Abstract
Epigenetics has been well understood for its role in cell development; however, it is now known to regulate many processes involved in immune cell activation in a variety of cells. The skin maintains homeostasis via crosstalk between immune and non-immune cells. Disruption of normal epigenetic regulation in these cells may alter the transcription of immune-regulatory factors and affect the immunological balance in the skin. This review summarizes recent evidence for the epigenetic regulation of skin immunity. Much of what is known about epigenetic involvement in skin immunity is associated with DNA methylation. This review focuses on epigenetic regulation of histone modification and chromatin remodeling and describes their role in the transcriptional regulation of immune-regulatory factors. While much is still unknown regarding the regulation of skin immunity via histone modification or chromatin remodeling, these processes may underlie the pathogenesis of chronic cutaneous immune disorders.
1. Introduction
The skin is an organ that is constantly exposed to external stimuli and functions as the first-line of defense against invasion to initiate immune responses [Citation1]. It maintains homeostasis by rapidly responding to external environmental signals that are sensed by the outermost layer of the skin, the epidermis. Upon receiving external stimuli, epidermal cells are first activated by themselves, and then, activate surrounding epidermal cells and immune cells [Citation2–4]. This strengthens both the structural and immunological barriers of the skin, and preemptively prepares the skin for a putative invasion by external stimuli. The activated epidermal cells and responding immune cells also possess a rapid recovery system that enables a quick return to the steady state after exposure to external stimuli. The plasticity of epidermal and immune cells, which can flexibly shift between steady and activated states, is therefore responsible for the maintenance of skin homeostasis [Citation1,Citation5]. Crosstalk with immune cells is also active in other non-immune skin cells, including fibroblasts, nerve cells, and vascular endothelial cells. Thus, non-immune cells as well as immune cells support skin immunity through the reciprocal production of immune-regulatory factors. Disruption of the appropriate crosstalk between these cells results in prolonged inflammation as well as abnormal activation and differentiation of skin component cells, which may manifest as chronic inflammatory skin diseases, such as psoriasis and atopic dermatitis [Citation1,Citation5].
Epigenetics is defined as the mechanisms that regulate transcription without altering DNA sequences [Citation6]. It is unlikely that genetic mutations occur within a short period during which skin component cells, including immune cells, epidermal or follicle cells, nerve cells, fibroblasts and vascular endothelial cells, respond to external stimuli. How do these cells then control the expression of genes necessary for cell activation and subsequent repression within such a short period? One possibility is that an epigenetic regulatory mechanism is evoked in which cells regulate transcription by altering the structure of genomic DNA in response to stimuli [Citation6]. Given that the skin is constantly exposed to environmental signals, epigenetics may play a pivotal role in the regulation of skin immunity.
This review begins with an overview of the basics of epigenetic systems, followed by a description of chromatin structure, types of histone modification and their individual roles, and the enzymes involved in histone modification and chromatin remodeling. We then summarize the mechanism by which skin immunity is regulated via crosstalk between immune and non-immune cells, and discuss the current understanding and future perspectives of epigenetic regulation in skin immunity based on the latest findings.
2. Structure of chromatin and regulation of gene expression
Human cells contain two meters of genomic DNA that is tightly folded and packed within the nucleus. Genomic DNA forms a secondary structure referred to as chromatin that fits into a limited space [Citation7]. The basic unit of chromatin, the nucleosome, is consisted of 147 bp genomic DNA and a core histone octamer. DNA is negatively charged and histones are positively charged, and the opposing charges allow DNA to wrap itself tightly around the histone octamer to form a nucleosome. Initiation of transcription requires the binding of RNA polymerase II and several basic transcription factors, called TFIIA and TFIIB, bind to promoters located near the transcription start sites [Citation8]. Sequence-specific DNA-binding transcription factors (TFs) are involved in the enhancement of transcription. TFs bind to enhancers and cause genomic DNA to form looped structures that shorten the distance between enhancers and promoters, thereby promoting the transcription of the target genes. Transcriptional activity is also closely related to the degree of DNA condensation associated with chromatin structure [Citation6,Citation8]. Tightly packed chromatin, called closed chromatin or heterochromatin, restricts the access of RNA polymerase II and the transcription factors to the regulatory sites, and consequently, suppresses the expression of target genes. Open chromatin or euchromatin that is less condensed allows easier access of the transcriptional machinery to DNA, thus setting target genes to be more actively transcribed.
3. Histone modification
The mechanisms of epigenetic regulation are classified into three major categories: DNA methylation, post-translational histone modification, and chromatin remodeling [Citation6,Citation9,Citation10]. This review focuses on the latter two categories. Post-translational histone modification facilitates the addition or removal of acetyl or methyl groups on histones via covalent binding by histone modifying enzymes [Citation11]. Acetylation decreases the positive charge of histones and consequently reduces the affinity between histones and DNA. This results in the loosening of chromatin structure which is associated with enhanced transcriptional activity. The chromatin status and associated transcriptional activity regarding methylation depend on the residues to be modified and the type of methylation [Citation7]. Combinations of different histone modifications are associated with the relaxation or condensation of chromatin structures, affecting transcriptional activity. The histone modifying enzymes that typically mediate the addition or removal of chemical groups on histones via covalent binding are referred to as epigenetic players. These include the ‘writers’ that introduce chemical modification on histones, ‘readers’ that recognize these modifications, and ‘erasers’ that remove the modification from histones [Citation12]. The representative histone modification enzymes are summarized in .
Table 1. Representative histone modification enzymes and their functions [Citation51–60].
4. Chromatin remodeling
ATP-dependent chromatin remodeling complexes facilitate chromatin repositioning in a non-covalent manner by utilizing energy from the hydrolysis of ATP. Examples of chromatin repositioning include nucleosome sliding, unwrapping, histone removal, and nucleosome reorganization [Citation13]. Each phenomenon is facilitated by different multi-subunit chromatin remodeling complexes, including switch/sucrose-non-fermenting (SWI/SNF), nucleosome remodeling deacetylase (Mi-2/NuRD), imitation switch (ISWI), and inositol requiring 80 (INO80) () [Citation14–17]. Chromatin dynamics induced by the ATP-dependent chromatin remodeling complexes affect the ability of the transcriptional machinery, including histone modifying enzymes and/or DNA-binding transcription factors, to access DNA and exert their functions. These complexes work in concert with their corresponding transcriptional machinery and are thus involved in both activation and repression of transcription [Citation18,Citation19].
Table 2. Components of ATP-dependent nucleosome remodeling complexes [Citation14–17].
5. Epigenetic dysregulation of immune cells in skin immunity
Epigenetic dysfunction of immune cells has a direct impact on skin immunity. Gene expression during the development and activation of immune cells is supported by changes in the chromatin environment. A comprehensive analysis of the chromatin environment in immune cells of normal skin was reported by Q. Liu and colleagues [Citation20]. Their findings that the chromatin accessibility of dermal macrophages is more similar to that of endothelial cells than that of other myeloid cells are of great interest in terms of cell plasticity. In immune cells under inflammatory conditions, dysfunction of histone modifying enzymes or chromatin remodeling complexes can cause changes in the chromatin environment, and thus, inappropriately activate or inactivate the corresponding DNA-binding transcription factors. This may lead to either over- or under-production of cytokines by immune cells with potentially pathogenic outcomes. In skin diseases, atopic dermatitis is a type 2 helper T (Th2) cell- and type 2 innate lymphoid cell (ILC2) -related disease, and psoriasis is a typical Th17 cell-related disease [Citation21,Citation22]. Lichen planus and contact dermatitis are known as Th1-type skin immune diseases. Autoimmune bullous diseases are characterized by the proliferation and activation of a variety of immune cells such as neutrophils, monocytes, eosinophils, mast cells, and B cells [Citation23]. It can be assumed that all of the immune cells involved in these skin disorders have an underlying epigenetic dysfunction, which may be responsible for the immunological imbalance () [Citation24,Citation25]. Many of the epigenetic regulators of histone modification and chromatin remodeling are expressed and functional during T cell development and activation [Citation26,Citation27]. The biological effects and target genes of these regulators are increasingly being reported, however, much still remains to be elucidated regarding the types and mechanisms of the regulators involved in individual skin immune diseases.
Figure 1. Epigenetic regulation of skin immunity. Epigenetic dysregulation of either or both immune and non-immune cells affects the homeostatic balance between these cells via immune-regulatory factors. Disruption of homeostasis induces a skewed immune response. Establishment of epigenetic milieu leads to disease development of chronic cutaneous immune diseases.
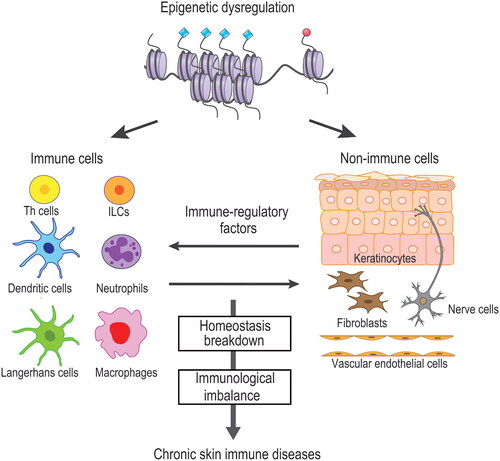
Currently, studies on the epigenetic environment in immune cells from patients with skin diseases, and its association with the relevant transcriptional factors are sparse. One study has reported histone mark signatures in PBMCs from psoriasis patients, showing decreased levels of global histone H4 acetylation compared to healthy controls [Citation28]. However, they make no mention of the possible regulatory regions responsible for the observed quantitative changes in histone modification or the target genes affected. Thus, the detailed mechanisms by which the changes in histone modification regulate the expression of disease-related genes remain unelucidated. In another study, the overall degree of histone acetylation or methylation throughout genomic DNA is suggested to be an indicator of subsequent therapeutic response [Citation29]. There is no difference in expression levels of H3K27me3 between healthy controls and untreated psoriasis patients, however, only those that responded to biological treatment exhibited increased H3K27me3 levels. Recently, the enhancer of zeste homolog 2 (EZH2), a writer of H3K27me3, in regulatory T cells (Tregs) has been focused on its function [Citation30,Citation31]. EZH2 is required for Tregs activation, and Foxp3-specific EZH2 inactivation is known to impair immune tolerance [Citation32]. Although the cellular components in PBMCs that are responsible for the increase in H3K27me3 after biological treatment are unknown, the associations between cell-specific histone modification and therapeutic efficacy will be of considerable interest in the future.
6. Epigenetic dysregulation of epidermal cells in skin immunity
External stress stimuli are received directly by the epidermis, the outermost layer of the skin. The epidermis is mostly composed of keratinocytes, and the productive interplay between keratinocytes and the immune system is dependent on a series of immune-regulatory factors that are produced by keratinocytes upon exposure to external stimuli [Citation3,Citation4]. These factors include pro-inflammatory cytokines, such as IL-1, IL-18, IL-19, IL-20, IL-24, IL-33, IL-36, and TSLP, or chemokines, Cxcl1, Cxcl8, Cxcl9, Cxcl10, Cxcl14, Cxcl16, Ccl20, and Ccl27 [Citation33]. The expression patterns of these molecules are dependent on the type of signals received by the epidermis. Keratinocytes also express pattern recognition receptors (PARs), such as TLRs, and antimicrobial peptides (AMPs), including S100A7-A9, lipocalin, and cathelicidin, all of which are associated with innate immunity [Citation34]. Accumulating evidence suggests that epigenetic players of histone modification and chromatin remodeling functionally regulate epidermal stress response by targeting the factors mentioned above. Loss of EZH1 and EZH2, a histone H3K27 methyltransferase, in hair follicles exhibits delayed wound healing with defective cell proliferation [Citation35]. Derepression of the target genes Ink4b, Ink4a and Arf by EZH1/EZH2-induced loss of H3K27me3 marks is responsible for this delayed wound process [Citation35]. By contrast, the expression and activity of Jumonji domain-containing protein D3 (Jmjd3), a histone H3K27me3 demethylase, are enhanced at the leading edge of the injured epidermis in an in vitro scratch assay [Citation36]. DNA-binding transcription factors work in concert with specific epigenetic players to regulate gene expression programs. During the wound repair process, the NF-kB transcription factor exerts its functions in collaboration with histone modifying enzymes Jmjd3 to regulate the transcription of the target gene Notch1 [Citation37]. Mi-2/NuRD, an ATP-dependent chromatin remodeler, functions as a repressive epigenetic regulator in keratinocytes. Mi-2/NuRD becomes transiently dysfunctional in keratinocytes in response to acute stress caused by barrier disruption [Citation38]. As a result, the AP-1 transcription factor is induced to override Mi-2/NuRD-dependent gene expression programs. Target genes of this process include JUN, COL7A1 and RARA [Citation39].
Reports on epigenetic dysregulation of the epidermis associated with chronic inflammatory skin conditions are limited. EZH2 has been reported to be overexpressed in the epidermis of psoriatic lesional skin compared to normal skin [Citation40,Citation41]. Psoriasis-related cytokines induce human keratinocytes to upregulate the expression of EZH2 and promote the accumulation of H3K27me3 on genome DNA [Citation41]. Additionally, CDK4/6, a cell cycle regulatory gene, drives the activation of EZH2 in keratinocytes that further induces the activation of the collaborating transcription factor STAT3 [Citation40,Citation42]. The activation of this process subsequently transcribes the target gene Nfkbiz, a key inflammatory factor in psoriasis. Other transcription factors presumably activated in a disease-dependent manner in the epidermis of chronically inflamed skin include STAT1, STAT6, NF-kB, AP-1 and IRFs [Citation43]. These transcriptional factors respectively support the transcription of immune-regulatory factors in keratinocytes under the control of their specific epigenetic regulators to exert their functions [Citation8,Citation44]. Therefore, dysregulated epigenetic mechanisms in keratinocytes lead to abnormal production of immune-regulatory factors that may affect the crosstalk with immune cells ().
7. Epigenetic dysregulation of other non-immune cells in skin immunity
Non-immune skin cells, such as fibroblasts, nerve cells, and vascular endothelial cells also play key roles in the regulation of local immunity [Citation45–47]. These cells produce a wide variety of immune-regulatory factors that control the activation or suppression of immune responses. In atopic dermatitis, the enhanced production of Cxcl12 and Ccl19 by dermal fibroblasts contributes to the local migration of Ccr4-positive NKT cells and Ccr7-positive dendritic cells, respectively [Citation48,Citation49]. Peripheral skin nerve cells express receptors for type 2 inflammation-related cytokines, such as IL-4, IL-13, and IL-31, which are responsible for the immune-mediated chronic itch in atopic dermatitis [Citation46]. Vascular endothelial cells are the initial cells with which circulating immune cells in the bloodstream interact when they invade skin tissue. Circulating immune cells migrate to the skin via the expression of adhesion molecules and/or selectins expressed on vascular endothelial cells. Vascular endothelial cells also contribute to the migration of immune cells via chemokines such as Ccl2 and Cxc10 [Citation47].
Thus, all of these non-immune cells also regulate cutaneous immune responses through the production of immune-regulatory factors. Although it is presumed that the epigenetic system in these cells is driven by the surrounding microenvironment and is involved in a series of transcriptional immunoregulatory programs, studies on epigenetic regulation of skin immunity in non-immune cells have only just begun. Nerve cells are reported to be susceptible to epigenetic modification during physiological events, such as trauma and neurological diseases [Citation50]. Thus, it would be interesting to explore the function of regulators regarding histone modification or chromatin remodeling in the regulation of itch-related mediators and their receptors in peripheral nerves. Much is still unknown regarding the epigenetic involvement in the crosstalk between non-immune and immune cells.
8. Conclusion
The importance of epigenetic regulation in the crosstalk between immune and non-immune cells in skin immunity is supported by an increasing number of studies, both from human samples and mouse models. Epigenetic dysregulation of both immune and non-immune cells evoked by environmental factors underlie the immunological imbalance, which may subsequently develop chronic skin immune diseases (). Future studies are essential to delineate epigenetic events that regulate the cellular functions of these cells in specific disease conditions. The reciprocal interplay between epigenetic regulators and DNA-binding transcription factors promotes cells toward disease-specific phenotypes via epigenetic reprogramming (). Understanding the precise mechanisms of epigenetic regulation in the disease context will be important for the development of novel therapeutics targeting epigenetics in cutaneous immunity.
Figure 2. Proposed model of epigenetic regulation in the development and progression of cutaneous immune diseases. Epigenetic regulators and DNA-binding transcription factors (TFs) are mutually recruited to genomic regulatory regions. Interplay between epigenetic regulators and TFs activates target genes associated with the disease. Epigenetic regulators include histone modifying enzymes and chromatin remodeling complexes. TFs and disease-related genes of atopic dermatitis and psoriasis are presented as representative.
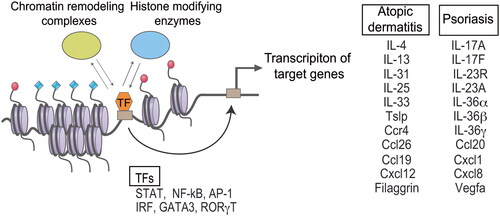
Disclosure statement
No potential conflict of interest was reported by the author(s).
Additional information
Funding
References
- Fuchs E, Blau HM. Tissue stem cells: architects of their niches. Cell Stem Cell. 2020;27(4):532–556.
- Pittelkow MR. Psoriasis: more than skin deep. Nat Med. 2005;11(1):17–18.
- Nestle FO, Di Meglio P, Qin JZ, et al. Skin immune sentinels in health and disease. Nat Rev Immunol. 2009;9(10):679–691.
- Kupper TS, Fuhlbrigge RC. Immune surveillance in the skin: mechanisms and clinical consequences. Nat Rev Immunol. 2004;4(3):211–222.
- Ge Y, Fuchs E. Stretching the limits: from homeostasis to stem cell plasticity in wound healing and cancer. Nat Rev Genet. 2018;19(5):311–325.
- Apostolou E, Hochedlinger K. Chromatin dynamics during cellular reprogramming. Nature. 2013;502(7472):462–471.
- Olins DE, Olins AL. Chromatin history: our view from the bridge. Nat Rev Mol Cell Biol. 2003;4(10):809–814.
- Li B, Carey M, Workman JL. The role of chromatin during transcription. Cell. 2007;128(4):707–719.
- Fuks F. DNA methylation and histone modifications: teaming up to silence genes. Curr Opin Genet Dev. 2005;15(5):490–495.
- Talbert PB, Henikoff S. Histone variants on the move: substrates for chromatin dynamics. Nat Rev Mol Cell Biol. 2017;18(2):115–126.
- Morgan MAJ, Shilatifard A. Reevaluating the roles of histone-modifying enzymes and their associated chromatin modifications in transcriptional regulation. Nat Genet. 2020;52(12):1271–1281.
- Gillette TG, Hill JA. Readers, writers, and erasers: chromatin as the whiteboard of heart disease. Circ Res. 2015;116(7):1245–1253.
- Tong JK, Hassig CA, Schnitzler GR, et al. Chromatin deacetylation by an ATP-dependent nucleosome remodelling complex. Nature. 1998;395(6705):917–921.
- Kadoch C, Hargreaves DC, Hodges C, et al. Proteomic and bioinformatic analysis of mammalian SWI/SNF complexes identifies extensive roles in human malignancy. Nat Genet. 2013;45(6):592–601.
- Zhang Y, LeRoy G, Seelig HP, et al. The dermatomyositis-specific autoantigen Mi2 is a component of a complex containing histone deacetylase and nucleosome remodeling activities. Cell. 1998;95(2):279–289.
- Bartholomew B. ISWI chromatin remodeling: one primary actor or a coordinated effort? Curr Opin Struct Biol. 2014;24:150–155.
- Tosi A, Haas C, Herzog F, et al. Structure and subunit topology of the INO80 chromatin remodeler and its nucleosome complex. Cell. 2013;154(6):1207–1219.
- Zhang J, Jackson AF, Naito T, et al. Harnessing of the nucleosome-remodeling-deacetylase complex controls lymphocyte development and prevents leukemogenesis. Nat Immunol. 2011;13(1):86–94.
- Swygert SG, Peterson CL. Chromatin dynamics: interplay between remodeling enzymes and histone modifications. Biochim Biophys Acta. 2014;1839(8):728–736.
- Liu Q, Zaba LC, Satpathy AT, et al. Chromatin accessibility landscapes of skin cells in systemic sclerosis nominate dendritic cells in disease pathogenesis. Nat Commun. 2020;11(1):5843.
- O'Shea JJ, Paul WE. Mechanisms underlying lineage commitment and plasticity of helper CD4+ T cells. Science. 2010;327(5969):1098–1102.
- Cavani A, Albanesi C, Traidl C, et al. Effector and regulatory T cells in allergic contact dermatitis. Trends Immunol. 2001;22(3):118–120.
- Egami S, Yamagami J, Amagai M. Autoimmune bullous skin diseases, pemphigus and pemphigoid. J Allergy Clin Immunol. 2020;145(4):1031–1047.
- Luo A, Leach ST, Barres R, et al. The microbiota and epigenetic regulation of T helper 17/regulatory T cells: in search of a balanced immune system. Front Immunol. 2017;8:417.
- Obata Y, Furusawa Y, Hase K. Epigenetic modifications of the immune system in health and disease. Immunol Cell Biol. 2015;93(3):226–232.
- Weng NP, Araki Y, Subedi K. The molecular basis of the memory T cell response: differential gene expression and its epigenetic regulation. Nat Rev Immunol. 2012;12(4):306–315.
- Ansel KM, Lee DU, Rao A. An epigenetic view of helper T cell differentiation. Nat Immunol. 2003;4(7):616–623.
- Zhang P, Su Y, Zhao M, et al. Abnormal histone modifications in PBMCs from patients with psoriasis vulgaris. Eur J Dermatol. 2011;21(4):552–557.
- Ovejero-Benito MC, Reolid A, Sanchez-Jimenez P, et al. Histone modifications associated with biological drug response in moderate-to-severe psoriasis. Exp Dermatol. 2018;27(12):1361–1371.
- Wang D, Quiros J, Mahuron K, et al. Targeting EZH2 reprograms intratumoral regulatory T cells to enhance cancer immunity. Cell Rep. 2018;23(11):3262–3274.
- Huang J, Zhang J, Guo Z, et al. Easy or Not-The advances of EZH2 in regulating T cell development, differentiation, and activation in antitumor immunity. Front Immunol. 2021;12:741302.
- DuPage M, Chopra G, Quiros J, et al. The chromatin-modifying enzyme Ezh2 is critical for the maintenance of regulatory T cell identity after activation. Immunity. 2015;42(2):227–238.
- Feldmeyer L, Keller M, Niklaus G, et al. The inflammasome mediates UVB-induced activation and secretion of interleukin-1beta by keratinocytes. Curr Biol. 2007;17(13):1140–1145.
- Miller LS, Modlin RL. Toll-like receptors in the skin. Semin Immunopathol. 2007;29(1):15–26.
- Ezhkova E, Lien WH, Stokes N, et al. EZH1 and EZH2 cogovern histone H3K27 trimethylation and are essential for hair follicle homeostasis and wound repair. Genes Dev. 2011;25(5):485–498.
- Na J, Lee K, Na W, et al. Histone H3K27 demethylase JMJD3 in cooperation with NF-kappaB regulates keratinocyte wound healing. J Invest Dermatol. 2016;136(4):847–858.
- Na J, Shin JY, Jeong H, et al. JMJD3 and NF-kappaB-dependent activation of Notch1 gene is required for keratinocyte migration during skin wound healing. Sci Rep. 2017;7(1):6494.
- Shibata S. Chromatin dynamics and epigenetics in skin stress adaptation. J Dermatol Sci. 2021;103(2):66–72.
- Shibata S, Kashiwagi M, Morgan BA, et al. Functional interactions between Mi-2beta and AP1 complexes control response and recovery from skin barrier disruption. J Exp Med. 2020;217(3):jem.20182402.
- Muller A, Dickmanns A, Resch C, et al. The CDK4/6-EZH2 pathway is a potential therapeutic target for psoriasis. J Clin Invest. 2020;130(11):5765–5781.
- Zhang T, Yang L, Ke Y, et al. EZH2-dependent epigenetic modulation of histone H3 lysine-27 contributes to psoriasis by promoting keratinocyte proliferation. Cell Death Dis. 2020;11(10):826.
- Sano S, Chan KS, Carbajal S, et al. Stat3 links activated keratinocytes and immunocytes required for development of psoriasis in a novel transgenic mouse model. Nat Med. 2005;11(1):43–49.
- Platanitis E, Decker T. Regulatory networks involving STATs, IRFs, and NFkappaB in inflammation. Front Immunol. 2018;9:2542.
- Roh TY, Cuddapah S, Zhao K. Active chromatin domains are defined by acetylation islands revealed by genome-wide mapping. Genes Dev. 2005;19(5):542–552.
- Davidson S, Coles M, Thomas T, et al. Fibroblasts as immune regulators in infection, inflammation and cancer. Nat Rev Immunol. 2021;21(11):704–717.
- Steinhoff M, Ahmad F, Pandey A, et al. Neuroimmune communication regulating pruritus in atopic dermatitis. J Allergy Clin Immunol. 2022;149(6):1875–1898.
- Amersfoort J, Eelen G, Carmeliet P. Immunomodulation by endothelial cells - partnering up with the immune system? Nat Rev Immunol. 2022;22(9):576–588.
- Sun Z, Kim JH, Kim SH, et al. Skin-resident natural killer T cells participate in cutaneous allergic inflammation in atopic dermatitis. J Allergy Clin Immunol. 2021;147(5):1764–1777.
- He H, Suryawanshi H, Morozov P, et al. Single-cell transcriptome analysis of human skin identifies novel fibroblast subpopulation and enrichment of immune subsets in atopic dermatitis. J Allergy Clin Immunol. 2020;145(6):1615–1628.
- Landgrave-Gomez J, Mercado-Gomez O, Guevara-Guzman R. Epigenetic mechanisms in neurological and neurodegenerative diseases. Front Cell Neurosci. 2015;9:58.
- Demyanenko S, Sharifulina S. The role of post-translational acetylation and deacetylation of signaling proteins and transcription factors after cerebral ischemia: facts and hypotheses. Int J Mol Sci. 2021;22(15):7947.
- Schneider A, Chatterjee S, Bousiges O, et al. Acetyltransferases (HATs) as targets for neurological therapeutics. Neurotherapeutics. 2013;10(4):568–588.
- Hyun K, Jeon J, Park K, et al. Writing, erasing and reading histone lysine methylations. Exp Mol Med. 2017;49(4):e324.
- Shilatifard A. The COMPASS family of histone H3K4 methylases: mechanisms of regulation in development and disease pathogenesis. Annu Rev Biochem. 2012;81:65–95.
- Shi Y, Lan F, Matson C, et al. Histone demethylation mediated by the nuclear amine oxidase homolog LSD1. Cell. 2004;119(7):941–953.
- Hojfeldt JW, Agger K, Helin K. Histone lysine demethylases as targets for anticancer therapy. Nat Rev Drug Discov. 2013;12(12):917–930.
- Pirrotta V. Polycombing the genome: pcG, trxG, and chromatin silencing. Cell. 1998;93(3):333–336.
- Cao R, Wang L, Wang H, et al. Role of histone H3 lysine 27 methylation in polycomb-group silencing. Science. 2002;298(5595):1039–1043.
- Agger K, Cloos PA, Christensen J, et al. UTX and JMJD3 are histone H3K27 demethylases involved in HOX gene regulation and development. Nature. 2007;449(7163):731–734.
- De Santa F, Totaro MG, Prosperini E, et al. The histone H3 lysine-27 demethylase Jmjd3 links inflammation to inhibition of polycomb-mediated gene silencing. Cell. 2007;130(6):1083–1094.