ABSTRACT
The conjugating green algae (Zygnematophyceae) are the closest relatives of land plants and hence are of great evolutionary interest. Besides the popular placoderm desmids and the filamentous species, there is an underappreciated diversity of unicellular zygnematophytes with a much “simpler” morphology and smooth cell walls – traditionally referred to as “saccoderm desmids”. These saccoderm desmids have a broad geographic distribution and are ecologically diverse. Many species inhabit terrestrial habitats such as dead wood, rock surfaces and glacial ice. Furthermore, several of the saccoderm genera have turned out to be highly polyphyletic and are typically poorly captured by environmental sequencing approaches. One of these genera is Mesotaenium Nägeli, with ~70 described species and infraspecific taxa united only by a relatively simple (plate- or ribbon-like) chloroplast structure. Here, we shed some light on these inconspicuous yet important members of the algal flora and present an updated rbcL gene phylogeny of the conjugating green algae, including several new lineages of Mesotaenium-like zygnematophytes. We depict the subtle morphological differences among these lineages and discuss our updated phylogeny in the light of ecology and cell biology. In addition, we review published knowledge on photoprotective strategies of zygnematophytes, the latest insights into their evolutionary innovations, and address some technical challenges in exploring this elusive group of microalgae. Some new observations of saccoderm desmids in undersampled habitats and of their microbial associates (e.g., parasites) point to interesting avenues for future research.
Introduction
It is now well established that the conjugating green algae (Zygnematophyceae , Streptophyta) represent the sister clade of all land plants (); Cheng et al. (Citation2019); Timme, Bachvaroff, & Delwiche (Citation2012); Wickett et al. (Citation2014); Wodniok et al., Citation2011). Hence, these algae are of special interest as they can provide information on how members of the “green lineage” (Viridiplantae) became multicellular and conquered the land in a process termed “terrestrialization” (Delaux, Nanda, Mathé, Sejalon-Delmas, & Dunand, Citation2012; Gerrienne, Servais, & Vecoli, Citation2016). The sequence of evolutionary events and the physiological adaptations that might have paved the way to a “life on land” represent an exciting and timely field of research that has already led to some view-changing insights (Cannell et al., Citation2020; Cheng et al., Citation2019; de Vries, Curtis, Gould, & Archibald, Citation2018; Wang et al., Citation2021). Several cellular and metabolic features that were initially known only from land plants have also been found in streptophyte green algae. This includes a number of specific stress responses (de Vries et al., Citation2020; Holzinger et al., Citation2014), homologues of phytohormone receptors (de Vries et al., Citation2018; Sun et al., Citation2019), and key enzymes of the phenylpropanoid pathway (de Vries, de Vries, Slamovits, Rose, & Archibald, Citation2017). Furthermore, the evolutionary importance of the zygnematophytes was demonstrated in a genomic study that revealed horizontal gene transfer from soil bacteria to unicellular, terrestrial representatives of this algal class, most notably genes encoding homologues of GRAS transcription factors and PYR/PYL/RCAR-like abscisic acid receptors, which might have had roles in the terrestrialization of these algae (Cheng et al., Citation2019).
Figure 1. Evolutionary history of the Zygnematophyceae and depiction of the three morphologically defined groups of these algae. (a) Schematic phylogenetic tree of the Streptophyta showing the sister relationship of the Zygnematophyceae and land plants, and the internal diversity of zygnematophytes. Note that the saccoderm desmids plus the filamentous forms (traditionally referred to as “Zygnematales”) are paraphyletic. Most of the internal phylogeny is unresolved except the deepest-branching position of Spirogloea. (b) Three traditional groups of zygnematophytes defined by gross cell morphology: filamentous zygnematophytes (Spirogyra spp.), placoderm desmids (Micrasterias sp.) with isthmus and obvious symmetry, and saccoderm desmids without isthmus and cell wall ornamentations (strain SAG 12.97, for details see main text).
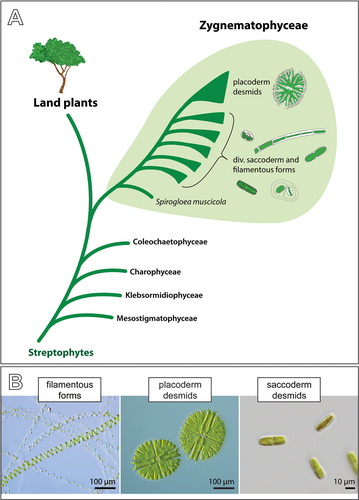
The Zygnematophyceae are stunningly diverse in terms of cellular organization, physiology and ecology (Brook & Williamson, Citation2010; Coesel & Meesters, Citation2007; Ettl & Gärtner, Citation2014; Guiry, Citation2013; Hall & McCourt, Citation2015). They probably encompass far more than the ca. 4,000 described species (Gerrath, Citation1993; Gontcharov, Citation2008; Šťastný & Kouwets, Citation2012), which are traditionally divided into three groups: the placoderm desmids, the saccoderm desmids and the filamentous forms ()). Most in-depth studies on cell biology, ecology and genetics have been on the beautiful placoderm desmids, which are characterized by two symmetrical semi-cells, and on the filamentous species (e.g., de Vries et al., Citation2018; Hainz, Wöber, & Schagerl, Citation2009; Holzinger & Pichrtová, Citation2016; Kawai et al., Citation2022; Lütz-Meindl, Citation2016; Stastny, Skaloud, Langenbach, Nemjova, & Neustupa, Citation2013; Zhou, Wilkens, Hanelt, & von Schwartzenberg, Citation2021). The unicellular, structurally much simpler saccoderm desmids have not had as much attention in the more recent past – probably due to their relatively plain appearance and the difficulties in differentiating species and genera. In fact, the saccoderm desmids have turned out to be highly polyphyletic (Gontcharov, Citation2008; Gontcharov, Marin, & Melkonian, Citation2003, Citation2004). However, they are still lumped into a few ill-defined genera, most importantly Mesotaenium and Cylindrocystis (Guiry & Guiry, Citation2021), and their true diversity remains largely unrecognized (see also Mollenhauer, Citation1986). This is an impediment to science, as the saccoderm desmids vary greatly in ecology and cellular adaptations. They show a wide geographic distribution (including polar regions) and occur in habitats that vary strongly in temperature, chemical conditions and water availability (e.g., Busch & Hess, Citation2021; West & West, Citation1904; Williamson et al., Citation2020). Most notably, many saccoderm desmids colonize terrestrial habitats (Brook & Williamson, Citation2010; Ettl & Gärtner, Citation2014; Fučíková, Hall, Johansen, & Lowe, Citation2008) and might be valuable for exploring adaptive strategies to terrestrial stressors (Cheng et al., Citation2019).
In this work, we explored saccoderm desmids of the Mesotaenium type, i.e., unicellular zygnematophytes with a ribbon- or plate-like chloroplast, and provide an updated molecular phylogeny with 12 distinct lineages of these algae. A new, provisional clade nomenclature emphasizes the true genetic diversity of these organisms and enables unambiguous communication about them. Based on this system, we summarize the structural and ecological variations found in Mesotaenium-like zygnematophytes and discuss their photoprotective adaptations, which we trust will inspire future research on these underappreciated microalgae.
Material and methods
Sampling, isolation and maintenance of algal strains
Natural samples (mostly algal biofilms on surfaces) were collected at several sites in Germany and in the Great Smoky Mountains, USA, as listed in Supplementary table S1. The samples were suspended in water, and single algal cells were isolated with a micropipette, washed in sterile water and transferred into the liquid growth medium Waris-H (McFadden & Melkonian, Citation1986). After several weeks of growth under artificial light (white LEDs, photon fluence rate 30 µmol m−2 s−1, 14:10 h light–dark cycle) at 16°C, the resulting cells were ultrasonicated on ice (max. 10 s) with the XL-2000 ultrasonicator (Misonix Inc., USA) or the UW 2070 ultrasonicator (Bandeline electronic, DE) to liberate cells from the mucilage and sprayed onto solidified culture medium Waris-H (1.5% agar) with pressurized air. After a few days of incubation at 16°C, bacteria-free cells picked and transferred to liquid culture medium were used as sources of the axenic cultures used in this study. Very homogeneous natural samples of Mesotaenium-like zygnematophytes were directly ultrasonicated and spray-plated as detailed above. The liquid cultures were maintained at a photon fluence rate of 30 µmol m−2 s−1, a 14:10 h light–dark cycle, at 16°C, and were subcultured about every 2 months. Cultures on agar slants (solidified Waris-H) were maintained at 10°C and subcultured every 6 months. As indicated in Supplementary table S1, several of the studied strains were obtained from public culture collections, namely the Culture Collection of Algae at the University of Cologne (CCAC, now Central Collection of Algal Cultures in Essen, Germany), Culture Collection of Algae at Göttingen University (SAG, Germany) and the Coimbra Culture Collection of Algae (ACOI, Portugal). The strains isolated during this study can be obtained from the corresponding author (SH) upon request.
Light microscopy and photography
Images of algal growth types were taken with a Nikon D3100 digital single-lens reflex camera (Nikon, Tokyo, Japan) equipped with RAYNOX Macro Conversion Lenses (Yoshida Industry Co., Ltd., Tokyo, Japan). Regular brightfield microscopy and photodocumentation of cultures used the Motic AE2000 inverted microscope (Motic Hong Kong Limited, Hong Kong) equipped with a MikroLive 6.4MP CMOS camera (MikroLive, Oppenau). For high-resolution imaging, the Zeiss IM35 inverted microscope (Carl Zeiss, Oberkochen, Germany) equipped with the objective lenses Plan 40×/0.65 and Planapochromat 63×/1.4, electronic flash and the Canon EOS 6D digital single-lens reflex camera (Canon, Tokyo, Japan) was used, or the Zeiss Axio Observer inverted microscope equipped with the objective lenses Plan-Neofluar 40×/1.3 and Plan-Neofluar 100×/1.3 and the Axiocam 512 colour (Carl Zeiss, Oberkochen, Germany) was used. The colour balance and contrast of micrographs were adjusted with Photoshop CS4 (Adobe Inc., California, USA).
DNA sequencing, alignment and molecular phylogenetics
Algal material from 2 ml of a running culture was collected by centrifugation (5,000 g, 5 min), resuspended in sterile water and lysed by ultrasonication on ice (5 × 5 s) with the XL-2000 ultrasonicator or by grinding in liquid nitrogen. In the case of ultrasonicated material, insoluble debris was pelleted by centrifugation (5,000 g, 1 min) and the supernatant was used directly as a template for PCR (details below). The ground material was subjected to Chelex extraction as follows: 1–10 µl of the algal suspension/frozen material was mixed with 50 µl Tris-HCl buffer (10 mM; pH 8.5), Chelex 100 beads (5%; Sigma-Aldrich) and 2 µl proteinase K (20 mg ml–1; Thermo Fisher Scientific, Massachuesetts, USA), then incubated at 56°C for 45 min and finally at 98°C for 20 min. The samples were centrifuged at 17 000 g for 3 min, and the supernatant was used for PCR. The chloroplast-encoded gene for the large chain of the ribulose bisphosphate carboxylase (rbcL) was amplified by a semi-nested PCR with the primers MaGo1F, MaGo2F and MaGo3R (Gontcharov et al., Citation2004) and Invitrogen Taq DNA Polymerase (Thermo Fisher Scientific, Massachuesetts, USA) or the repliQa HiFi ToughMix (Quanta Bio, Massachuesetts, USA), sequenced and assembled as previously described (Busch & Hess, Citation2021). The generated rbcL gene sequences have been deposited in GenBank under the accession numbers OM241454–OM241471. The sequences were manually aligned with rbcL gene sequences of other Zygnematophyceae (alignment from Busch & Hess, Citation2021) using the SeaView 4.5.4 alignment editor (Galtier, Gouy, & Gautier, Citation1996; Gouy, Guindon, & Gascuel, Citation2010). The resulting dataset of 92 sequences and 1295 sites (including all codon positions) was subjected to phylogenetic inferences with neighbour joining (NJ) and maximum likelihood (ML) methods using MEGA software version X (Kumar et al., Citation2018). For ML analyses, we applied the GTR+I +G model (discrete Gamma distribution; five categories). Branch support was assessed with 1000 bootstrap repetitions.
Results and discussion
Genetic and phenotypic diversity of Mesotaenium-like zygnematophytes
The structurally simplest zygnematophytes have mainly been assigned to the genus Mesotaenium. These inconspicuous, unicellular algae are characterized by a smooth cell wall and a more or less ribbon-like plastid (Nägeli, Citation1849). According to AlgaeBase, the genus Mesotaenium contains 29 recognized species and 39 infraspecific taxa (including synonyms; Guiry & Guiry, Citation2021), most of which were described on the basis of gross morphological characters such as the cell shape and size of vegetative material. Unfortunately, the lack of meaningful original descriptions has complicated species identification of these life forms, which is reflected in the conflicting information in different monographs about desmids (e.g., Brook & Williamson, Citation2010; Coesel & Meesters, Citation2007; Ettl & Gärtner, Citation2014; Lenzenweger, Citation2003). Molecular sequence information for a few of these species revealed that the genus Mesotaenium is polyphyletic and that Mesotaenium-like zygnematophytes form at least six separate lineages within the conjugating green algae (Busch & Hess, Citation2021; Gontcharov, Citation2008; Gontcharov & Melkonian, Citation2010). Despite the available phylogenetic information, most of these lineages have not been studied in detail and we lack proper genus names for them. This leads to a general underappreciation of their actual genetic and ecological diversity. However, exceptions are the Mesotaenium-like zygnematophytes of the genera Ancylonema Berggren and Serritaenia A.Busch & S.Hess, which have recently been subjected to a more detailed characterization and a taxonomic treatment (Busch & Hess, Citation2021; Procházková, Řezanka, Nedbalová, & Remias, Citation2021). Increasing amounts of data for certain strains on the genomic, cell biological and ecological levels necessitates a clear nomenclatural separation of distinct phylogenetic lineages to avoid a mix-up in evolutionary interpretations. The genome-sequenced strain SAG 12.97, for example, has repeatedly been referred to as “Mesotaenium endlicherianum” (Cheng et al., Citation2019; Gontcharov, Citation2008; Gontcharov et al., Citation2003, Citation2004; Matasci et al., Citation2014), although, in our opinion, it does not match the original description of that species very well (Nägeli, Citation1849). Even though taxonomic problems will take much more time and effort to be resolved (e.g., by assessing original material from several species in question), an unambiguous naming system for the different lineages of Mesotaenium-like zygnematophytes and more biological data on them is desirable.
To further explore the diversity of Mesotaenium-like zygnematophytes, we sampled various aquatic and terrestrial habitats (see below for details) and obtained additional strains from public and private culture collections. In total, we studied 31 strains (listed in Supplementary table S1) and inferred a phylogenetic tree of the Zygnematophyceae based on the most widely available genetic marker for that algal class, the gene for the large chain of the ribulose bisphosphate carboxylase (rbcL gene). As shown in several published phylogenies, this gene is unable to resolve the deepest branches within the phylogeny but was well suited to differentiate new zygnematophycean lineages at the genus level (). Our culture-based approach resulted in the identification of 12 lineages of Mesotaenium-like zygnematophytes, which are phylogenetically distinct; several of them could be distinguished by morphology as well (see below for details). Two lineages are credibly assigned to existing genera, namely the Ancylonema lineage and the Serritaenia lineage (; Busch & Hess, Citation2021; Procházková et al., Citation2021). Taxonomic assignment of strains from other lineages will need careful re-evaluation, as detailed in the lineage-specific sections below. For these lineages, we introduce a provisional nomenclature from “Meso-1” to “Meso-10” (). Similar approaches have been used to name phylogenetic clades in other groups of microbial eukaryotes, e.g., in the rhizarians (e.g., Bass & Cavalier-Smith, Citation2004; Bass et al., Citation2009), alveolates (e.g., Guillou et al., Citation2008) and stramenopiles (e.g., Massana et al., Citation2004; Massana, Del Campo, Sieracki, Audic, & Logares, Citation2014). Some lineages in our phylogeny are directly related to each other but still have distinct designations (e.g., Meso-1, Meso-2 and the Serritaenia lineage). This is based on clear-cut phenotypic differences and marked genetic distances.
Figure 2. Maximum likelihood phylogeny of the Zygnematophyceae inferred from rbcL gene sequences revealing 12 lineages of Mesotaenium-like zygnematophytes (highlighted in green). Bootstrap support values (1000 replicates) are shown at the branches (ML/NJ), except for those with full support (100%; bold branches) or support below 50% with the ML inference (omitted). New sequences generated in this study are in bold and Mesotaenium-like zygnematophytes with genome or transcriptome data are denoted by stars. The two grey columns on the right indicate the habitat types (AE = aerophytic, SU = submerged, ICE = ice/snow) and photoprotective adaptations (see legend at the bottom of the figure) of Mesotaenium-like algae and some other zygnematophytes. The scale bar represents 0.05 expected substitutions per site.
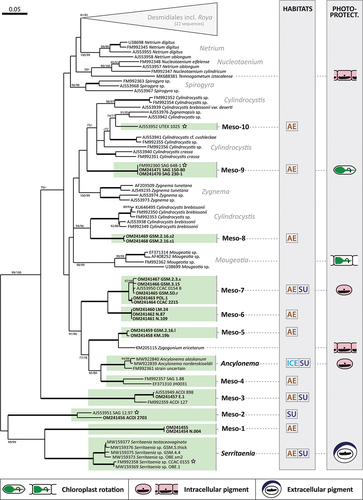
An overview of the morphological diversity of Mesotaenium-like zygnematophytes from different lineages is given in . All cells have a smooth cell wall and no isthmus but vary largely in size. While some strains are smaller than 10 µm, others exceed 100 µm in length (compare Meso-1 and Meso-10 in ). Important diagnostic characters include the cell shape, chloroplast number in interphase, chloroplast morphology and position, pyrenoid morphology and position of the nucleus. Cell shapes can be roughly categorized as cylindrical (e.g., Serritaenia, Meso-1), elliptical (e.g., Meso-7) or elongate with rounded (e.g., Meso-6), conical (e.g., Meso-3, Meso-9) or truncate (e.g., Meso-2) cell ends. The chloroplasts range in number from one (Serritaenia, Meso-1 and Meso-2) to two (Meso-4, Meso-5 and Meso-7) and can occupy a parietal (e.g., Meso-2, Meso-6 and Meso-7) or axial position (e.g., Serritaenia, Meso-3 and Meso-8). True ribbon-like, axial chloroplasts as described for the type species of the genus Mesotaenium (M. endlicherianum Nägeli) have so far only been found in the lineages Meso-3 and Meso-9, suggesting that the genus Mesotaenium might belong to one of these lineages. However, currently, there is no strain that clearly matches the original description of M. endlicherianum, so that this important genus name cannot be applied with certainty. More common are lenticular (e.g., Meso-1 and Meso-7) and channel-like chloroplasts (Meso-2). Almost all lineages have chloroplasts with smooth margins, with the exception of Serritaenia (serrate/dentate margins) and Meso-2 (undulating margins). Pyrenoids are present in all lineages and vary in shape (circular or lentiform), size and conspicuousness. The nuclei of all studied strains are fairly inconspicuous but can be identified by the central spherical nucleolus. The position of the nucleus within the cell is of great diagnostic value. It is either central, i.e., on the longitudinal cell axis (often nested between the chloroplasts: Meso-5, Meso-6, Meso-7 and Meso-8), or displaced by the chloroplast (Serritaenia, Meso-1 and Meso2). It is important to note that some characters, especially chloroplast morphology, can vary with environmental conditions (e.g., Serritaenia species develop additional chloroplast ridges in laboratory culture; Busch & Hess, Citation2021) and that chloroplasts can be largely obscured by colourless globules (depending on the physiological state of the cells). Hence, cultures grown under defined conditions can have advantages in assessing the morphology of Mesotaenium-like zygnematophytes. This also applies to the chloroplast number, which doubles before cell division.
Figure 3. Morphological diversity of Mesotaenium-like zygnematophytes (differential interference contrast). (a) Strain GSM.5.thin (Serritaenia testaceovaginata). (b) Strain KH.2.sm (lineage Meso-1). (c) Strain SAG 12.97 sometimes referred to as “M. endlicherianum” (lineage Meso-2). (d) Strain E.1 (lineage Meso-3). (e) Strain GSM.2.16.s2 (lineage Meso-8). (f) Strain SAG 230–1 (lineage Meso-9). (g) Strain GSM.2.16.l (lineage Meso-5). (h) Strain SAG 1.88 (lineage Meso-4). (i) Strain LM.24 (lineage Meso-6). (j) Strain CCAC 2215 (lineage Meso-7). (k) Strain UTEX 1025 (lineage Meso-10). Scale bar in (a) is 10 µm and applies to all panels.
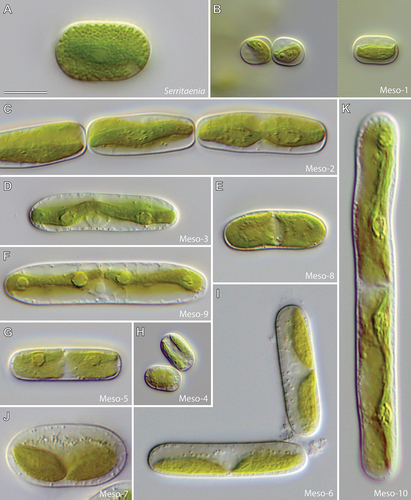
Mesotaenium-like zygnematophytes show also various “growth forms” in laboratory cultures, which probably result from differences in cell division and mucilage secretion. In liquid culture, cells can be solitary (); e.g., Meso-6, Meso-7), or form chains (); e.g., Meso-2, Meso-5), gelatinous films on submerged surfaces and/or discrete gelatinous “colonies” (); e.g., Serritaenia, Meso-1). Colony morphology and colour on solid media show marked differences as well. While some lineages form irregular gelatinous masses (e.g., Serritaenia), others produce flat, film-like plaques with even (); Meso-4) or erose (); Meso-3) margins or raised colonies with irregular surface (); Meso-1). All these observations on cell morphology and growth forms indicate profound biological variation in the 12 lineages of Mesotaenium-like zygnematophytes identified in this study. Short summaries of each of these lineages are provided in the following.
Figure 4. Growth forms of Mesotaenium-like zygnematophytes in culture. (a) Single cells in liquid culture, strain GSM.2.3.s (lineage Meso-7). (b) Cell chains in liquid culture, strain KM.19b (lineage Meso-5). (c) Colony-like mucilaginous aggregates in liquid culture, strain GSM.5.thin (Serritaenia testaceovaginata). (d) Shiny, smooth plaque with even margins on agar, strain SAG 1.88 (Meso-4) (e) Rough plaque with erose margins on agar, strain ACOI 127 (Meso-3). (f) Raised colonies with irregular surface on agar, strain KH.2.sm (Meso-1). Scale bars 100 µm in (a)–(c); 1 mm in (d) and (e); 5 mm in (f).
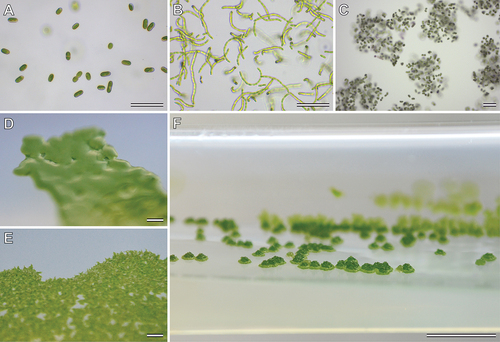
The Serritaenia lineage is the sister lineage of Meso-1 in our phylogeny with robust bootstrap support (99/99). The separation of the two lineages is based on clear-cut morphological differences and significant genetic distance (). The genus Serritaenia encompasses strains that were formerly associated with the species names Mesotaenium macrococcum, M. braunii and M. testaceovaginatum, but correct application of the two first names needs additional taxonomic research on difficult-to-obtain original material (for details see Busch & Hess, Citation2021). Interphase cells of Serritaenia species are mostly cylindrical with rounded cell ends ()), while cells shortly after division are ovoid and show a typical angled arrangement. Chain formation was never observed in Serritaenia. The single chloroplast is an axial plate with serrated or indented margins ()). The chloroplast can develop one or two additional ridges and thereby become more complex. In liquid laboratory cultures, Serritaenia cells can form irregular, gelatinous colonies ()) or confluent mucilage films. However, the mucilage can be dissolved under certain environmental conditions, and the cells become solitary. Serritaenia species are most prominent in terrestrial habitats and are known from central Europe, the United Kingdom, North America and South America. Some species can form mass developments in the form of gelatinous biofilms on various substrates (dead wood, leaf litter, soil, and bryophytes). Furthermore, Serritaenia represents the only known lineage of the Zygnematophyceae whose members produce an extracellular sunscreen pigment (Busch & Hess, Citation2021). Despite some (questionable) statements in the older literature, intracellular (pinkish) pigments have never been observed by the authors of this study (potentially these statements are based on confusion with lineage Meso-7).
Lineage Meso-1 contains two strains which have identical rbcL gene sequences (). The cells measure <15 µm in length and are cylindrical with rounded, rather flat cell ends. The plate-like or lenticular chloroplast with smooth margins is mostly parietal, rarely axial ()). Both strains were isolated from bryophytes on dead wood in temperate forests of Germany. In these habitats, cells of lineage Meso-1 are entirely embedded in mucilage (which can form tube-like structures) and can co-occur with Serritaenia species. The cells of lineage Meso-1 resemble Mesotaenium macrococcum var. lagerheimii Willi Krieg. to some extent (Krieger, Citation1933).
Lineage Meso-2 includes two strains characterized by elongated cells with flat, almost truncated cell ends ()). The single chloroplast can be plate-like but is frequently parietal with folded lateral sides, resulting in a channel-like morphology. The chloroplast bears undulate margins and rather prominent, lentiform pyrenoids. In culture, cells of lineage Meso-2 readily form chains through cell division and terminal mucilage secretion ()). Both strains were isolated from aquatic habitats, from freshwater plankton in Portugal (SAG 12.97) and from a brook in Spain (ACOI 2703). The genome-sequenced strain SAG 12.97 has been of interest (e.g., Bonnot et al., Citation2019; Cannell et al., Citation2020; Cheng et al., Citation2019; Donoghue & Paps, Citation2020; Wang et al., Citation2021) and was repeatedly referred to as Mesotaenium endlicherianum, but this designation is probably based on a misidentification as suggested by major morphological differences to the original description of that species (Nägeli, Citation1849).
Lineage Meso-3 comprises three strains, which match the description of Mesotaenium caldariorum (Lagerheim) Hansgirg (Hansgirg, Citation1886) and are listed under this name in public culture collections (see Supplementary table S1 for strain names). The cells are elongated with conical cell ends and contain an axial, ribbon-like chloroplast with clearly visible circular pyrenoids ()). In the top view, the chloroplast matches the shape of the cell (i.e., with tapered ends), while in the side view, it appears as a thin ribbon. The strains ACOI 127 and ACOI 898 were isolated from moist soil (Portugal) and flowing water (Madeira, Portugal), respectively. Strain E.1 derives from wet moss from a waterfall (Germany). Interestingly, the lineage Meso-3 is morphologically indistinct from lineage Meso-9 but shows a high genetic divergence (). Whether Meso-3 and Meso-9 are sister lineages remains to be solved by multigene phylogenies.
Lineage Meso-4 contains two strains, whose cells are cylindrical, sometimes slightly bent, with rounded cell ends ()). Each cell contains two parietal, plate-like chloroplasts situated at opposing lateral sides of the cell. Some cells can have four chloroplasts (before cell division). The strain SAG 1.88 derives from the Signy Islands (Antarctica) and was isolated from bare mineral soil (Broady, Citation1976). For the strain JH0031, no sampling information is available. Strain SAG 1.88 was previously assigned to the genus Fottea (Fottea pyrenoidosa; Broady, Citation1976), which, however, belongs to the chlorophytes (with its type species F. cylindrica Hindák). Hence, the species was transferred to the genus Mesotaenium (Petlovany, Citation2014) and is currently named Mesotaenium pyrenoidosum (P.A. Broady) Petlovany (Guiry & Guiry, Citation2021; Petlovany, Citation2014). However, the morphology of strain SAG 1.88 is certainly in conflict with that of the type species of Mesotaenium (M. endlicherianum).
The Ancylonema lineage contains three sequences, two of which are from the ice-dwelling species Ancylonema nordenskioeldii and Ancylonema alaskanum (here the epithet of the latter species is corrected in gender according to Articles 62.2 (c), 23.5 and 32.2; see Turland et al., Citation2018). The latter species was formerly known as Mesotaenium berggrenii (Procházková et al., Citation2021). For the third rbcL gene sequence, no strain information is available (). The cells are cylindrical or elongated with broadly rounded cell ends. They have one or two parietal, lenticular or cup-shaped chloroplasts with a circular pyrenoid and a central nucleus (Remias, Holzinger, & Lütz, Citation2009, Citation2012). As far as we know, there are no cultivated strains available, despite several attempts to cultivate these glacier algae (Remias et al., Citation2009, Citation2012) (see below for geographic information), where they can form blooms (Procházková et al., Citation2021; Remias et al., Citation2012; Williamson et al., Citation2020). Despite their close relationship, the two Ancylonema species differ drastically in cell size and shape. Cell chain formation is known in A. nordenskioeldii. Both A. nordenskioeldii and A. alaskanum contain red-brown vacuolar pigments, suspected sunscreen compounds (see below for details).
Lineage Meso-5 is closely related to the filamentous, terrestrial green alga Zygogonium ericetorum, with full bootstrap support (). The lineage comprises two strains, which cannot be assigned to an existing species with certainty, but show some similarities to the species Mesotaenium truncatum West & G.S.West (West & West, Citation1904). The cells are elongated with rather flat, almost truncated cell ends and contain a parietal plate-like chloroplast with a marked central indentation. This creates the impression of two chloroplasts, but there is a bridge between the two lobes. The nucleus is situated in the central region between the two chloroplast lobes ()). Both strains readily form cell chains and floating films at the air–water interface in liquid culture. They were isolated from moist and acidic terrestrial surfaces in Germany (strain KM.19b) and Tennessee, USA (strain GSM.2.16.l).
Lineage Meso-6 contains three strains, which are morphologically similar to Mesotaenium chlamydosporum De Bary (De Bary, Citation1858). The cells are elongated with broadly rounded cell ends and contain one or two shovel-like chloroplasts, which are always parietal ()). The cytoplasm often contains small, granular inclusions that can cover the nucleus and the chloroplast ()). The three strains derive from terrestrial samples (Germany).
Lineage Meso-7 contains six strains, which are morphologically similar to Mesotaenium mirificum W. Archer (Archer, Citation1864). Except for the elliptical shape, the cells are similar to those of lineage Meso-6 (compare )). The parietal, shovel-shaped chloroplasts and the nucleus are often surrounded by small granules ()). The strains were isolated from different freshwater and terrestrial habitats in Germany, Poland, the Great Smoky Mountains (USA), and Ontario, Canada (see Supplementary table S1 for details). Members of this lineage were found with copious mucilage in their natural habitats, which, however, is often lost in liquid culture. Strain GSM.5D.r was observed with pink cell content in its natural habitat (wet rocks, Great Smoky Mountains, Tennessee, USA).
Lineage Meso-8 contains two strains and has not been detected before (). The cells are cylindrical or slightly elongated with round cell ends ()). There are two parietal, shovel-shaped chloroplasts per cell, and the cytoplasm contains numerous small granules, often surrounding the nucleus in the form of a chain. Both strains were isolated from black-greenish mucilage on soil in the Great Smoky Mountains (Tennessee, USA).
Lineage Meso-9 is represented by three strains, which have identical rbcL gene sequences (). The strains are morphologically similar to Mesotaenium caldariorum (Lagerheim) (Hansgirg, Citation1886) and are also named as such in culture collections and in published phylogenies (e.g., Surek, Beemelmanns, Melkonian, & Bhattacharya, Citation1994). As mentioned above, lineage Meso-9 is morphologically indistinct from lineage Meso-3 (compare )) but shows high genetic divergence (). The cells are elongated with conical cell ends and contain a central ribbon-like chloroplast with clearly visible, circular pyrenoids ()). The strains were isolated from moist terrestrial surfaces, a boggy meadow in Austria (SAG 150.80) and a wet brick in the Czech Republic (SAG 648.1). For SAG 230–1, no sampling information is available. Furthermore, the plastid movement and phytochromes of the strain SAG 648–1 (=UTEX 41) were studied intensively (see below for details).
Lineage Meso-10 is represented by one strain (UTEX 1025), which is morphologically similar to Mesotaenium kramstai (Lemmermann, Citation1896) and also named as such in culture collections as well as in published phylogenies (e.g., Gontcharov et al., Citation2003). The cells are long, rod-shaped with rounded cell ends, and the chloroplast is a ribbon with folded margins ()). The chloroplast is, however, quite variable in shape. The strain derives from Texas (USA) from “air”. It is the largest known zygnematophyte associated with the genus Mesotaenium.
The updated rbcL phylogeny reveals massive polyphyly of the genus Mesotaenium and, furthermore, reveals a so far hidden diversity of small, unicellular zygnematophytes. Probably, there are still many more new lineages to discover. As we show here, Mesotaenium-like algae are morphologically diverse, and specific cellular details can be used to distinguish some but not all lineages of these algae.
Distribution and ecology of Mesotaenium-like zygnematophytes
Mesotaenium-like zygnematophytes appear to be distributed over the whole globe as indicated by accounts from all over Europe, Africa, North and South America, Australia, certain areas in Asia, as well as the Arctic and Antarctic (e.g., De Bary, Citation1858; Hansgirg, Citation1886; Krieger, Citation1937; Ling & Seppelt, Citation1990; Remias et al., Citation2012; West & West, Citation1904). They inhabit various aquatic and terrestrial habitat types, including extreme habitats such as glacial ice (details below). Hence, different Mesotaenium-like zygnematophytes appear to vary strongly in their ecology. The phylogenetic tree in gives a rough overview of the habitat preferences of the 12 lineages defined in this study. The strains labelled as “aerophytic” were isolated from terrestrial surfaces that are subjected to periodic desiccation, while those associated with the term “submerged” come from flowing or standing waters that ensure permanent supply of water. All except two of the 12 lineages contain aerophytic strains, with seven lineages exclusively known from terrestrial habitats. Hence, a “life on land” appears to be very common among Mesotaenium-like zygnematophytes.
The habitats of representatives with a submerged lifestyle such as strains of the lineages Meso-2 and Meso-3 include puddles, boggy pools, brooks and streamlets (Gontcharov et al., Citation2004; West & West, Citation1904). Strain E.1 of lineage Meso-3 was isolated from wet moss in a waterfall, which is also considered a submerged habitat ()). Blooms of suspended cells seem to be rare, but in certain cases, Mesotaenium-like zygnematophytes can form a skin on the water surface as reported for an aquatic species determined as M. endlicherianum (Hansgirg, Citation1886).
Figure 5. Habitats and biotic interactions of Mesotaenium-like zygnematophytes. (a) Waterfall with wet moss cushions (near Nohn, Eifel, Germany) inhabited by a species of lineage Meso-3. (b) Tree trunk with black algal crust (nature reserve “Heiliges Meer”, Recke, Germany). (c) Decaying dead wood with green, mucilaginous layer (nature reserve “Schwarzes Wasser”, Wesel, Germany). (d) Rock surface of “wet walls” colonized by various microalgae, including species of Serritaenia and Meso-7 (Great Smoky Mountains, North Carolina, USA). (e) Molinia caerulea (purple moor grass) in a Molinia-dominated degeneration phase of a heathland is heavily colonized by Serritaenia species (nature reserve “Heiliges Meer”, Recke, Germany). (f) Undetermined pleurocarpous moss almost entirely covered by a black crust (Wiehl, Germany). (g) Same moss plant as shown in (f) after rehydration in water. The moss is heavily colonized by a Serritaenia species that forms globular colonies. (h) Araucarian forest (Nahuelbuta National Park, Chile) with rocks colonized by a potentially chasmoendolithic Serritania species (courtesy of T. Darienko). (i) Glacial ice inhabited by Ancylonema species (Morteratsch Glacier, Switzerland; courtesy of D. Remias). (j) Vampyrellid amoeba extracting the content of a Serritaenia species shown during feeding (brightfield; courtesy of H. Schulp). (k) Digestive cyst of the same vampyrellid species shown in (j) next to two perforated and emptied algal cells (brightfield; courtesy of H. Schulp). (l) Gelatinous colony of Serritaenia testaceovaginata with (fungal?) hyphae (differential interference contrast). (m) Cell of Serritaenia testaceovaginata from the population shown in (l) penetrated by the filamentous, fungus-like parasite (differential interference contrast). Scale bars 10 µm in (l) and (m), not available for (j) and (k).
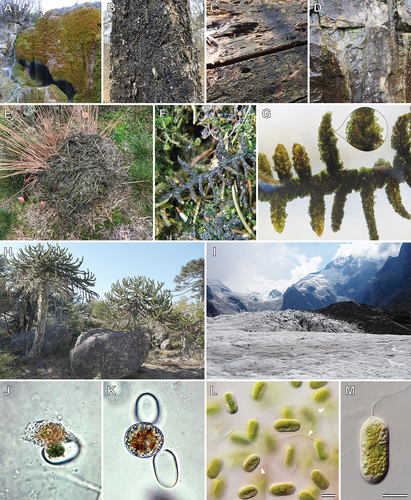
The aerophytic strains of lineages Meso-1, Meso-5, Meso-7, Meso-8 and Serritaenia from temperate zones colonize tree bark ()), dead wood ()), rock surfaces ()), soil and bryophytes. There are also accounts of Mesotaenium-like zygnematophytes on damp soil in heathers and warm houses (Hansgirg, Citation1886; West & West, Citation1901), and it seems that most species occur on acidic substrates. They are often part of multi-species communities, e.g., intermingled with chlorophyte green algae, cyanobacteria or moss protonema. However, Serritaenia species and the members of lineage Meso-7 can also form uni-algal, gelatinous patches that are visible with the naked eye (Busch & Hess, Citation2021; De Bary, Citation1858; West & West, Citation1904). In some instances, we observed mass developments of Serritaenia species that covered relatively large areas in moist German spruce forests and Molinia-dominated heathland () and Busch & Hess, Citation2021). In these areas, Serritaenia can overgrow entire bryophytes (e.g., various acrocarpous and pleurocarpous mosses) with severe effects on the plants ()). Similar mass developments were reported for M. chlamydosporum and M. mirificum (Hansgirg, Citation1886; West & West, Citation1901), which might belong to the lineages Meso-6 and Meso-7, respectively. Furthermore, one Serritaenia strain (not yet sequenced) was isolated from a rock in an Araucaria forest in the Nahuelbuta National Park, Chile ()). According to the collectors, the algal material was extracted from cracks in the rock, suggesting a chasmoendolithic lifestyle (pers. comm. Tatyana Darienko).
Mesotaenium-like zygnematophytes also colonize extreme habitats and are especially well known from glacial ice and snow in alpine or polar regions (e.g., Procházková et al., Citation2021; Yallop et al., Citation2012). The two cryophilic algal species Ancylonema nordenskioeldii and Ancylonema alaskanum (formerly known as Mesotaenium breggrenii) were found in the Arctic (e.g., Harding, Jungblut, Lovejoy, & Vincent, Citation2011; Uetake, Naganuma, Hebsgaard, Kanda, & Kohshima, Citation2010; Williamson et al., Citation2020), Alaska (Takeuchi, Citation2001), the Antarctic (Izaguirre & Pizarro, Citation1997; Ling & Seppelt, Citation1990), on a Himalayan glacier (Yoshimura, Kohshima, & Ohtani, Citation1997), a Chilean glacier (Takeuchi & Kohshima, Citation2004) and in the European Alps (Procházková et al., Citation2021). In these habitats, Ancylonema species can bloom in the ice, which results in large, grey or brown areas ()). This darkening caused by a reddish-brown, intracellular pigments (details below) leads to increased absorption of solar radiation and melting rates of glacial ice (Takeuchi, Kohshima, & Seko, Citation2001; Williamson et al., Citation2018, Citation2020).
Despite their broad distribution and ecological versatility, Mesotaenium-like zygnematophytes are poorly captured by environmental sequence data, and most of the known diversity stems from culture-based approaches. Large environmental sequencing studies have been conducted in the open oceans and the pelagic zone of lakes (e.g., Boenigk et al., Citation2018; de Vargas et al., Citation2015), which are typically not very rich in zygnematophytes. Studies on terrestrial samples and microbial communities on trees often focus on bacteria, fungi and heterotrophic protists (e.g., Heger et al., Citation2018; Nacke et al., Citation2011; O’Brien, Parrent, Jackson, Moncalvo, & Vilgalys, Citation2005; Walden et al., Citation2021). There are, however, several environmental sequencing studies on biological soil crusts in dry polar regions and on glacial ice, which detected zygnematophycean amplicons, including those of the cryophilic Ancylonema nordenskioeldii (Büdel, Dulić, Darienko, Rybalka, & Friedl, Citation2016; Garcés-Pastor et al., Citation2019; Lutz, Anesio, Edwards, & Benning, Citation2017; Rippin, Citation2018; Rippin, Lange, Sausen, & Becker, Citation2018; Samolov et al., Citation2020). The typical habitats of mesophilic saccoderm desmids, i.e., various surfaces in temperate forests, heathers and moorlands, remain undersampled. Based on our microscopic observations and the historical literature, we expect that environmental sequencing directed towards algal biofilms in temperate zones will be a useful method to further expand our phylogenetic knowledge about unicellular zygnematophytes and reveal more of their ecological preferences.
Furthermore, there are observations that point to interesting biotic interactions between Mesotaenium-like zygnematophytes and other microbes. An unidentified, potentially undescribed vampyrellid amoeba (Vampyrellida, Rhizaria) was documented to extract the cellular contents of Serritaenia cells in a terrestrial sample ()). These “protoplast feeders” often have a narrow prey range (Hess & Suthaus, Citation2022), and the undersampled microhabitats mentioned above might contain their own heterotrophic microfauna. In addition, we repeatedly observed (fungal?) hyphae running through the gelatinous colonies of Serritaenia species from different geographic localities in Europe and North America (), arrowheads). These potentially biotrophic parasites penetrate algal cells and eventually lead to cell death ()). So far, we know almost nothing about these heterotrophic associates of terrestrial microalgae, but our first attempts to isolate them promise to change this situation.
Cellular adaptations of Mesotaenium-like zygnematophytes to environmental factors
As shown above, various Mesotaenium-like zygnematophytes have a terrestrial lifestyle (). Hence, they face strong fluctuations in water availability and temperature and increased exposure to sunlight. Mesotaenium-like zygnematophytes certainly have cellular adaptations to these abiotic stressors, for instance, the excessive mucilage secretion observed in Serritaenia and lineage Meso-7 that probably results in an increased water-holding capacity and extended photosynthetic periods. However, the cellular reactions of Mesotaenium-like zygnematophytes to desiccation are unstudied, in contrast to those of the filamentous forms. We know that Zygnema and Zygogonium accumulate osmolytes (sucrose), upregulate aquaporins and stress-related molecules (early light-inducible proteins, chaperones, late embryogenesis abundant proteins and DNA repair proteins), and they alter the thickness and composition of their cell walls (de Vries et al., Citation2018; Fuller, Citation2013; Herburger & Holzinger, Citation2015; Herburger, Remias, Holzinger, & Elster, Citation2016; Herburger, Xin, & Holzinger, Citation2019; Holzinger, Tschaikner, & Remias, Citation2010; Rippin, Becker, & Holzinger, Citation2017; Sørensen et al., Citation2011). In natural material of Mesotaenium-like zygnematophytes, one can observe pronounced cellular changes as well, which deserve future analysis.
Three remarkable photoprotective strategies have been studied in Mesotaenium-like zygnematophytes: (i) rotary chloroplast movement, (ii) vacuolar pigmentation and (iii) sunscreen mucilage. As shown in our phylogeny, these strategies are not necessarily limited to a single lineage but can also be found in other, even filamentous members (). Rotary chloroplast movement was intensely studied in strain SAG 648–1 (referred to as Mesotaenium caldariorum) of lineage Meso-9 and in the filamentous zygnematophyte Mougeotia scalaris, both of which have a similar, ribbon-like chloroplast morphology (Haupt & Scheuerlein, Citation1990; Wada, Grolig, & Haupt, Citation1993). Depending on the light conditions, these algae re-arrange the chloroplast within the cell by axial rotation and alter the chloroplast surface that faces the light source between maximum (chloroplast in top view) and minimum surface (chloroplast in profile view). In both algae, an interplay of two photosensory receptors, phytochromes and cryptochromes, was found to trigger this photo-orientation of the chloroplast, and it has been hypothesized that the movement is mediated by the actin cytoskeleton (Haupt, Citation1991; Klein, Wagner, & Blatt, Citation1980; Kraml, Büttner, Haupt, & Herrmann, Citation1988; Wagner, Haupt, & Laux, Citation1972). However, research on chloroplast rotation in unicellular zygnematophytes ceased more than 20 years ago, and we suspect that there is much more to explore. Relatively recently, time-lapse microscopy of Spirogloea muscicola revealed an oscillating rotation of its helical chloroplast (Cheng et al., Citation2019), but it is yet unknown whether this relates to photoprotection (e.g., through rotational self-shading of the chloroplast).
The two cryophilic species of the Ancylonema lineage are well known for their intense, intracellular pigmentation (Remias, Holzinger, Aigner, & Lütz, Citation2012; Remias et al., Citation2009). The water-soluble, red-brown pigments are localized in one or more vacuoles of the cell and can largely obscure other cellular details ()). The pigment of A. alaskanum was isolated from natural material and identified as a glycosylated purpurogallin derivative by nuclear magnetic resonance spectroscopy (Remias et al., Citation2012). The isolated substance was shown to absorb both ultraviolet radiation and visible light, suggesting a function as an intracellular sunscreen (Remias et al., Citation2012). Another study corroborated the strong UV absorbance of the abundant Ancylonema pigments and revealed their pronounced effect on overall cellular energy absorption (increased 50-fold by phenolic pigments; Williamson et al., Citation2020). This enables Ancylonema species to withstand the extreme solar irradiance present in glacier environments, while the chloroplasts are still low-light adapted (Williamson et al., Citation2020). The absorbed short-wavelength radiation is converted to heat and leads to melting of the glacier ice. This has severe impact on the integrity of the Greenland Ice Sheet, which experiences blooms of Anyclonema species (Williamson et al., Citation2018, Citation2020; Yallop et al., Citation2012). However, vacuolar pigments are not limited to the cryophilic species of the Ancylonema lineage but have been observed in several other zygnematophytes that colonize habitats of increased solar radiation (e.g., sun-exposed dead wood, ground in heathlands and shallow high mountain lakes). This includes members of lineage Meso-7 ()), Cylindrocystis brebissonii f. cryophila (no genetic information available) and the filamentous zygnematophytes Zygogonium ericetorum ()) and Temnogametum iztacalense ()) (Aigner, Remias, Karsten, Holzinger, & Bassi, Citation2013; Garduño-Solórzano et al., Citation2021; Nedbalová & Sklenár, Citation2008). Hence, the production of intracellular pigments occurs in several distinct lineages of the Zygnematophyceae (), and differences in colour (red-brown vs. purple) indicate variations in chemical structure and physical properties. In fact, the purple pigment of Zygogonium ericetorum might be a highly branched polymer of glucose with traces of ester-linked polyphenolic moieties (e.g., gallic acid) that form a colourful complex with ferric iron (Newsome & van Breemen, Citation2012). In addition, Holzinger et al. (Citation2010) detected two water-soluble compounds that mainly absorb UV light, suggesting that the chemical photoprotection of zygnematophytes can be composed of several compounds.
Figure 6. Non-photosynthetic pigments of Mesotaenium-like algae and other zygnematophytes. (a) Ancylonema alaskanum with dark-red vacuolar contents (Morteratsch Glacier, Switzerland; courtesy of D. Remias). (b) Ancylonema nordenskioeldii with red cellular contents (Morteratsch Glacier, Switzerland; micrograph from Procházková et al., Citation2021). (c) Cells of lineage Meso-7 with rose cellular contents (Wet walls, Great Smoky Mountains, North Carolina, USA). (d) Zygogonium ericetorum with pink vacuolar contents (Streamlet, Schoenwieskopf, Tyrol, Austria; micrograph from Aigner et al., Citation2013). (e) Temnogametum iztacalense with purple vacuolar contents (Lake La Luna, Nevado de Toluca, Mexico; courtesy of G. Garduño Solorzano). (f) Serritaenia sp. with purple-blue pigment in extracellular mucilage (from moss shown in )). Scale bars 10 µm, scale information for (c) not available.
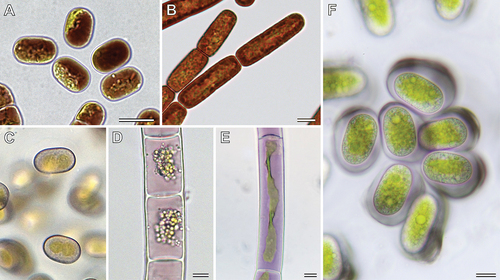
Another sunscreen strategy, which appears to be unique among zygnematophytes, is found in the genus Serritaenia. These algae produce mucilaginous capsules with an extracellular, insoluble pigment that may function as external sunscreen ()). The pigmented mucilage occupies a perfect position to shade the entire cell and was shown to effectively absorb over the UV–vis range, with a peak at about 300 nm (Busch & Hess, Citation2021). The synthesis of Serritaenia´s sunscreen mucilage is not constitutive and can be specifically induced by UVB radiation under laboratory conditions. The colouration of the mucilage varies in nature (bright red, brown, black and blue), which is (partially) influenced by the pH value of the surrounding medium. All Serritaenia species tested so far produce blueish mucilage under our culture conditions. The chemical structure of the pigment involved is as yet unknown.
All three photoprotective strategies found in the zygnematophytes (chloroplast movements, intracellular and extracellular pigments) provide interesting analogies to phenomena known from land plants, e.g., chloroplast avoidance movement (Kasahara et al., Citation2002), vacuolar anthocyanins (Steyn, Wand, Holcroft, & Jacobs, Citation2002) and cell walls with potentially photoprotective pigments (Hooijmaijers & Gould, Citation2007; Mårtensson & Nilsson, Citation1974). Yet, most of the cellular and biochemical background of such adaptations in zygnematophytes is still unresolved, and the unicellular Mesotaenium-like zygnematophytes might be useful laboratory models for future research.
Perspectives for future research
The available cultures of diverse Mesotaenium-like zygnematophytes enable various experimental studies. Ecophysiological comparisons concerning light preferences, freezing and drought tolerance may provide explanations for the unexpected diversity of these otherwise inconspicuous algae. A very obvious difference between the lineages is the varying propensity to form extracellular mucilage and the varying properties of the latter (e.g., confluent, capsular, hierarchical layers and terminal mucilage pillows). The mucilaginous, extracellular polymers of zygnematophytes have mainly been studied in Penium, Micrasterias, Netrium and several filamentous forms (e.g., Domozych, Citation2014; Eder & Lütz-Meindl, Citation2010; Eder & Lütz‐Meindl, Citation2008; Palacio-López, Tinaz, Holzinger, & Domozych, Citation2019; Pfeifer et al., Citation2021), leaving the exopolymers of unicellular, terrestrial zygnematophytes biochemically unexplored. The sophisticated experiments performed on the studied zygnematophytes already revealed how complex the extracellular polymers of these algae are, containing pectic substances, hemicelluloses, arabinogalactan-proteins and rhamnogalactan proteins (Domozych et al., Citation2014; Palacio-López, Tinaz, Holzinger, & Domozych, Citation2019; Pfeifer et al., Citation2021; Sørensen et al., Citation2011 and references therein). It would certainly be very interesting to extend such studies to some terrestrial members of the Mesotaenium-like algae.
Despite the numerous phylogenetic studies performed on zygnematophytes, the deeper branches of this algal class are still unresolved (e.g., Gontcharov, Citation2008; Hall, Karol, McCourt, & Delwiche, Citation2008). In addition, we demonstrated that with manageable effort, one can find zygnematophycean lineages that have not been detected before. To better understand the evolution of the zygnematophytes, we need increased sampling and cultivation (including cells that look similar to known strains/species) as well as genomic or transcriptomic data from all relevant lineages. The latter can be used for multigene phylogenies, which, hopefully, will better resolve the branching pattern of major zygnematophycean clades in the future. At the moment, such data from Mesotaenium-like zygnematophytes are still scarce, limited to four out of 12 lineages (Serritaenia, Meso-2, Meso-9 and Meso-10; see stars in ). However, next-generation sequencing data from additional lineages are in progress.
The in-depth analysis of genomic and transcriptomic data also provides information on molecular innovations that might relate to major evolutionary transitions. The genomic analysis of strain SAG 12.97 (lineage Meso-2), for example, revealed expanded orthogroups that likely evolved in the common ancestor of the zygnematophytes and land plants. This includes genes for transcription factors, phytohormone signalling and genes involved in the biosynthesis and remodelling of the cell wall (Cheng et al., Citation2019). An interesting gene expansion was discovered for the GRAS transcription factor family, with a potential origin from soil bacteria by horizontal gene transfer (Cheng et al., Citation2019). Another interesting example is small RNA pathway-related genes that possibly contribute to pathogen defence and antibacterial immunity, a topic poorly explored in unicellular algae (Wang et al., Citation2021). To really understand the evolution of such mechanisms, dense genomic sampling at a phylogenetic level will be essential. Hence, biodiversity exploration is crucial, including inconspicuous life forms such as desmids of the Mesotaenium-type.
Supplemental Material
Download MS Word (20.4 KB)Acknowledgments
We thank Barbara and Michael Melkonian (formerly Culture Collection of Algae at the University of Cologne, CCAC), Maike Lorenz (Culture Collection of Algae at Göttingen University, SAG), Mariana Assunção and Lilia Santos (Coimbra Culture Collection of Algae, ACOI) and Karl-Heinz Linne von Berg (University of Cologne) for providing algal cultures. Gloria Garduño Solorzano (National Autonomous University of Mexico), Daniel Remias (University of Applied Sciences Upper Austria), Andreas Holzinger (Innsbruck University) and Tatyana Darienko (Göttingen University) kindly provided micrographs and information on algal isolates. Furthermore, we acknowledge the permissions to sample in the Great Smoky Mountains National Park (USA; permit number GRSM-2017-SCI-2168) and the nature reserve “Heiliges Meer” of the LWL-Museum of Natural History (Recke, Germany) very much. This work was funded by the German Research Foundation, grants 417585753 (Emmy Noether Programme) and 491244984 (both to S.H.).
Disclosure statement
No potential conflict of interest was reported by the author(s).
Supplementary material
Supplemental data for this article can be accessed online at https://doi.org/10.1080/26388081.2022.2081819
Additional information
Funding
References
- Aigner, S., Remias, D., Karsten, U., Holzinger, A., & Bassi, R. (2013). Unusual phenolic compounds contribute to ecophysiological performance in the purple-colored green alga Zygogonium ericetorum (Zygnematophyceae, Streptophyta) from a high-alpine habitat. Journal of Phycology, 49, 648–660.
- Archer, W. (1864). Original communications: An endeavour to identify Palmogloea macrococca (Kütz.) with description of the plant believed to be meant, and of a new species, both, however, referable rather to the genus Mesotaenium (Näg.). Journal of Cell Science, 2, 109–132.
- Bass, D., & Cavalier-Smith, T. (2004). Phylum-specific environmental DNA analysis reveals remarkably high global biodiversity of Cercozoa (Protozoa). International Journal of Systematic and Evolutionary Microbiology, 54, 2393–2404.
- Bass, D., Chao, E. E.-Y., Nikolaev, S., Yabuki, A., Ishida, K., Berney, C., … Cavalier-Smith, T. (2009). Phylogeny of novel naked filose and reticulose Cercozoa: Granofilosea cl. n. and Proteomyxidea revised. Protist, 160, 75–109.
- Boenigk, J., Wodniok, S., Bock, C., Beisser, D., Hempel, C., Grossmann, L., … Jensen, M. (2018). Geographic distance and mountain ranges structure freshwater protist communities on a European scalе. Metabarcoding and Metagenomics, 2, e21519.
- Bonnot, C., Hetherington, A. J., Champion, C., Breuninger, H., Kelly, S., & Dolan, L. (2019). Neofunctionalisation of basic helix−loop−helix proteins occurred when embryophytes colonised the land. New Phytologist, 223, 993–1008.
- Broady, P. A. (1976). Six new species of terrestrial algae from Signy Island, South Orkney Islands, Antarctica. British Phycological Journal, 11, 387–405.
- Brook, A. J., & Williamson, D. B. (2010). A monograph on some British desmids. London: Ray Society.
- Büdel, B., Dulić, T., Darienko, T., Rybalka, N., & Friedl, T. (2016). Cyanobacteria and algae of biological soil crusts. In B. Weber, B. Büdel, & J. Belnap (Eds.), Biological soil crusts: An organizing principle in drylands (Vol. 226, pp. 55–80). Basel: Springer International Publishing.
- Busch, A., & Hess, S. (2021). Sunscreen mucilage: A photoprotective adaptation found in terrestrial green algae (Zygnematophyceae). European Journal of Phycology, 1–18.
- Cannell, N., Emms, D. M., Hetherington, A. J., MacKay, J., Kelly, S., Dolan, L., & Sweetlove, L. J. (2020). Multiple metabolic innovations and losses are associated with major transitions in land plant evolution. Current Biology, 30, 1783–1800.e11.
- Cheng, S., Xian, W., Fu, Y., Marin, B., Keller, J., Wu, T., … Melkonian, M. (2019). Genomes of subaerial Zygnematophyceae provide insights into land plant evolution. Cell, 179, 1057–1067.e14.
- Coesel, P. F. M., & Meesters, K. J. (2007). Desmids of the Lowlands: Mesotaeniaceae and Desmidiaceae of the European Lowlands. Leiden: BRILL.
- De Bary, A. (1858). Untersuchungen über die Familie der Conjugaten: 91 pp. Leipzig.
- de Vargas, C., Audic, S., Henry, N., Decelle, J., Mahé, F., Logares, R., … Velayoudon, D., et al. (2015). Eukaryotic plankton diversity in the sunlit ocean. Science, 348, 1261605.
- de Vries, J., de Vries, S., Slamovits, C. H., Rose, L. E., & Archibald, J. M. (2017). How embryophytic is the biosynthesis of phenylpropanoids and their derivatives in streptophyte algae? Plant & Cell Physiology, 58, 934–945.
- de Vries, J., Curtis, B. A., Gould, S. B., & Archibald, J. M. (2018). Embryophyte stress signaling evolved in the algal progenitors of land plants. Proceedings of the National Academy of Sciences, 115, E3471–E3480.
- de Vries, J., de Vries, S., Curtis, B. A., Zhou, H., Penny, S., Feussner, K., … Archibald, J. M. (2020). Heat stress response in the closest algal relatives of land plants reveals conserved stress signaling circuits. The Plant Journal, 103, 1025–1048.
- Delaux, P.-M., Nanda, A. K., Mathé, C., Sejalon-Delmas, N., & Dunand, C. (2012). Molecular and biochemical aspects of plant terrestrialization. Perspectives in Plant Ecology, Evolution and Systematics, 14, 49–59.
- Domozych, D. S., Sørensen, I., Popper, Z. A., Ochs, J., Andreas, A., Fangel, J. U., … Willats, W. G. (2014). Pectin metabolism and assembly in the cell wall of the charophyte green alga Penium margaritaceum. Plant Physiology, 165, 105–118.
- Domozych, D. S. (2014). Penium margaritaceum: A unicellular model organism for studying plant cell wall architecture and dynamics. Plants, 3, 543–558.
- Donoghue, P., & Paps, J. (2020). Plant evolution: Assembling land plants. Current Biology, 30, R81–R83.
- Eder, M., & Lütz‐Meindl, U. (2008). Pectin‐like carbohydrates in the green alga Micrasterias characterized by cytochemical analysis and energy filtering TEM. Journal of Microscopy, 231, 201–214.
- Eder, M., & Lütz-Meindl, U. (2010). Analyses and localization of pectin-like carbohydrates in cell wall and mucilage of the green alga Netrium digitus. Protoplasma, 243, 25–38.
- Ettl, H., & and Gärtner, G. (2014). Syllabus der Boden-, Luft- und Flechtenalgen. Berlin Heidelberg: Springer Spektrum.
- Fučíková, K., Hall, J. D., Johansen, J. R., & Lowe, R. (2008). Desmid flora of the Great Smoky Mountains National Park, USA. Bibliotheca Phycologica, 113, 1–59.
- Fuller, C. L. (2013). Examining morphological and physiological changes in Zygnema irregulare during a desiccation and recovery period (PhD Thesis).
- Galtier, N., Gouy, M., & Gautier, C. (1996). Seaview and phylo_win: Two graphic tools for sequence alignment and molecular phylogeny. Bioinformatics, 12, 543–548.
- Garcés-Pastor, S., Wangensteen, O. S., Pérez-Haase, A., Pèlachs, A., Pérez-Obiol, R., Cañellas-Boltà, N., … Vegas-Vilarrúbia, T. (2019). DNA metabarcoding reveals modern and past eukaryotic communities in a high-mountain peat bog system. Journal of Paleolimnology, 62, 425–441.
- Garduño-Solórzano, G., Martínez-García, M., Scotta Hentschke, G., Lopes, G., Castelo Branco, R., Vasconcelos, V. M. O., … Quintanar-Zúñiga, R. E. (2021). The phylogenetic placement of Temnogametum (Zygnemataceae) and description of Temnogametum iztacalense sp. nov., from a tropical high mountain lake in Mexico. European Journal of Phycology, 56, 159–173.
- Gerrath, J. F. (1993). The biology of desmids: A decade of progress. Progress in Phycological Research, 9, 79–192.
- Gerrienne, P., Servais, T., & Vecoli, M. (2016). Plant evolution and terrestrialization during Palaeozoic times—The phylogenetic context. Review of Palaeobotany and Palynology, 227, 4–18.
- Gontcharov, A. A., Marin, B., & Melkonian, M. (2003). Molecular phylogeny of conjugating green algae (Zygnemophyceae, Streptophyta) inferred from SSU rDNA sequence comparisons. Journal of Molecular Evolution, 56, 89–104.
- Gontcharov, A. A., Marin, B., & Melkonian, M. (2004). Are combined analyses better than single gene phylogenies? A case study using SSU rDNA and rbcL sequence comparisons in the Zygnematophyceae (Streptophyta). Molecular Biology and Evolution, 21, 612–624.
- Gontcharov, A. A. (2008). Phylogeny and classification of Zygnematophyceae (Streptophyta): Current state of affairs. Fottea, 8, 87–104.
- Gontcharov, A. A., & Melkonian, M. (2010). Molecular phylogeny and revision of the genus Netrium (Zygnematophyceae, Streptophyta): Nucleotaenium gen. nov. Journal of Phycology, 46, 346–362.
- Gouy, M., Guindon, S., & Gascuel, O. (2010). SeaView Version 4: A multiplatform graphical user interface for sequence alignment and phylogenetic tree building. Molecular Biology and Evolution, 27, 221–224.
- Guillou, L., Viprey, M., Chambouvet, A., Welsh, R. M., Kirkham, A. R., Massana, R., … Worden, A. Z. (2008). Widespread occurrence and genetic diversity of marine parasitoids belonging to Syndiniales (Alveolata). Environmental Microbiology, 10, 3349–3365.
- Guiry, M. D. (2013). Taxonomy and nomenclature of the Conjugatophyceae (= Zygnematophyceae). ALGAE, 28, 1–29.
- Guiry, M. D., & Guiry, G. M. (2021, December 21). AlgaeBase. World-wide electronic publication, Galway: National University of Ireland. https://www.algaebase.org
- Hainz, R., Wöber, C., & Schagerl, M. (2009). The relationship between Spirogyra (Zygnematophyceae, Streptophyta) filament type groups and environmental conditions in Central Europe. Aquatic Botany, 91, 173–180.
- Hall, J. D., Karol, K. G., McCourt, R. M., & Delwiche, C. F. (2008). Phylogeny of the conjugating green algae based on chloroplast and mitochondrial nucleotide sequence data (1). Journal of Phycology, 44, 467–477.
- Hall, J. D., & McCourt, R. M. (2015). Chapter 9 - Conjugating green algae including desmids. In J. D. Wehr, R. G. Sheath, & J. P. Kociolek (Eds.), Freshwater Algae of North America (Second Edition) (pp. 429–457). San Diego: Academic Press. doi:10.1016/B978-0-12-385876-4.00009-8
- Hansgirg, A. (1886). Prodromus der Algenflora von Böhmen. 1. Theil [Teil]. Enthaltend die Rhodophyceen, Phaeophyceen und einen Teil der Chlorophyceen. Řivnáč.
- Harding, T., Jungblut, A. D., Lovejoy, C., & Vincent, W. F. (2011). Microbes in high Arctic snow and implications for the cold biosphere. Applied and Environmental Microbiology, 77, 3234–3243.
- Haupt, W., & Scheuerlein, R. (1990). Chloroplast movement. Plant, Cell & Environment, 13, 595–614.
- Haupt, W. (1991). Phytochrome and cryptochrome: Coaction or interaction in the control of chloroplast orientation. In Riklis E.(ed.), Photobiology (pp. 479–489). New York: Springer.
- Heger, T. J., Giesbrecht, I. J. W., Gustavsen, J., Del Campo, J., Kellogg, C. T. E., Hoffman, K. M., … Keeling, P. J. (2018). High-throughput environmental sequencing reveals high diversity of litter and moss associated protist communities along a gradient of drainage and tree productivity. Environmental Microbiology, 20, 1185–1203.
- Herburger, K., & Holzinger, A. (2015). Localization and quantification of callose in the streptophyte green algae Zygnema and Klebsormidium: Correlation with desiccation tolerance. Plant & Cell Physiology, 56, 2259–2270.
- Herburger, K., Remias, D., Holzinger, A., & Elster, J. (2016). The green alga Zygogonium ericetorum (Zygnematophyceae, Charophyta) shows high iron and aluminium tolerance: Protection mechanisms and photosynthetic performance. FEMS Microbiology Ecology, 92, fiw103.
- Herburger, K., Xin, A., & Holzinger, A. (2019). Homogalacturonan accumulation in cell walls of the green alga Zygnema sp. (Charophyta) increases desiccation resistance. Frontiers in Plant Science, 10, 540.
- Hess, S., & Suthaus, A. (2022). The vampyrellid amoebae (Vampyrellida, Rhizaria). Protist, 173, 1–27. doi:10.1016/j.protis.2021.125854
- Holzinger, A., Tschaikner, A., & Remias, D. (2010). Cytoarchitecture of the desiccation-tolerant green alga Zygogonium ericetorum. Protoplasma, 243, 15–24.
- Holzinger, A., Kaplan, F., Blaas, K., Zechmann, B., Komsic-Buchmann, K., Becker, B., & Araujo, W. L. (2014). Transcriptomics of desiccation tolerance in the streptophyte green alga Klebsormidium reveal a land plant-like defense reaction. PLOS ONE, 9, e110630.
- Holzinger, A., & Pichrtová, M. (2016). Abiotic stress tolerance of charophyte green algae: New challenges for omics techniques. Frontiers in Plant Science, 7, 678.
- Hooijmaijers, C. A. M., & Gould, K. S. (2007). Photoprotective pigments in red and green gametophytes of two New Zealand liverworts. New Zealand Journal of Botany, 45, 451–461.
- Izaguirre, I., & Pizarro, H. (1997). Epilithic algae in a glacial stream at Hope Bay (Antarctica). Polar Biology, 19, 24–31.
- Kasahara, M., Kagawa, T., Oikawa, K., Suetsugu, N., Miyao, M., & Wada, M. (2002). Chloroplast avoidance movement reduces photodamage in plants. Nature, 420, 829–832.
- Kawai, J., Kanazawa, M., Suzuki, R., Kikuchi, N., Hayakawa, Y., & Sekimoto, H. (2022). Highly efficient transformation of the model zygnematophycean alga Closterium peracerosum-strigosum-littorale complex by square-pulse electroporation. New Phytologist, 233, 569–578.
- Klein, K., Wagner, G., & Blatt, M. R. (1980). Heavy-meromyosin-decoration of microfilaments from Mougeotia protoplasts. Planta, 150, 354–356.
- Kraml, M., Büttner, G., Haupt, W., & Herrmann, H. (1988). Chloroplast orientation in Mesotaenium: The phytochrome effect is strongly potentiated by interaction with blue light. In Tazawa M.(ed.), Cell Dynamics (pp. 172–179). Wien: Springer.
- Krieger, W. (1933). 39. Die Desmidiaceen. Rabenhorst’s Kryptogamen-Flora von Deutschland, Österreich Und Der Schweiz, 13.
- Krieger, W. (1937). Die Desmidiaceen Europas mit Berücksichtigung der auβereuropäischen Arten. Rabenhorst’s Kryptogamen Flora. Dt, Österr. Schweiz., 3, 377–712.
- Kumar, S., Stecher, G., Li, M., Knyaz, C., Tamura, K., & Battistuzzi, F. U. (2018). MEGA X: Molecular evolutionary genetics analysis across computing platforms. Molecular Biology and Evolution, 35, 1547–1549.
- Lemmermann, E. (1896). Zur Algenflora des Riesengebirges. Berlin: Friedländer & Sohn.
- Lenzenweger, R. (2003). Desmidiaceenflora von Österreich, Teil 4. Stuttgart: Schweizerbart’sche Verlagsbuchhandlung. https://www.schweizerbart.de/publications/detail/isbn/9783443600389/Desmidiaceenflora-von-sterreich-Teil-4
- Ling, H. U., & Seppelt, R. D. (1990). Snow algae of the Windmill Islands, continental Antarctica. Mesotaenium berggrenii (Zygnematales, Chlorophyta) the alga of grey snow. Antarctic Science, 2, 143–148.
- Lutz, S., Anesio, A. M., Edwards, A., & Benning, L. G. (2017). Linking microbial diversity and functionality of Arctic glacial surface habitats: Arctic glacial surface habitats. Environmental Microbiology, 19, 551–565.
- Lütz-Meindl, U. (2016). Micrasterias as a model system in plant cell biology. Frontiers in Plant Science, 7, 999.
- Mårtensson, O., & Nilsson, E. (1974). On the morphological colour of bryophytes. Lindbergia, 2, 145–159.
- Massana, R., Castresana, J., Balagué, V., Guillou, L., Romari, K., Groisillier, A., … Pedrós-Alió, C. (2004). Phylogenetic and ecological analysis of novel marine stramenopiles. Applied and Environmental Microbiology, 70, 3528–3534.
- Massana, R., Del Campo, J., Sieracki, M. E., Audic, S., & Logares, R. (2014). Exploring the uncultured microeukaryote majority in the oceans: Reevaluation of ribogroups within stramenopiles. The ISME Journal, 8, 854–866.
- Matasci, N., Hung, L.-H., Yan, Z., Carpenter, E. J., Wickett, N. J., Mirarab, S., … Wong, G. K. S., et al. (2014). Data access for the 1,000 Plants (1KP) project. GigaScience, 3, 2047–217X-3–17.
- McFadden, G. I., & Melkonian, M. (1986). Use of Hepes buffer for microalgal culture media and fixation for electron microscopy. Phycologia, 25, 551–557.
- Mollenhauer, D. (1986). Concepts and strategies for research in Mesotaeniaceae. Beiheft Zur Nova Hedwigia, 56, 43–60.
- Nacke, H., Thürmer, A., Wollherr, A., Will, C., Hodac, L., Herold, N., … Daniel, R. (2011). Pyrosequencing-based assessment of bacterial community structure along different management types in German forest and grassland soils. PLOS ONE, 6, e17000.
- Nägeli, C. (1849). Gattungen einzelliger Algen: Physiologisch und systematisch bearbeitet. Zürich: Friedrich Schulthess.
- Nedbalová, L., & Sklenár, P. (2008). New records of snow algae from the Andes of Ecuador. Arnaldoa, 15, 17–20.
- Newsome, A. G., & van Breemen, R. B. (2012). Characterization of the purple vacuolar pigment of Zygogonium ericetorum alga. Planta Medica, 78, J20.
- O’Brien, H. E., Parrent, J. L., Jackson, J. A., Moncalvo, J.-M., & Vilgalys, R. (2005). Fungal community analysis by large-scale sequencing of environmental samples. Applied and Environmental Microbiology, 71, 5544–5550.
- Palacio-López, K., Tinaz, B., Holzinger, A., & Domozych, D. S. (2019). Arabinogalactan proteins and the extracellular matrix of charophytes: A sticky business. Frontiers in Plant Science, 10, 447.
- Petlovany, O. V. (2014). Zygnematales. In Tsarenko, P. M., Wasser, S. P., Nevo, E.(Eds.), Algae of Ukraine: Diversity, nomenclature, taxonomy, ecology and geography (Vol. 4, pp. 11–61). Königstein: Koeltz Botanical Books.
- Pfeifer, L., Utermöhlen, J., Happ, K., Permann, C., Holzinger, A., von Schwartzenberg, K., & Classen, B. (2021). Search for evolutionary roots of land plant arabinogalactan-proteins in charophytes: Presence of a rhamnogalactan-protein in Spirogyra pratensis (Zygnematophyceae). The Plant Journal, 109, 568–584.
- Procházková, L., Řezanka, T., Nedbalová, L., & Remias, D. (2021). Unicellular versus filamentous: The glacial alga Ancylonema alaskana comb. et stat. nov. and its ecophysiological relatedness to Ancylonema nordenskioeldii (Zygnematophyceae, Streptophyta). Microorganisms, 9, 1103.
- Remias, D., Holzinger, A., & Lütz, C. (2009). Physiology, ultrastructure and habitat of the ice alga Mesotaenium berggrenii (Zygnemaphyceae, Chlorophyta) from glaciers in the European Alps. Phycologia, 48, 302–312.
- Remias, D., Schwaiger, S., Aigner, S., Leya, T., Stuppner, H., & Lütz, C. (2012). Characterization of an UV- and VIS-absorbing, purpurogallin-derived secondary pigment new to algae and highly abundant in Mesotaenium berggrenii (Zygnematophyceae, Chlorophyta), an extremophyte living on glaciers. FEMS Microbiology Ecology, 79, 638–648.
- Remias, D., Holzinger, A., Aigner, S., & Lütz, C. (2012). Ecophysiology and ultrastructure of Ancylonema nordenskiöldii (Zygnematales, Streptophyta), causing brown ice on glaciers in Svalbard (high Arctic). Polar Biology, 35, 899–908.
- Rippin, M., Becker, B., & Holzinger, A. (2017). Enhanced desiccation tolerance in mature cultures of the streptophytic green alga Zygnema circumcarinatum revealed by transcriptomics. Plant & Cell Physiology, 58, 2067–2084.
- Rippin, M., Lange, S., Sausen, N., & Becker, B. (2018). Biodiversity of biological soil crusts from the Polar regions revealed by metabarcoding. FEMS Microbiology Ecology, 94. doi:10.1093/femsec/fiy036
- Rippin, M., Borchhardt, N., Williams, L., Colesie, C., Jung, P., Büdel, B., Becker, B. (2018). Genus richness of microalgae and Cyanobacteria in biological soil crusts from Svalbard and Livingston Island: Morphological versus molecular approaches. Polar Biology, 15, 909–923.
- Samolov, E., Baumann, K., Büdel, B., Jung, P., Leinweber, P., Mikhailyuk, T., … Glaser, K. (2020). Biodiversity of algae and cyanobacteria in biological soil crusts collected along a climatic gradient in Chile using an integrative approach. Microorganisms, 8, 1047.
- Sørensen, I., Pettolino, F. A., Bacic, A., Ralph, J., Lu, F., O’Neill, M. A., … Willats, W. G. T. (2011). The charophycean green algae provide insights into the early origins of plant cell walls. The Plant Journal, 68, 201–211.
- Stastny, J., Skaloud, P., Langenbach, D., Nemjova, K., & Neustupa, J. (2013). Polyphasic evaluation of Xanthidium antilopaeum and Xanthidium cristatum (Zygnematophyceae, Streptophyta) species complex. Journal of Phycology, 49, 401–416.
- Šťastný, J., & Kouwets, F. A. C. (2012). New and remarkable desmids (Zygnematophyceae, Streptophyta) from Europe: Taxonomical notes based on LM and SEM observations. Fottea, 12, 293–313.
- Steyn, W. J., Wand, S. J. E., Holcroft, D. M., & Jacobs, G. (2002). Anthocyanins in vegetative tissues: A proposed unified function in photoprotection. New Phytologist, 155, 349–361.
- Sun, Y., Harpazi, B., Wijerathna-Yapa, A., Merilo, E., de Vries, J., Michaeli, D., … Mosquna, A. (2019). A ligand-independent origin of abscisic acid perception. Proceedings of the National Academy of Sciences, 116, 24892–24899.
- Surek, B., Beemelmanns, U., Melkonian, M., & Bhattacharya, D. (1994). Ribosomal RNA sequence comparisons demonstrate an evolutionary relationship between Zygnematales and charophytes. Plant Systematics and Evolution, 191, 171–181.
- Takeuchi, N. (2001). The altitudinal distribution of snow algae on an Alaska glacier (Gulkana Glacier in the Alaska Range). Hydrological Processes, 15, 3447–3459.
- Takeuchi, N., Kohshima, S., & Seko, K. (2001). Structure, formation, and darkening process of albedo-reducing material (cryoconite) on a Himalayan glacier: A granular algal mat growing on the glacier. Arctic, Antarctic, and Alpine Research, 33, 115–122.
- Takeuchi, N., & Kohshima, S. (2004). A snow algal community on Tyndall Glacier in the southern Patagonia icefield, Chile. Arctic, Antarctic, and Alpine Research, 36, 92–99.
- Timme, R. E., Bachvaroff, T. R., & Delwiche, C. F. (2012). Broad phylogenomic sampling and the sister lineage of land plants. PLOS ONE, 7, e29696.
- Turland, N. J., Wiersema, J. H., Barrie, F. R., Greuter, W., Hawksworth, D. L., Herendeen, P. S., … Smith, G. et al. (Eds.). (2018). International code of nomenclature for algae, fungi, and plants (Shenzhen Code) adopted by the Nineteenth International Botanical Congress Shenzhen, China, July 2017. Regnum Vegetabile 159. Glashütten: Koeltz Botanical Books. doi:10.12705/Code.2018
- Uetake, J., Naganuma, T., Hebsgaard, M. B., Kanda, H., & Kohshima, S. (2010). Communities of algae and cyanobacteria on glaciers in west Greenland. Polar Science, 4, 71–80.
- Wada, M., Grolig, F., & Haupt, W. (1993). New trends in photobiology: Light-oriented chloroplast positioning. Contribution to progress in photobiology. Journal of Photochemistry and Photobiology. B, Biology, 17, 3–25.
- Wagner, G., Haupt, W., & Laux, A. (1972). Reversible inhibition of chloroplast movement by cytochalasin B in the green alga Mougeotia. Science, 176, 808–809.
- Walden, S., Jauss, R.-T., Feng, K., Fiore-Donno, A. M., Dumack, K., Schaffer, S., … Bonkowski, M. (2021). On the phenology of protists: Recurrent patterns reveal seasonal variation of protistan (Rhizaria: Cercozoa and Endomyxa) communities in tree canopies. FEMS Microbiology Ecology, 97, fiab081.
- Wang, S., Liang, H., Xu, Y., Li, L., Wang, H., Sahu, D. N., … Liu, H. (2021). Genome-wide analyses across Viridiplantae reveal the origin and diversification of small RNA pathway-related genes. Communications Biology, 4, 1–11.
- West, W., & West, G. S. (1901). The alga-flora of Yorkshire: A complete account of the known freshwater algae of the county, with many notes on their affinities and distribution (Vol. 5). York: Yorkshire Naturalists’ Union.
- West, W., & West, G. S. (1904). A monograph of the British Desmidiaceae (Vol. 1). London: Ray Society.
- Wickett, N. J., Mirarab, S., Nguyen, N., Warnow, T., Carpenter, E., Matasci, N., … Leebens-Mack, J. (2014). Phylotranscriptomic analysis of the origin and early diversification of land plants. Proceedings of the National Academy of Sciences, 111, E4859–E4868.
- Williamson, C. J., Anesio, A. M., Cook, J., Tedstone, A., Poniecka, E., Holland, A., … Yallop, M. L. (2018). Ice algal bloom development on the surface of the Greenland Ice Sheet. FEMS Microbiology Ecology, 94, fiy025.
- Williamson, C. J., Cook, J., Tedstone, A., Yallop, M., McCutcheon, J., Poniecka, E., … Anesio, A. (2020). Algal photophysiology drives darkening and melt of the Greenland ice sheet. Proceedings of the National Academy of Sciences, 117, 5694–5705.
- Wodniok, S., Brinkmann, H., Glöckner, G., Heidel, A. J., Philippe, H., Melkonian, M., & Becker, B. (2011). Origin of land plants: Do conjugating green algae hold the key? BMC Evolutionary Biology, 11, 104.
- Yallop, M. L., Anesio, A. M., Perkins, R. G., Cook, J., Telling, J., Fagan, D., … Roberts, N. W. (2012). Photophysiology and albedo-changing potential of the ice algal community on the surface of the Greenland ice sheet. The ISME Journal, 6, 2302–2313.
- Yoshimura, Y., Kohshima, S., & Ohtani, S. (1997). A community of snow algae on a Himalayan glacier: Change of algal biomass and community structure with altitude. Arctic and Alpine Research, 29, 126.
- Zhou, H., Wilkens, A., Hanelt, D., & von Schwartzenberg, K. (2021). Expanding the molecular toolbox for Zygnematophyceae – Transient genetic transformation of the desmid Micrasterias radians var. evoluta. European Journal of Phycology, 56, 51–60.