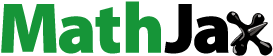
Abstract
The present study is an attempt to investigate the applicability of a natural coagulant Moringa Oleifera (MO) as a potential impending alternative for the treatment of textile wastewater. Face Central Composite Design in Response Surface Methodology was used to optimize the performance of the coagulation process in terms of turbidity and colour removal efficiency. Three independent variables which are pH (2-4), coagulant dosage (4-8 ml) and settling time (10-30 min) were investigated. Quadratic models were developed for each response and their statistical fitness was evaluated using ANOVA analysis. All models were proved to be statistically adequate with R2 higher than 96%. Of the investigated variables, pH was found to have significant effect on both responses (P-value <0.001), whereas, coagulant dosage affected the colour removal efficiency significantly. Multi-response optimization was applied to optimize the process variables for both responses simultaneously using the RSM desirability function approach. Maximum turbidity reduction by more than 81% and colour removal efficiencies by more than 77% were reached at the optimum conditions of pH 2, coagulant dosage 4 mL and settling time 21 min. These results prove that MO could be used as an alternative to the conventional coagulants for real textile wastewater treatment.
1. Introduction
Coagulation is one of the commonly used technologies for the treatment of industrial wastewater (Mohd-Salleh, Mohd-Zin, & Othman, Citation2019). Despite the development of different advanced technologies for wastewater treatment (Gupta & Saleh, Citation2013) such as physical methods (Hube et al., Citation2020; Suhas et al., Citation2016), adsorption (Gupta, Rastogi, Dwivedi, & Mohan, Citation1997; Burakov et al., Citation2018; Gupta, Ali, Saleh, Siddiqui, & Agarwal, Citation2013; Gupta, Nayak, & Agarwal, Citation2015; Ahmaruzzaman & Gupta, Citation2011; Ali, Citation2018), chemical and biological oxidation technologies (Hodaifa, Gallardo, García, Kowalska, & Seyedsalehi, Citation2019; Gogate, Thanekar, & Oke, Citation2020), electrochemical method (Zhang et al., Citation2020; Ali, Citation2012), nanostructured photocatalytic technologies (Kumar & Chowdhury, Citation2020; Mohammed Redha, Abdulla Yusuf, Amin, & Bououdina, Citation2020; Gupta, Jain, Nayak, Agarwal, & Shrivastava, Citation2011; Saleh & Gupta, Citation2012; Saravanan et al., Citation2016; Rajendran et al., Citation2016; Saravanan, Gupta, Prakash, Narayanan, & Stephen, Citation2013; Saravanan, Gupta, et al., Citation2013), and integrated and hybrid processes (Ang & Mohammad, Citation2019; Demir, Chehade, Dincer, Yuzer, & Selcuk, Citation2019), coagulation still finds application as a popular method in various fields (Ang & Mohammad, Citation2020). The popularity of coagulation for the treatment of different industrial effluents (Sher, Malik, & Liu, Citation2013; Bruno, Campo, Giustra, De Marchis, & Di Bella, Citation2020; Saha, Boro, & Das, Citation2019) is attributed to its low capital cost and the simplicity of the separation approach (Jiang, Citation2015). It is a process by which impurities like suspended particles and colloids in the wastewater are allowed to destabilize through neutralizing their charges by the addition of coagulants (Jiang, Citation2015). The destabilized particles are then agglomerated into larger aggregates that can rapidly settle down and thus easily separated from the water (Ang & Mohammad, Citation2020).
One of the major applications of coagulation can be found in textile industries (Torres et al., Citation2019) where large amounts of water are spent and substantial quantities of effluents are discharged. The quality and complexity of the effluent may vary depending on the processes involved at the various production stages, like bleaching, dyeing, printing, etc (Torres et al., Citation2019). Each of these processes contributes to the release of different types of persistent and non-biodegradable pollutants into the wastewater, making it hard to be treated (Jorfi et al., Citation2016; Dasgupta, Sikder, Chakraborty, Curcio, & Drioli, Citation2015). Among all the complex constituents, dyes are considered as one of the most prominent sources of pollutants in the textile wastewater (Dasgupta et al., Citation2015; Dil et al., Citation2016; Saravanan, Gupta, et al., Citation2013) as 50% of their dosage is washed away during the process into the waste stream (Harrelkas, Azizi, Yaacoubi, Benhammou, & Pons, Citation2009). Acid and reactive dyes in particular are designed to resist conventional wastewater treatment technologies (Zahrim, Tizaoui, & Hilal, Citation2011; Anjaneyulu, Sreedhara Chary, & Samuel Suman Raj, Citation2005). Many researches have been conducted on the removal of such dyes (Saravanan, Gupta, et al., Citation2013; Nekouei, Nekouei, Tyagi, & Gupta, Citation2015; Mittal, Mittal, Malviya, & Gupta, Citation2010; Saleh & Gupta, Citation2014; Saleh & Gupta, Citation2011; Saravanan, Khan, et al., Citation2015; Saravanan, Khan, et al., Citation2015; Ghaedi et al., Citation2015; Saravanan, Gupta, et al., Citation2013; Ali et al., Citation2019), however, coagulation was found to be a very effective approach that can efficiently accelerate the removal of such pollutants (Jorfi et al., Citation2016; Riera-Torres, Gutiérrez-Bouzán, & Crespi, Citation2010). The effectiveness of the dye coagulation performance is highly influenced by the selection of the coagulant type and dosage, both factors can either promote or impede the treatment efficiency (Mohd-Salleh et al., Citation2019; Dotto, Fagundes-Klen, Veit, Palácio, & Bergamasco, Citation2019). Generally, coagulants can be classified as organic and inorganic, yet, for industrial scale applications, inorganic coagulants such as iron and aluminum salts, like ferric chloride (FeCl3) and alum (aluminium sulphate) (Al2(SO4)3), are predominant (Dotto et al., Citation2019; Tiaiba, Citation2018). Nevertheless, the use of inorganic coagulants has eminent drawbacks, one of which is the production of large quantities of toxic sludge (Dotto et al., Citation2019). The toxic sludge was reported to be the result of unreacted chemical residuals as well as reaction byproducts of polymers in treated water (Mohd-Salleh et al., Citation2019). Alum based sludge for example was described to be difficult to dewater (Gupta et al., Citation2011). Another potential problem is associated with health issues such as Alzheimer’s, and dementia (Kristianto, Kurniawan, & Soetedjo, Citation2018).
Natural coagulants, on the other hand, are proved to be overcoming such drawbacks. They are eco-friendly, toxic free and renewable resources, especially those extracted from plants (Mohd-Salleh et al., Citation2019). Moreover, natural coagulants have biodegradable characteristics (Ang & Mohammad, Citation2019), which make them safe for ecological and living organisms (Mohd-Salleh et al., Citation2019). They are readily available, with relatively lower cost than inorganic coagulants and they produce smaller sludge volumes (Ang & Mohammad, Citation2019). These advantages make them suitable alternatives to inorganic types.
Natural coagulants could be classified as plant-based and non-plant based coagulants in general, and they can be used as full replacements or alongside the inorganic coagulants (Villaseñor-Basulto, Astudillo-Sánchez, del Real-Olvera, & Bandala, Citation2018). Manholer et al. (Manholer et al., Citation2019) investigated the use of mucilage extracted from the fruit of Dillenia Indica as a support to the FeCl3.6H2O chemical coagulant for enhancing the coagulation process in the treatment of textile effluent. More efficient chemical oxygen demand (COD) removal was achieved at a low chemical coagulant dosage and a higher natural coagulant dosage. They successfully reached 67.66%, 96.86% and 91.12% COD, turbidity and colour removal, respectively. Many researchers investigated the performance of other natural coagulants such as potato starch (Ahmad, Citation2018), papaya seeds (Kristianto et al., Citation2018), sago, chitin (Saritha, Karnena, & Dwarapureddi, Citation2019) and okra (Freitas et al., Citation2015) as possible alternatives to the chemical coagulants. Strychnospotatorum (Nirmali seeds) and Eirchorrniacrassipes (water hyacinth) were seen to give better reduction for total suspended solids (TSS), COD and biological oxygen demand (BOD) over alum (Prabhakaran, Manikandan, & Boopathi, Citation2020). Among the extensive list of natural coagulants, Moringa Oleifera (MO) extract (Demir et al., Citation2019; Jiang, Citation2015) has been intensively investigated previously (Saleem & Bachmann, Citation2019). MO extract is reported to contain proteins acting as active coagulating agents that can effectively destabilize the suspended particles (Villaseñor-Basulto et al., Citation2018). In a recent study using MO, 92% colour removal efficiency was achieved for synthetic Congo red dye solution at coagulant dosage of 200 mg/L and 60 min flocculation time (Suresh, Ganesh, & Velmurugan, Citation2019).
Most of the coagulation studies using natural coagulants were conducted on synthetic textile waste (Kristianto et al., Citation2018; Suresh et al., Citation2019; Obiora-Okafo & Onukwuli, Citation2017; Onukwuli, Obiora-Okafo, & Omotioma, Citation2019; Dalvand et al., Citation2016) or surface water (Adesina, Abdulkareem, Yusuff, Lala, & Okewale, Citation2019), yet little attention was given to real waste samples. Until recently, the new trends in the field were directed towards investigating the performance of these natural coagulants on real waste samples for the purpose of finding better alternatives for industrial applications (Dotto et al., Citation2019; Rambe, Citation2018; Dos Santos, Citation2018). Although, some of these studies gave reasonably good results when applied to synthetic waste, but the removal efficiency was comparatively low when applied to real samples (Saritha et al., Citation2019). Yet many have concluded that MO has a great potential as an alternative coagulant for the treatment of textile waste water regardless of its complex nature (Dotto et al., Citation2019). Thus, there seem a lot of opportunities for further investigations and more research to be carried out in this field.
Different optimization tools were employed to investigate the effect of the operating parameters on the efficiency of the coagulation process. Rambe et al. (Zahrim et al., Citation2011) used the conventional, single variable experiments to study the factors affecting the coagulation process using MO for the treatment of jeans leaching waste. In another similar study using single variable experimental approach, MO was evaluated for treatment of segregated and mixed wastewater from the cotton industry. However, these studies neither investigated the interference effect between the process variables nor their significance (Nekouei et al., Citation2015). In contrast, the Design of Experiment (DoE) approach provides a systematic investigation and optimization of the process variables with a few experiments. Additionally, it offers the possibility of exploring multiple variables effects as well as their complex interactions (Lundstedt et al., Citation1998). Ahmad et al. (Ahmad, Citation2018) utilized the Taguchi design of experiments and observed that temperature, pH and coagulant dosage were the most significant parameters affecting the coagulation process. Similarly, Obiora-Okafo and Onukwuli employed Response Surface Methodology (RSM) to optimize the coagulation process of different natural coagulants, including MO, for colour removal of Acid Red 101 in synthesized solution (Obiora-Okafo & Onukwuli, Citation2017). Optimum operating conditions were determined for each coagulant and the colour removal efficiency was observed to increase in acidic solution. Dotto et al. (Citation2019) recently applied factorial design to determine the required coagulant concentration and sedimentation time for textile wastewater treatment obtained from industrial laundry containing reactive dyes.
In the present work, a case study is presented on the applicability of MO as a natural coagulant for the treatment of wastewater effluent from a local textile industry in the Kingdom of Bahrain. A simple, yet effective extraction method for extracting the active agents from MO is described. A systematic investigation approach using the DoE, based on RSM is employed to establish the relationship between the output responses and the input variables. Consulting on the concerned industry, two crucial responses were selected: turbidity and colour removal efficiency. Among the candidate operating variables, coagulant dosage, pH and settling time were selected as the variables, which are most likely to have significant effect on the responses. Multi-response optimization are employed, where a single optimum condition is determined for all responses simultaneously. Unlike other recent studies in the field (Torres et al., Citation2019), in this work all optimization experiments were conducted using real waste samples without purification or pre-treatment and no synthetic samples were used anywhere in this investigation.
2. Materials and methods
Textile wastewater samples were obtained from a local textile plant in the Kingdom of Bahrain. Fresh samples were collected and used within 24 h, without any further purification, treatments or dilution. shows the characteristics of wastewater samples collected from the textile factory. All measurements were taken on the day of collection.
Table 1. Characteristics of the textile wastewater.
The reagents used were analytical grade without any further purification. They are: sodium chloride (NaCl) purchased from Research-Lab Fine Chem Industries, hydrochloric acid (HCl) (37 wt.%) purchased from Sharlau and sodium hydroxide (NaOH) purchased from Timstar Laboratory Suppliers ltd.
2.1. Preparation of MO seed extracts
Moringa Oleifera seed powder was purchased from Neoteric DCBA (India). The active proteins from MO coagulant were extracted by mixing 5 g of dry MO seed powder in 100 mL of 1 M NaCl solution. The mixture was stirred for 1 h using a magnetic stirrer (IKA®-Werke, Germany). The sample was then filtered through 15 cm filter paper (Dr. Watts, India) and the filtrate was used as the coagulant stock solution.
2.2. Characterization of MO extract
A Fourier transform-infrared (FT-IR) absorption spectrometer (Alpha, Bruker Optics GmbH, Germany) was used to verify the presence of the MO active component in the extracted solution. The infrared spectra were recorded on ATR diamond crystal infrared spectrometer in the range of 4000 to 600 cm−1 at a resolution of 4 cm−1. The spectrum was compared with that of the dry MO seed powder.
2.3. Coagulation experiments
The coagulation experiments were performed using a conventional jar test apparatus (Phipps & Bird, U.S.A) equipped with six beakers of 500 mL and six paddle stirrers. 300 ml of wastewater was added to each beaker and its pH was adjusted to the required experimental values by adding HCl or NaOH solutions dropwise. The required volume of coagulant was then added and the mixture was rapidly mixed for 4 min at 100 rpm, followed by slow mixing for 20 min at 30 rpm. All the suspensions were allowed to settle down for 10 to 30 min depending on the experiment. Supernatants of each beaker were withdrawn after settling for turbidity analysis using turbidimeter (Hach 2100 P) and absorbance analysis using UV-Vis spectrophotometer path length 1 cm, apparatus (Shimadzu UV-1800) at maximum absorbance wavelengths of 682 nm and 510 nm. Colour removal was determined by comparing the absorbance of the supernatant from each coagulation experiment to that of the wastewater at maximum absorbance wavelengths. The colour removal efficiency (CR) was then calculated using the following equation:
(1)
(1)
where A0 and A are the initial and final absorbance values at the maximum wavelengths, before and after coagulation-flocculation treatment, respectively.
2.4. Experimental design and data analysis
In this research, RSM with Central Composite Design (CCD) was applied to optimize the process variables. The Faced Centered Central Composite Design (FCCD) of the CCD was implemented by setting α to 1. In FCCD option, the star points are at the centre of each face of the factorial space and thus, 3 levels of each factor are required (Obiora-Okafo & Onukwuli, Citation2017). Three independent variables, namely, pH, coagulant dosage and settling time were studied at three levels. Two main responses were selected based upon consultation with the concerned industry: turbidity and colour removal efficiency. The values of each independent variable at the three levels: low (−1), central (0), and high (+1) are shown in .
Table 2. Levels of the independent process variables used in the experimental design.
For three independent variables, the FCCD full factorial design gave a total of 20 experiments according to Equationequation 2(2)
(2) . These experiments include eight factorial points (2k), six axial points (2k) and six replicated centre points (cp).
(2)
(2)
where N is the total number of experimental runs, k is the number of independent variables, and cp is the number of the central points (Barker & Milivojevich, Citation2016).
To predict the effect of the experimental variables and their interaction on the responses, an empirical correlation based on a quadratic (second-order polynomial) equation was developed according to the following expression (Lundstedt et al., Citation1998):
(3)
(3)
where Y is the predicted response,
is the constant coefficient,
is the linear effect coefficients,
is the quadratic coefficients,
is the interaction effect coefficient,
and
are the coded values of the variables and ε is the random error.
Analysis of variance (ANOVA) was applied to determine the statistical significance of the predicted full quadratic models and the significance of the independent variables affecting the studied responses at 95% confidence level. For a model to be significant, and for the variable to have a significant effect, the P-value should be less than 0.05. The quality of the model fit was also determined by the coefficient of determination (R2). R2 values greater than 0.8 are acceptable for chemical processes (Lundstedt et al., Citation1998). A statistical software package Minitab 18 (Minitab Inc., State College, PA, USA), has been used to design the experiment and to model and analyze the results. Surface and contour plots were used to illustrate the interactive effects of the independent variables on the responses.
3. Results and discussion
3.1. Ftir of the MO extract
It was reported that salt solution extraction of MO gave better coagulation properties comparing to water extraction. This was correlated to the higher amounts of soluble protein in the extract when salt extraction is used, probably because salts increase the ionic strength (Villaseñor-Basulto et al., Citation2018). Moreover, previous studies reported that MO extraction using NaCl salt solutions was more effective than other salt solutions (Prasad, Citation2009). Based on this, salt solution extraction using 1 M NaCl solution was preferred in this work. FTIR spectrum of the extract was recorded to identify the different functional groups and verify the presence of the active protein. This spectrum was then compared to that of MO seed powder and the results are illustrated in .
Figure 1. FTIR spectra for (a) NaCl solution (b) Moringa Oleifera powder (c) MO extract in NaCl solution.
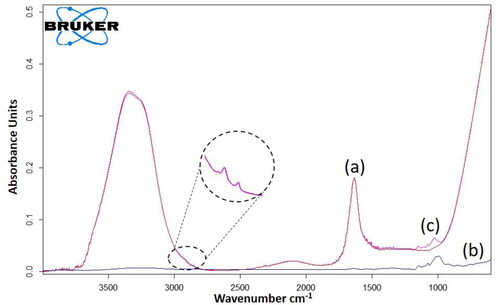
shows the FTIR spectrum for 1 M NaCl solution. The broad intense peak centred at 3335.67 cm−1 is attributed to the O-H stretching, while that at approximately 1635 cm−1 is caused by the O–H–O scissors bending (Mojet, Ebbesen, & Lefferts, Citation2010). These two peaks are correlated to the water molecules. illustrates the FTIR spectrum for MO powder. The broad band between 4000 and 3000 cm−1 may denote the presence of both H-bonded amines and OH groups presented in the proteins, fatty acids, carbohydrates and lignin units in organic matter (Araújo, Citation2013; Reddy, Seshaiah, Reddy, & Lee, Citation2012).The two peaks shown at approximately 2921 cm−1 and 2850 cm−1 are related to asymmetric and symmetric stretching of the C-H bond of the CH2 group respectively (Araújo, Citation2013). The overlapping peaks in the band between 1800 to 1300 cm−1 can be correlated to C = O stretching (Araújo, Citation2013). The peaks at 1733 cm−1 and 1715 cm−1 may represent the carbonyl component due to the lipids, and the shoulder peak at 1650 cm−1 could be attributed to the carbonyl amides present in the protein (Araújo, Citation2013; Nonfodji, Fatombi, Ahoyo, Osseni, & Aminou, Citation2020; Cui et al., Citation2019). The peak observed at 1557 cm−1 could be related to stretching connecting CN and also the deformation of the N-H bond present in the proteins of seeds (Araújo, Citation2013).
The two intense peaks at 2921 cm−1 and 2850 cm−1 that were shown in the MO powder FTIR spectrum, appear again in the MO extracted solution (), indicating successful extract of the active components. These two peaks could be assigned to the predominantly lipid component of the seed, present in a high fraction as that of protein (Araújo, Citation2013). However, due to the very intense and broad bands of water spectrum over the full scan range, the other peaks was difficult to identify clearly (Mojet et al., Citation2010).
3.2. Coagulation results
The effect of independent variables on the turbidity and colour removal efficiency was studied by performing several experiments with different combinations of these variables. RSM equipped with FCCD was used to optimize the process variables. The experimental design matrix along with the obtained responses for each experiment are shown in . The absorption spectra of the untreated wastewater and of the supernatant from each coagulation experiment are shown in . Two absorption peaks are clearly at 682 nm and 510 nm in this figure, confirming the observed dark blue colour of indigo dye in the wastewater (Wambuguh & Chianelli, Citation2008).
Table 3. Set of the coded experimental conditions of independent variables and the corresponding responses.
The experimental data were then used to compute empirical models correlating each response to the three studied independent variables based on the second order polynomial Equationequation 3(3)
(3) . These quadratic models are given as Equationequations 4-6 in uncoded units.
Regression Equation for CR1 in Uncoded Units:
(4)
(4)
Regression Equation for CR2 in Uncoded Units:
(5)
(5)
Regression Equation for Turbidity in Uncoded Units:
(6)
(6)
3.3. Statistical analysis of the models
ANOVA was used to examine the validity of the model. The ANOVA analysis data for the three models are shown in and . As given in from the high F-values and low P-values (<0.05) for the three models, all three models proved to be significant and well fitted at 95% confidence level. In addition, the models did not exhibit lack-of-fit as confirmed from the P-values (> 0.05) indicating that the relationships between the responses and the independent variables assumed in the models are reasonable. Furthermore, the overall performance of the models was measured by the coefficient of determination R2 as shown in . For all three models, the R2 values were greater than 96%, indicating the suitability of the models. Moreover, the insignificant differences between the values of the predicted R2 and adjusted R2 for the three models confirm the accuracy of the models.
Table 4. ANOVA statistical parameters obtained for the models.
Table 5. ANOVA analysis of the models’ terms for the three responses.
illustrates the normal probability plot of residual as well as the model prediction plot for each of the studied responses. For all the three responses, the data in the normal probability plots formed a nearly linear pattern. The data did not show strong deviation from a straight line, demonstrating normal distribution, thus proving a good fit of the models. The model prediction plots for the three responses revealed excellent fit into linear relationship as demonstrated by the R2 values, thus, confirming the validation of the predicted response values with the actual response values. Therefore, the models can be used with high confidence to theoretically predict the represented responses.
The ANOVA analysis shown in along with the Pareto charts illustrated in are applied to identify the variables and interactions that exhibited significant effects on the investigated responses. It is clearly shown in , that the bars representing the pH effect and the square of pH cross the reference line that is at 2.23 for all the three responses. This indicates that both pH and pH2 terms are statistically significant at 95% confidence level. The significance of these terms is also confirmed by their corresponding P-values, which were less than 0.05, as illustrated in . It is also observed that the CD has a significant effect on the colour reduction efficiencies but has no significant effect on the turbidity. Yet, the ST seems to have no significant effect on any of the three responses as confirmed by the corresponding high P-values (>0.05) for the three responses. Furthermore, the Pareto charts revealed that none of the interaction terms affected the three responses significantly. For the square terms, with the exception of the previously discussed pH2, neither ST2 nor CD2 showed any significance on the turbidity and CR1 responses. However, the P-values of the latter square terms were close to the significance cut off at 95% confidence limit and the reference line when analyzed for the CR2 response. The P-values of the insignificant terms were higher than 0.05 ().
DF is Degree of Freedom, SS is Sum of Squares, MS is Mean Square, (adj) is Adjusted, (pre) is Predicted
3.4. Analysis of the response surface plots
The three-dimensional response surface plots of the quadratic model for the responses were developed as a function of two independent variables while the third variable was held constant ( and ) for graphical interpretation of the interactions.
Figure 5. 3 D Surface plots of the Turbidity response. Hold values: ST 20 min for pH vs. CD plots, CD 6 mL for pH vs. ST plots and pH 4 for CD vs. ST plots.
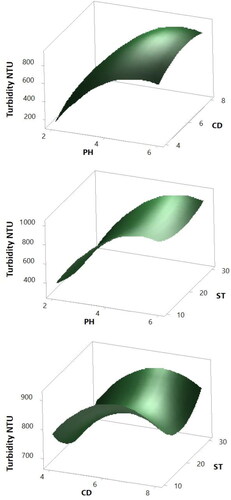
Figure 6. 3 D Surface plots of the Colour removal responses (a) CR1 (b) CR2. Hold values: ST 20 min for pH vs. CD plots, CD 6 mL for pH vs. ST plots and pH 4 for CD vs. ST plots.
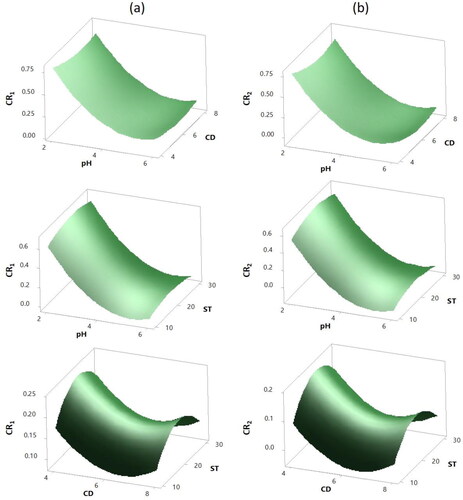
Referring to the turbidity response plots shown in , the pH-CD plot revealed the best response for turbidity at pH and CD values of 2 and 4 mL respectively. The pH increase caused a significantly sharp increase in the turbidity value for all ranges on CD. An increase in the turbidity was also observed with the increase of the CD, however, the effect of pH was more dominant as being the controlling factor on the turbidity response. Similarly, referring to the pH-ST plot for turbidity in , again a strong interaction between the pH and turbidity is observed in conformity with the previous results. Yet moderate interaction between the ST and turbidity is observed. As indicated by the pH-ST plot, any change in the ST value along its axis causes a slight change in the turbidity value. This change is clearly observed again in the CD-ST plot for turbidity. Here, the plot shows that the turbidity response increased at high and low settling times, and minimum values of the response are obtained at ST around 20 min. The same plot also revealed that minimum turbidity is achieved at low CD and moderate ST confirming the previous observations. These results prove the capability of MO for turbidity removal due to the cationic protein contained in the extract (Landázuri et al., Citation2018).
Moreover, the findings in the current work are in good agreement with previously reported studies. For example, Adesina et al. (Adesina et al., Citation2019) reported similar observations of the effect of ST and CD on the turbidity of surface water when MO was used as coagulant: the turbidity of the water increased with ST above or below 120 min. They also reported that low concentrations of MO leads to low turbidity of the water. Many other researches also observed the increase in the turbidity of the treated water with the increase of the MO dosage (Vunain, Masoamphambe, Mpeketula, Monjerezi, & Etale, Citation2019; Kang & Treviño, Citation2017; Sánchez-Martín, Ghebremichael, & Beltrán-Heredia, Citation2010). In an attempt to reduce the turbidity of the treated water, some researchers proposed a second step purification process of the MO extract using ion exchange to remove the inactive proteins (Sánchez-Martín et al., Citation2010). Their results showed enhancement in the turbidity removal based on the purified MO. In the current work, the MO extract was not subjected to any purifications, thus, the observed increase in the turbidity with the increase in coagulant dosage is usual.
With respect to pH, similar observations were obtained by Dotto et al. (Dotto et al., Citation2019). These researchers reported that at elevated pH values, the turbidity removal efficiency declined when NaCl-extracted MO was used as a coagulant. This behaviour was attributed to the reduction in flake formation and its subsequent sedimentation because of the low rate of the inter-particle contact between the MO and the particles in the medium.
Referring to the CR1 and CR2 plots in and b respectively, similar interaction trends for both responses are observed. For both responses, the pH-CD and pH-ST plots showed high dependency of the responses to the variation in the solution pH. A steep reduction in the colour removal efficiencies was observed with the pH increase. Thus, higher colour removal efficiencies were achieved at high acidic range and maximum removal efficiencies were obtained for solutions with initial pH of 2. At acidic conditions, the dye particles are negatively charged, promoting better performance with the cationic nature coagulant. Dotto et al. (Dotto et al., Citation2019) obtained similar results (pH = 2) when studying the colour removal using NaCl-extracted MO, verifying the cationic protein nature of the MO, which can improve the coagulant activity at low pH values. Furthermore, at high pH values a competition between OH- and the negatively charged contaminants may occur for polymer hydrolysis products (Obiora-Okafo & Onukwuli, Citation2017). Similar results were reported by Moghaddam et al. (Sadri Moghaddam, Alavi Moghaddam, & Arami, Citation2010). They mentioned that hydrolysis products could neutralize the negative charges on the dye molecules, however, with the lowering of the pH, reduction in the charge density may cause self-aggregation of the anionic dye molecules. The latter phenomenon can also explain the low CD requirement for the destabilization process at low pH values (Sadri Moghaddam et al., Citation2010). Low-pH conditions may arise due to proton-for-Na substitution in the mineral-fluid interface with saline waters, immobile waters or systems with low water: rock ratios in a variety of sedimentary environments (Cuadros, Afsin, Michalski, & Ardakani, Citation2012)
The effect of CD on the colour reduction efficiencies was significant but with lower degree compared to the effect of pH. Better reduction in the colour removal efficiencies was observed at low CD. Increasing the CD causes a decrease in colour removal; however, any further increase in the CD beyond a certain concentration leads to a slight increase in the colour removal efficiencies. The increase in colour removal efficiencies at higher coagulant dosage is most probably due to the increase in the active component in the dosage (Prasad, Citation2009). The CD-ST plots for colour removal efficiencies in clearly illustrate this behaviour. Peaks for maximum colour removal are clearly observed at low coagulant dosage. This confirms that the charge-neutralization and adsorption were the predominant mechanisms for the colour removal, since these mechanisms are usually dominant at low coagulant dosage (Obiora-Okafo & Onukwuli, Citation2017). Baptista et al. (Saravanan, Gupta, et al., Citation2013) verified that the highest removal rates in colour and turbidity were obtained when the lowest concentrations of MO coagulant were used. The CD-ST plots revealed that at CD higher than 4 mL the removal efficiencies tend to decrease. This behaviour could be attributed to overdosing. With overdosing, the supernatant quality deteriorates due to the “re-stabilization” of the dye particles, which reverses the particle charge (Obiora-Okafo & Onukwuli, Citation2017). Further increase in CD, however, caused a slight increase in the removal efficiencies as shown in the CD-ST plots. This increase could be related to the involvement of sweep flocculation and adsorption mechanisms (Sadri Moghaddam et al., Citation2010), which are favoured at high dosages (Obiora-Okafo & Onukwuli, Citation2017). The ST on the other hand, seemed to have lower effect on the colour efficiencies compared to pH and CD with P-values >0.05. Peaks for maximum colour removal efficiencies of more than 70% were observed at ST around 20 min and higher ST was inefficient. This suggests that equilibrium could be assumed to occur after 20 min at which the active sites are saturated hindering any further adsorption. Thus, destabilization of the aggregated flocs could probably occur after this ST (Obiora-Okafo & Onukwuli, Citation2017).
In summary, the 3 D surface plots revealed that the minimum turbidity and maximum colour removal efficiencies are found inside the design boundaries. Moreover, best responses seemed to be achieved at low pH and CD and moderate ST. Furthermore, low CD is advantageous not only on an economical basis, but also for lowering the organic matter load, thereby preventing the microbial growth (Šćiban, Klašnja, Antov, & Škrbić, Citation2009).
3.5. Process optimization
Different water quality has different operational requirements. Thus, to ensure techno-economic viability of the coagulation process it is vital to optimize the process variables according to the water quality characteristics of the effluent and the treated water quality requirements (Ali et al., Citation2019). Of the investigated variables pH proved to have a significant effect on the coagulation process. It was reported that at high alkalinity water, more dosage of the coagulant is required (Zheng et al., Citation2014). Yet acid conditions promote hydrolysis of the coagulant to form a high positive charge, which will enhance the coagulation process (Riera-Torres et al., Citation2010). Similarly, large doses of coagulant might not be economical. The amount of sludge produced might increase; consequently, a sludge dewatering system might not be sufficient (Riera-Torres et al., Citation2010). The settling time was found to affect the stabilization and destabilization of the charged particles depending on whether equilibrium was reached or not (Menkiti, Okoani, & Ejimofor, Citation2018). Consequently, this will affect the efficiency of the coagulation process. Therefore, for the enhancement of the coagulation process, the optimum operating conditions of the coagulation reaction in terms of pH, dosage and settling time must be determined depending on the type of coagulant used, and the quality of the wastewater.
In this work, multi-response optimization was performed using the RSM desirability function approach. The independent process variables were simultaneously optimized in order to find one optimum condition for the three responses. The desirability function approach was used to achieve minimum turbidity and maximum colour removal efficiencies. The optimum conditions for the responses are shown in as pH of 2, CD of 4 mL and ST of approximately 21 min. These optimum conditions were in great alignment with the results of 3 D response surface plots discussed in the previous section. More than 77% colour removal efficiencies and 148 NTU turbidity value corresponding to approximately 81% reduction in turbidity can be achieved at the optimum conditions. In comparison to the values achieved by the factory, which are 80% colour removal efficiency and 90% turbidity reduction in 20 min settling time, the results obtained under the optimum conditions in the study proved to be very feasible for industrial applications, both cost and time wise.
When compared with other natural coagulants, the results achieved in this study outweighed similar reported studies. Most of the natural coagulants reported in literature could not be used as standalone for the treatment of real textile waste samples, but were introduced as additives to enhance the performance of the inorganic coagulants. Others natural coagulants showed good performance only in synthetic or pre-treated media. However, the present work proved to achieve excellent performance in such complex media, without the need for pre-treatment or the addition of the inorganic coagulant. shows detailed comparison with some reported natural coagulants used for textile wastewater treatment.
Table 6. Performance compassion of MO coagulant used in the present study with other natural coagulants.
4. Conclusion
In the present study, the feasibility of using MO natural coagulant as an alternative for the coagulation process of a real waste effluent from a local textile industry was investigated. The experiments were performed in a jar test apparatus. RSM experimental design was employed to study the interaction between the independent variables affecting the coagulation, to develop response models and to optimize the process. The effect of three independent variables (pH, coagulant dosage and settling time) on turbidity, and colour removal efficiencies were studied. The results revealed that pH has the most significant effect of the three responses, while the coagulant dosage had a significant effect only on the colour removal efficiencies. Multi-response optimization was employed and it was found that more than 81% reduction in turbidity and 77% reduction in colour can be achieved at the optimum conditions of low pH (pH = 2) and low coagulant (CD = 4 mL) dosage and moderate settling time (ST = 21 min). It has been concluded that MO can be effectively used as an alternative to chemical coagulants for the treatment of real textile effluents. Moreover, due to the small amount requirement for coagulant dosage and short settling time, MO can be considered economical for large scale industrial applications.
Acknowledgments
The author gratefully acknowledges the local textile company for providing the wastewater samples.
Disclosure statement
No potential conflict of interest was reported by the author.
References
- Adesina, O. A., Abdulkareem, F., Yusuff, A. S., Lala, M., & Okewale, A. (2019). Response surface methodology approach to optimization of process parameter for coagulation process of surface water using Moringa oleifera seed. South African Journal of Chemical Engineering, 28, 46–51. doi:10.1016/j.sajce.2019.02.002
- Ahmad, S.W. (2018). Dye removal from textile waste water using potato starch: Parametric optimization using Taguchi design of experiments. Archives of Environmental Protection, 44(2), 26–31.
- Ahmaruzzaman, M., & Gupta, V. K. (2011). Rice husk and its ash as low-cost adsorbents in water and wastewater treatment. Industrial & Engineering Chemistry Research, 50(24), 13589–13613. doi:10.1021/ie201477c
- Ali, I. (2012). Removal of arsenate from aqueous solution by electro-coagulation method using Al-Fe electrodes. International Journal of Electrochemical Science., 7, 1898–1907.
- Ali, I. (2018). Microwave assisted economic synthesis of multi walled carbon nanotubes for arsenic species removal in water: Batch and column operations. Journal of Molecular Liquids, 271, 677–685. doi:10.1016/j.molliq.2018.09.021
- Ali, I., Burakova, I., Galunin, E., Burakov, A., Mkrtchyan, E., Melezhik, A., … Grachev, V. (2019). High-Speed and high-capacity removal of methyl orange and malachite green in water using newly developed mesoporous carbon: Kinetic and isotherm studies. ACS Omega, 4(21), 19293–19306. doi:10.1021/acsomega.9b02669
- Almeida, CAd. Vegetable residueof Chayote (Sechium edule SW.) as natural coagulant for treatment of textile wastewater. (2017).
- Ang, W.L., & Mohammad, A.W. (2019). Chapter 9 - Integrated and hybrid process technology. In C. M. Galanakis & E. Agrafioti (Eds.), Sustainable Water and Wastewater Processing (pp. 279–328). Elsevier.
- Ang, W.L., & Mohammad, A.W. (2020). State of the art and sustainability of natural coagulants in water and wastewater treatment. Journal of Cleaner Production, 262, 121267. doi:10.1016/j.jclepro.2020.121267
- Anjaneyulu, Y., Sreedhara Chary, N., & Samuel Suman Raj, D. (2005). Decolourization of industrial effluents - Available methods and emerging technologies - A review. Reviews in Environmental Science and Bio/Technology, 4(4), 245–273. doi:10.1007/s11157-005-1246-z
- Araújo, C.S.T. (2013). Bioremediation of waters contaminated with heavy metals using Moringa oleifera seeds as biosorbent. In Applied Bioremediation - Active and Passive Approaches. IntechOpen Limited.
- Barker, T.B., & Milivojevich, A. (2016). Quality by experimental design. London: CRC Press.
- Bruno, P., Campo, R., Giustra, M.G., De Marchis, M., & Di Bella, G. (2020). Bench scale continuous coagulation-flocculation of saline industrial wastewater contaminated by hydrocarbons. Journal of Water Process Engineering, 34, 101156. doi:10.1016/j.jwpe.2020.101156
- Burakov, A. E., Galunin, E. V., Burakova, I. V., Kucherova, A. E., Agarwal, S., Tkachev, A. G., & Gupta, V. K. (2018). Adsorption of heavy metals on conventional and nanostructured materials for wastewater treatment purposes: A review. Ecotoxicology and Environmental Safety, 148, 702–712. doi:10.1016/j.ecoenv.2017.11.034
- Cuadros, J., Afsin, B., Michalski, J. R., & Ardakani, M. (2012). Fast, microscale-controlled weathering of rhyolitic obsidian to quartz and alunite. Earth and Planetary Science Letters, 353-354, 156–162. doi:10.1016/j.epsl.2012.08.009
- Cui, C., Chen, S., Wang, X., Yuan, G., Jiang, F., Chen, X., & Wang, L. (2019). Characterization of Moringa oleifera roots polysaccharide MRP-1 with anti-inflammatory effect. International Journal of Biological Macromolecules, 132, 844–851. doi:10.1016/j.ijbiomac.2019.03.210
- Dalvand, A., Gholibegloo, E., Ganjali, M. R., Golchinpoor, N., Khazaei, M., Kamani, H., … Mahvi, A. H. (2016). Comparison of Moringa stenopetala seed extract as a clean coagulant with Alum and Moringa stenopetala-Alum hybrid coagulant to remove direct dye from Textile Wastewater. Environmental Science and Pollution Research International, 23(16), 16396–16405. doi:10.1007/s11356-016-6708-z
- Dasgupta, J., Sikder, J., Chakraborty, S., Curcio, S., & Drioli, E. (2015). Remediation of textile effluents by membrane based treatment techniques: A state of the art review. Journal of Environmental Management, 147, 55–72. doi:10.1016/j.jenvman.2014.08.008
- de Souza, M. T. F., de Almeida, C. A., Ambrosio, E., Santos, L. B., Freitas, T. K. F. d S., Manholer, D. D., … Garcia, J. C. (2016). Extraction and use of Cereus peruvianus cactus mucilage in the treatment of textile effluents. Journal of the Taiwan Institute of Chemical Engineers, 67, 174–183. doi:10.1016/j.jtice.2016.07.009
- Demir, M.E., Chehade, G., Dincer, I., Yuzer, B., & Selcuk, H. (2019). Synergistic effects of advanced oxidization reactions in a combination of TiO2 photocatalysis for hydrogen production and wastewater treatment applications. International Journal of Hydrogen Energy, 44(43), 23856–23867. doi:10.1016/j.ijhydene.2019.07.110
- Dil, E. A., Ghaedi, M., Ghaedi, A.M., Asfaram, A., Goudarzi, A., Hajati, S., … Gupta, V. K. (2016). Modeling of quaternary dyes adsorption onto ZnO–NR–AC artificial neural network: Analysis by derivative spectrophotometry. Journal of Industrial and Engineering Chemistry, 34, 186–197. doi:10.1016/j.jiec.2015.11.010
- Dos Santos, T.R.T. (2018). Evaluation of magnetic coagulant (Α-Fe2O3-MO) and its reuse in textile wastewater treatment. Water, Air, and Soil Pollution, 229(3)
- Dotto, J., Fagundes-Klen, M. R., Veit, M. T., Palácio, S. M., & Bergamasco, R. (2019). Performance of different coagulants in the coagulation/flocculation process of textile wastewater. Journal of Cleaner Production, 208, 656–665. doi:10.1016/j.jclepro.2018.10.112
- Freitas, T.K.F.S., Oliveira, V.M., de Souza, M.T.F., Geraldino, H.C.L., Almeida, V.C., Fávaro, S.L., & Garcia, J.C. (2015). Optimization of coagulation-flocculation process for treatment of industrial textile wastewater using okra (A. esculentus) mucilage as natural coagulant. Industrial Crops and Products, 76, 538–544. doi:10.1016/j.indcrop.2015.06.027
- Ghaedi, M., Hajjati, S., Mahmudi, Z., Tyagi, I., Agarwal, S., Maity, A., & Gupta, V.K. (2015). Modeling of competitive ultrasonic assisted removal of the dyes – Methylene blue and Safranin-O using Fe3O4 nanoparticles. Chemical Engineering Journal, 268, 28–37. doi:10.1016/j.cej.2014.12.090
- Gogate, P.R., Thanekar, P.D., & Oke, A.P. (2020). Strategies to improve biological oxidation of real wastewater using cavitation based pre-treatment approaches. Ultrasonics Sonochemistry, 64, 105016 doi:10.1016/j.ultsonch.2020.105016
- Gupta, V. K., Ali, I., Saleh, T. A., Siddiqui, M. N., & Agarwal, S. (2013). Chromium removal from water by activated carbon developed from waste rubber tires. Environmental Science and Pollution Research International, 20(3), 1261–1268. doi:10.1007/s11356-012-0950-9
- Gupta, V. K., Jain, R., Nayak, A., Agarwal, S., & Shrivastava, M. (2011). Removal of the hazardous dye—Tartrazine by photodegradation on titanium dioxide surface. Materials Science and Engineering: C, 31(5), 1062–1067. doi:10.1016/j.msec.2011.03.006
- Gupta, V. K., Rastogi, A., Dwivedi, M. K., & Mohan, D. (1997). Process Development for the removal of zinc and cadmium from wastewater using Slag—A Blast Furnace waste material. Separation Science and Technology, 32(17), 2883–2912. doi:10.1080/01496399708002227
- Gupta, V.K., & Saleh, T.A. (2013). Sorption of pollutants by porous carbon, carbon nanotubes and fullerene- An overview. Environmental Science and Pollution Research International, 20(5), 2828–2843. doi:10.1007/s11356-013-1524-1
- Gupta, V.K., Nayak, A., & Agarwal, S. (2015). Bioadsorbents for remediation of heavy metals: Current status and their future prospects. Environmental Engineering Research, 20(1), 1–18. doi:10.4491/eer.2015.018
- Harrelkas, F., Azizi, A., Yaacoubi, A., Benhammou, A., & Pons, M. N. (2009). Treatment of textile dye effluents using coagulation–flocculation coupled with membrane processes or adsorption on powdered activated carbon. Desalination, 235(1-3), 330–339. doi:10.1016/j.desal.2008.02.012
- Hodaifa, G., Gallardo, P. A. R., García, C. A., Kowalska, M., & Seyedsalehi, M. (2019). Chemical oxidation methods for treatment of real industrial olive oil mill wastewater. Journal of the Taiwan Institute of Chemical Engineers, 97, 247–254. doi:10.1016/j.jtice.2019.02.001
- Hube, S., Eskafi, M., Hrafnkelsdóttir, K. F., Bjarnadóttir, B., Bjarnadóttir, M. Á., Axelsdóttir, S., & Wu, B. (2020). Direct membrane filtration for wastewater treatment and resource recovery: A review. The Science of the Total Environment, 710, 136375 doi:10.1016/j.scitotenv.2019.136375
- Jiang, J.-Q. (2015). The role of coagulation in water treatment. Current Opinion in Chemical Engineering, 8, 36–44. doi:10.1016/j.coche.2015.01.008
- Jorfi, S., Barzegar, G., Ahmadi, M., Darvishi Cheshmeh Soltani, R., Alah Jafarzadeh Haghighifard, N., Takdastan, A., … Abtahi, M. (2016). Enhanced coagulation-photocatalytic treatment of Acid red 73 dye and real textile wastewater using UVA/synthesized MgO nanoparticles. Journal of Environmental Management, 177, 111–118. doi:10.1016/j.jenvman.2016.04.005
- Kang, J., & Treviño, J. (2017). Evaluating Moringa Oleifera, Papaya, and Pumpkin Seed as a Natural Coagulant. International Journal of Life Sciences Research, 5(2), 126–131.
- Kristianto, H., Kurniawan, M.A., & Soetedjo, J.N.M. (2018). Utilization of Papaya Seeds as natural coagulant for synthetic textile coloring agent wastewater treatment. International Journal on Advanced Science, Engineering and Information Technology, 8(5), 2071–2077. doi:10.18517/ijaseit.8.5.3804
- Kumar, K., & Chowdhury, A. (2020). Use of novel nanostructured photocatalysts for the environmental sustainability of wastewater treatments. In S. Hashmi & I.A. Choudhury, (Eds.), Encyclopedia of Renewable and Sustainable Materials (pp. 949–964). Oxford: Elsevier.
- Landázuri, A. C., Villarreal, J. S., Núñez, E. R., Pico, M. M., Lagos, A. S., Caviedes, M., & Espinosa, E. (2018). Experimental evaluation of crushed Moringa oleifera Lam. seeds and powder waste during coagulation-flocculation processes. Journal of Environmental Chemical Engineering, 6(4), 5443–5451. doi:10.1016/j.jece.2018.08.021
- Lundstedt, T., Seifert, E., Abramo, L., Thelin, B., Nyström, Å., Pettersen, J., & Bergman, R. (1998). Experimental design and optimization. Chemometrics and Intelligent Laboratory Systems, 42(1-2), 3–40. doi:10.1016/S0169-7439(98)00065-3
- Manholer, D. D., de Souza, M. T. F., Ambrosio, E., Freitas, T. K. F. d S., Geraldino, H. C. L., & Garcia, J. C. (2019). Coagulation/flocculation of textile effluent using a natural coagulant extracted from Dillenia indica. Water Science and Technology : a Journal of the International Association on Water Pollution Research, 80(5), 979–988. doi:10.2166/wst.2019.342
- Menkiti, M.C., Okoani, A.O., & Ejimofor, M.I. (2018). Adsorptive study of coagulation treatment of paint wastewater using novel Brachystegia eurycoma extract. Applied Water Science, 8(6), 189. doi:10.1007/s13201-018-0836-1
- Mittal, A., Mittal, J., Malviya, A., & Gupta, V. K. (2010). Removal and recovery of Chrysoidine Y from aqueous solutions by waste materials. Journal of Colloid and Interface Science, 344(2), 497–507. doi:10.1016/j.jcis.2010.01.007
- Mohammed Redha, Z., Abdulla Yusuf, H., Amin, R., & Bououdina, M. (2020). The study of photocatalytic degradation of a commercial azo reactive dye in a simple design reusable miniaturized reactor with interchangeable TiO2 nanofilm. Arab Journal of Basic and Applied Sciences, 27(1), 287–298. doi:10.1080/25765299.2020.1800163
- Mohd-Salleh, S.N.A., Mohd-Zin, N.S., & Othman, N. (2019). A review of wastewater treatment using natural material and its potential as aid and composite coagulant. Sains Malaysiana, 48(1), 155–164. doi:10.17576/jsm-2019-4801-18
- Mojet, B., Ebbesen, S., & Lefferts, L. (2010). ChemInform Abstract: Light at the Interface: The Potential of Attenuated Total Reflection Infrared Spectroscopy for Understanding Heterogeneous Catalysis in Water. Chemical Society Reviews, 39(12), 4643–4655. doi:10.1039/c0cs00014k
- Nekouei, F., Nekouei, S., Tyagi, I., & Gupta, V. K. (2015). Kinetic, thermodynamic and isotherm studies for acid blue 129 removal from liquids using copper oxide nanoparticle-modified activated carbon as a novel adsorbent. Journal of Molecular Liquids, 201, 124–133. doi:10.1016/j.molliq.2014.09.027
- Nonfodji, O. M., Fatombi, J. K., Ahoyo, T. A., Osseni, S. A., & Aminou, T. (2020). Performance of Moringa oleifera seeds protein and Moringa oleifera seeds protein-polyaluminum chloride composite coagulant in removing organic matter and antibiotic resistant bacteria from hospital wastewater. Journal of Water Process Engineering, 33, 101103. doi:10.1016/j.jwpe.2019.101103
- Obiora-Okafo, I.A., & Onukwuli, O.D. (2017). Optimization of coagulation-flocculation process for colour removal from Azo dye using natural polymers: Response surface methodological approach. Nigerian Journal of Technology, 36(2), 482–495. doi:10.4314/njt.v36i2.23
- Onukwuli, O.D., Obiora-Okafo, I.A., & Omotioma, M. (2019). Characterization and removal of colour from aqueous solution using bio-coagulants: Response surface methodological approach. Journal of Chemical Technology and Metallurgy, 54(1), 77–89.
- Patel, H., & Vashi, R.T. (2013). Comparison of naturally prepared coagulants for removal of COD and color from textile wastewater. Global Nest Journal, 15(4), 522–528.
- Prabhakaran, G., Manikandan, M., & Boopathi, M. (2020). Treatment of textile effluents by using natural coagulants. Materials Today: Proceedings, doi:10.1016/j.matpr.2020.03.029
- Prasad, R.K. (2009). Color removal from distillery spent wash through coagulation using Moringa oleifera seeds: Use of optimum response surface methodology. Journal of Hazardous Materials, 165(1-3), 804–811. doi:10.1016/j.jhazmat.2008.10.068
- Rajendran, S., Khan, M. M., Gracia, F., Qin, J., Gupta, V. K., & Arumainathan, S. (2016). Ce(3+)-ion-induced visible-light photocatalytic degradation and electrochemical activity of ZnO/CeO2 nanocomposite . Scientific Reports, 6(1), 31641 doi:10.1038/srep31641
- Rambe, A.M. (2018). The use of the Kelor Seeds (Moringa oleifera) as alternative coagulant in waste delivery process of textile industrial waste. in IOP Conference Series: Materials Science and Engineering. doi:10.1088/1757-899X/309/1/012075
- Reddy, D. H. K., Seshaiah, K., Reddy, A.V.R., & Lee, S.M. (2012). Optimization of Cd(II), Cu(II) and Ni(II) biosorption by chemically modified Moringa oleifera leaves powder. Carbohydrate Polymers, 88(3), 1077–1086. doi:10.1016/j.carbpol.2012.01.073
- Riera-Torres, M., Gutiérrez-Bouzán, C., & Crespi, M. (2010). Combination of coagulation–flocculation and nanofiltration techniques for dye removal and water reuse in textile effluents. Desalination, 252(1-3), 53–59. doi:10.1016/j.desal.2009.11.002
- Sadri Moghaddam, S., Alavi Moghaddam, M.R., & Arami, M. (2010). Coagulation/flocculation process for dye removal using sludge from water treatment plant: Optimization through response surface methodology. Journal of Hazardous Materials, 175(1-3), 651–657. doi:10.1016/j.jhazmat.2009.10.058
- Saha, S., Boro, R., & Das, C. (2019). Treatment of tea industry wastewater using coagulation-spinning basket membrane ultrafiltration hybrid system. Journal of Environmental Management, 244, 180–188. doi:10.1016/j.jenvman.2019.05.043
- Saleem, M., & Bachmann, R.T. (2019). A contemporary review on plant-based coagulants for applications in water treatment. Journal of Industrial and Engineering Chemistry, 72, 281–297. doi:10.1016/j.jiec.2018.12.029
- Saleh, T.A., & Gupta, V.K. (2011). Functionalization of tungsten oxide into MWCNT and its application for sunlight-induced degradation of rhodamine B. Journal of Colloid and Interface Science, 362(2), 337–344. doi:10.1016/j.jcis.2011.06.081
- Saleh, T.A., & Gupta, V.K. (2012). Photo-catalyzed degradation of hazardous dye methyl orange by use of a composite catalyst consisting of multi-walled carbon nanotubes and titanium dioxide. Journal of Colloid and Interface Science, 371(1), 101–106. doi:10.1016/j.jcis.2011.12.038
- Saleh, T.A., & Gupta, V.K. (2014). Processing methods, characteristics and adsorption behavior of tire derived carbons: A review. Advances in Colloid and Interface Science, 211, 93–101. doi:10.1016/j.cis.2014.06.006
- Sánchez-Martín, J., Ghebremichael, K., & Beltrán-Heredia, J. (2010). Comparison of single-step and two-step purified coagulants from Moringa oleifera seed for turbidity and DOC removal. Bioresource Technology, 101(15), 6259–6261. doi:10.1016/j.biortech.2010.02.072
- Saravanan, R., Gupta, V.K., Prakash, T., Narayanan, V., & Stephen, A. (2013). Synthesis, characterization and photocatalytic activity of novel Hg doped ZnO nanorods prepared by thermal decomposition method. Journal of Molecular Liquids, 178, 88–93. doi:10.1016/j.molliq.2012.11.012
- Saravanan, R., Joicy, S., Gupta, V. K., Narayanan, V., & Stephen, A. (2013). Visible light induced degradation of methylene blue using CeO2/V2O5 and CeO2/CuO catalysts. Materials Science & Engineering. C, Materials for Biological Applications, 33(8), 4725–4731. doi:10.1016/j.msec.2013.07.034
- Saravanan, R., Karthikeyan, N., Gupta, V. K., Thirumal, E., Thangadurai, P., Narayanan, V., & Stephen, A. (2013). ZnO/Ag nanocomposite: An efficient catalyst for degradation studies of textile effluents under visible light. Materials Science & Engineering C, Materials for Biological Applications, 33(4), 2235–2244. doi:10.1016/j.msec.2013.01.046
- Saravanan, R., Karthikeyan, S., Gupta, V. K., Sekaran, G., Narayanan, V., & Stephen, A. (2013). Enhanced photocatalytic activity of ZnO/CuO nanocomposite for the degradation of textile dye on visible light illumination. Materials Science & Engineering C, Materials for Biological Applications, 33(1), 91–98. doi:10.1016/j.msec.2012.08.011
- Saravanan, R., Khan, M. M., Gupta, V. K., Mosquera, E., Gracia, F., Narayanan, V., & Stephen, A. (2015). ZnO/Ag/Mn2O3 nanocomposite for visible light-induced industrial textile effluent degradation, uric acid and ascorbic acid sensing and antimicrobial activity. RSC Advances, 5(44), 34645–34651. doi:10.1039/C5RA02557E
- Saravanan, R., Mansoob Khan, M., Gupta, V. K., Mosquera, E., Gracia, F., Narayanan, V., & Stephen, A. (2015). ZnO/Ag/CdO nanocomposite for visible light-induced photocatalytic degradation of industrial textile effluents. Journal of Colloid and Interface Science, 452, 126–133. doi:10.1016/j.jcis.2015.04.035
- Saravanan, R., Sacari, E., Gracia, F., Khan, M. M., Mosquera, E., & Gupta, V. K. (2016). Conducting PANI stimulated ZnO system for visible light photocatalytic degradation of coloured dyes. Journal of Molecular Liquids, 221, 1029–1033. doi:10.1016/j.molliq.2016.06.074
- Saritha, V., Karnena, M.K., & Dwarapureddi, B.K. (2019). Exploring natural coagulants as impending alternatives towards sustainable water clarification– A comparative studies of natural coagulants with alum. Journal of Water Process Engineering, 32, 100982. doi:10.1016/j.jwpe.2019.100982
- Šćiban, M., Klašnja, M., Antov, M., & Škrbić, B. (2009). Removal of water turbidity by natural coagulants obtained from chestnut and acorn. Bioresource Technology, 100(24), 6639–6643. doi:10.1016/j.biortech.2009.06.047
- Shamsnejati, S., Chaibakhsh, N., Pendashteh, A. R., & Hayeripour, S. (2015). Mucilaginous seed of Ocimum basilicum as a natural coagulant for textile wastewater treatment. Industrial Crops and Products, 69, 40–47. doi:10.1016/j.indcrop.2015.01.045
- Sher, F., Malik, A., & Liu, H. (2013). Industrial polymer effluent treatment by chemical coagulation and flocculation. Journal of Environmental Chemical Engineering, 1(4), 684–689. doi:10.1016/j.jece.2013.07.003
- Suhas, Gupta, V.K., Carrott, P.J.M., Singh, R., Chaudhary, M., Kushwaha, S. (2016). Cellulose: A review as natural, modified and activated carbon adsorbent. Bioresource Technology, 216, 1066–1076. doi:10.1016/j.biortech.2016.05.106
- Suresh, A., Ganesh, B., & Velmurugan, S. (2019). Decolorisation of azo dye (Congo red) from synthetic solution using natural coagulants. Journal of Surface Science and Technology, 35(3-4), 151–154.
- Tiaiba, M. (2018). Study of chemical coagulation conditions for a disperse red dye removal from aqueous solutions. Membrane Water Treatment, 9(1), 9–15.
- Torres, N. H., Souza, B. S., Ferreira, L. F. R., Lima, Á. S., Dos Santos, G. N., & Cavalcanti, E. B. (2019). Real textile effluents treatment using coagulation/flocculation followed by electrochemical oxidation process and ecotoxicological assessment. Chemosphere, 236, 124309 doi:10.1016/j.chemosphere.2019.07.040
- Villaseñor-Basulto, D. L., Astudillo-Sánchez, P. D., del Real-Olvera, J., & Bandala, E. R. (2018). Wastewater treatment using Moringa oleifera Lam seeds: A review. Journal of Water Process Engineering, 23, 151–164. doi:10.1016/j.jwpe.2018.03.017
- Vunain, E., Masoamphambe, E. F., Mpeketula, P. M. G., Monjerezi, M., & Etale, A. (2019). Evaluation of coagulating efficiency and water borne pathogens reduction capacity of Moringa oleifera seed powder for treatment of domestic wastewater from Zomba, Malawi. Journal of Environmental Chemical Engineering, 7(3), 103118. doi:10.1016/j.jece.2019.103118
- Wambuguh, D., & Chianelli, R. (2008). Indigo dye waste recovery from blue denim textile effluent: A by-product synergy approach. New Journal of Chemistry, 32(12), 2189. doi:10.1039/b806213g
- Zahrim, A.Y., Tizaoui, C., & Hilal, N. (2011). Coagulation with polymers for nanofiltration pre-treatment of highly concentrated dyes: A review. Desalination, 266(1-3), 1–16. doi:10.1016/j.desal.2010.08.012
- Zhang, M., Zhang, Z., Liu, S., Peng, Y., Chen, J., & Yoo Ki, S. (2020). Ultrasound-assisted electrochemical treatment for phenolic wastewater. Ultrasonics Sonochemistry, 65, 105058 doi:10.1016/j.ultsonch.2020.105058
- Zheng, H., Sun, Y., Guo, J., Li, F., Fan, W., Liao, Y., & Guan, Q. (2014). Characterization and evaluation of dewatering properties of PADB, a highly efficient cationic flocculant. Industrial & Engineering Chemistry Research, 53(7), 2572–2582. doi:10.1021/ie403635y