZnO nanorod arrays on flexible and non-flexible substrates. Beilstein Journal of Nanotechnology, 6, 720–725. doi:10.3762/bjnano.6.73
- Gao, Y. P., Sisk, C. N., & Hope-Weeks, L. J. (2007). A sol-gel route to synthesize monolithic zinc oxide aerogels. Chemistry of Materials, 19, 6007–6011. doi:10.1021/cm0718419
- Ghosh, C. K., Das, S., & Chattopadhyay, K. K. (2007). Enhancement of thermopower of Mn doped ZnO thin film. Physica B: Condensed Matter, 399(1), 38–46. doi:10.1016/j.physb.2007.05.019
- Hasnidawani, J. N., Azlina, H. N., Norita, H., Bonnia, N. N., Ratim, S., & Ali, E. S. (2016). Synthesis of ZnO nanostructures using Sol-Gel method. Procedia Chemistry, 19, 211–216. doi:10.1016/j.proche.2016.03.095
- Khayyat, S. A., Abaker, M., Umar, A., Alkattan, M. O., Alharbi, N. D., & Baskoutas, S. (2012). Synthesis and characterizations of Cd-doped ZnO multipods for environmental remediation application. Journal of Nanoscience and Nanotechnology, 12, 8453–8458. doi:10.1166/jnn.2012.6801
- Kim, K., & Kang, S. (2011). Investigation of photoluminescence mechanisms of ZnO through experimental and first-principles calculation methods. Acta Materialia, 59(1), 126–132. doi:10.1016/j.actamat.2010.09.016
- Krstulovic, N., Salamon, K., Budimlija, O., Kovac, J., Dasovic, J., Umek, P., & Capan, I. (2018). Parameters optimization for synthesis of Al-doped ZnO nanoparticles by laser ablation in water. Applied Surface Science, 440, 916–925. doi:10.1016/j.apsusc.2018.01.295
- Lee, D.W., De Los Santos, L.V., Seo, J.W., Felix, L. L., Bustamante, A. D., Cole, J.M., & Barnes, C. H. W. (2010). The structure of graphite oxide: Investigation of its surface chemical groups. The Journal of Physical Chemistry B, 114, 5723–5728. doi:10.1021/jp1002275
- Meddouri, M., Djouadi, D., Chelouche, A., Touam, T., & Chergui, A. (2014). Effect of co-solvent on structural and morphological properties of ZnO aerogel prepared by a modified sol-gel process. The European Physical Journal: Applied Physics, 66, 10402–10406. doi:10.1051/epjap/2014140061
- Mir, F. A., & Batoo, K. M. (2016). Effect of Ni and Au ion irradiations on structural and optical properties of nanocrystalline Sb-doped SnO2 thin films. Applied Physics A, 122, 417–418. doi:10.1007/s00339-016-9948-3
- Mouzaia, F., Djouadi, D., Chelouche, A., & Hammiche, L. (2018). Al-doping effects on structural and morphological properties of ZnO aerogels synthesized in supercritical ethanol, international symposium on materials and sustainable development (pp. 81–88). Springer International Publishing AG. doi:10.1007/978-3-319-89707-3_10
- Nafees, M., Liaqut, W., Ali, S., & Shafique, M. A. (2013). Synthesis of ZnO/Al:ZnO nanomaterial: Structural and band gap variation in ZnO nanomaterial by Al doping. Applied Nanoscience, 3(1), 49–55. doi:10.1007/s13204-012-0067-y
- Neves, N., Barros, R., Antunes, E., Calado, J., Fortunato, E., Martins, R., & Ferreira, I. (2012). Aluminum doped zinc oxide sputtering targets obtained from nanostructured powders: Processing and application. Journal of the European Ceramic Society, 32, 4381–4391. doi:10.1016/j.jeurceramsoc.2012.08.007
- Patterson, C. (2006). Role of defects in ferromagnetism in Zn1-xCoxO: A hybrid density functional study. Physical Review B, 74, 144432–144413. doi:10.1103/PhysRevB.74.144432
- Phan, D. T., Farag, A. A. M., Yakuphanoglu, F., & Chung, G. S. (2012). Optical and photoluminescence properties of Ga doped ZnO nanostructures by sol-gel method. Journal of Electroceramics, 29(1), 12–22. doi:10.1007/s10832-012-9731-6
- Ren, R., Xin, R., Ge, X., & Leng, Y. (2009). Characterization and structural analysis of zinc-substituted hydroxyapatites. Acta Biomaterialia, 5, 3141–3149. doi:10.1016/j.actbio.2009.04.014
- Ridhuan, N. S., Razak, K. A., Lockman, Z., & Abdul Aziz, A. (2012). Structural and morphology of ZnO nanorods synthesized using ZnO seeded growth hydrothermal method and its properties as UV sensing. PLoS One, 7, e50405. doi:10.1371/journal.pone.0050405
- Robles-Águila, M. J., Luna-López, J. A., Hernández de la Luz, A. D., Martínez-Juárez, J., & Rabana, M. E. (2018). Synthesis and characterization of nanocrystalline ZnO doped with Al3+ and Ni2+ by a sol–gel method coupled with ultrasound irradiation. Crystals, 8, 406–410. doi:10.3390/cryst8110406
- Salam, S., Islam, M., & Akram, A. (2013). Sol–gel synthesis of intrinsic and aluminum-doped zinc oxide thin films as transparent conducting oxides for thin film solar cells. Thin Solid Films, 529, 242–247. doi:10.1016/j.tsf.2012.10.079
- Sayari, A., & El Mir, L. (2015). Structural and optical characterization of Ni and Al co-doped ZnO nanopowders synthesized via the sol-gel process. KONA Powder and Particle Journal, 32, 154–162. doi:10.14356/kona.2015003
- Shahid, M. U., Deen, K. M., Ahmad, A., Akram, M. A., Aslam, M., & Akhtar, W. (2016). Formation of Al-doped ZnO thin films on glass by sol–gel process and characterization. Applied Nanoscience, 6, 235–241. doi:10.1007/s13204-015-0425-7
- Sharma, D., & Jha, R. (2017). Transition metal (Co, Mn) co-doped ZnO nanoparticles: Effect on structural and optical properties. Journal of Alloys and Compounds, 698, 532–538. doi:10.1016/j.jallcom.2016.12.227
- Singhal, S., Kaur, J., Namgyal, T., & Sharma, R. (2012). Cu-doped ZnO nanoparticles: Synthesis, structural and electrical properties. Physica B: Condensed Matter, 407, 1223–1226. doi:10.1016/j.physb.2012.01.103
- Song, M. K., Lee, M. Y., Seo, J. H., Kim, M. H., & Yang, S. Y. (2015). Synthesis of high crystalline Al-doped ZnO nanopowders from Al2O3 and ZnO by radio-frequency thermal plasma. Journal of Nanomaterials, 2015, 1–6. doi:10.1155/2015/151532
- Sowri Babu, K., Ramachandra Reddy, A., Sujatha, C., Venugopal Reddy, K., & Mallika, A. N. (2013). Synthesis and optical characterization of porous ZnO. Journal of Advanced Ceramics, 2, 260–265. doi:10.1007/s40145-013-0069-6
- Sperling, R. A., & Parak, W. J. (2010). Surface modification, functionalization and bioconjugation of colloidal inorganic nanoparticles. Philosophical Transactions. Series A, Mathematical, Physical, and Engineering Sciences, 368, 1333–1383. doi:10.1098/rsta.2009.0273
- Spyrou, K., Potsi, G., Diamanti, E. K., Ke, X., Serestatidou, E., Verginadis, I. T., … Van Tendeloo, G. (2014). Towards novel multifunctional pillared nanostructures: Effective intercalation of adamantylamine in graphene oxide and smectite clays. Advanced Functional Materials, 24, 5841–5850. doi:10.1002/adfm.201400975
- Srinivasulu, T., Saritha, K., & Ramakrishna Reddy, K. T. (2017). Synthesis and characterization of Fe-doped ZnO thin films deposited by chemical spray pyrolysis. Modern Electronic Materials, 3, 76–85. doi:10.1016/j.moem.2017.07.001
- Thandavan, T. M. K., Gani, S. M. A., Wong, C. S., & Nor, M. R. (2015). Enhanced photoluminescence and raman properties of Al-doped ZnO nanostructures prepared using thermal chemical vapor deposition of methanol assisted with heated brass. PLoS One, 10, e0121756. doi:10.1371/journal.pone.0121756
- Wang, M., Lee, K. E., Hahn, S. H., Kim, E. J., Kim, S., Chung, J. S., … Park, C. (2007). Optical and photoluminescent properties of sol-gel Al-doped ZnO thin films. Materials Letters, 61, 1118–1121. doi:10.1016/j.matlet.2006.06.065
- Wang, N., Yang, Y., & Yang, G. (2011). Great blue-shift of luminescence of ZnO nanoparticle array constructed from ZnO quantum dots. Nanoscale Research Letters, 6(1), 338–331. doi:10.1186/1556-276X-6-338
- Xia, C. H., Hu, C. G., Hu, C. H., Ping, Z., & Wang, F. (2011). Room-temperature ferromagnetic properties of Cu-doped ZnO rod arrays. Bulletin of Materials Science, 34, 1083–1087. doi:10.1007/s12034-011-0154-9
- Zhai, C. H., Zhang, R. J., Chen, X., Zheng, Y. X., Wang, S. Y., Liu, J., … Chen, L. Y. (2016). Effects of Al doping on the properties of ZnO thin films deposited by atomic layer deposition. Nanoscale Research Letters, 11(1), 407–408. doi:10.1186/s11671-016-1625-0
- Zhang, S. B., Wei, S. H., & Zunge, A. (2001). Intrinsic n-type versus p-type doping asymmetry and the defect physics of ZnO. Physical Review B, 63, 075205–075207. doi:10.1103/PhysRevB.63.075205