ABSTRACT
The environment-friendly disposal approaches for sewage sludge remain a challenge worldwide, and agricultural application of the treated sewage sludge, i.e. biosolids, as soil conditioner and nutrient source for plants, is still one of the most promising options for dealing with the waste. In this study, we compared the effects of long-term applications of biosolids and mineral fertilizer on soil microbial community using phospholipid fatty acid profiles in an agricultural field. The microbes predominant in the mineral fertilizer soil remained the most abundant in the biosolids-amended soil. Bacterial and fungal communities presented downtrends in both absolute and relative amounts; however, percentage of Gram-positive bacteria remained unchanged. Soil pH displayed significant negative correlations with microbial communities and explained 64%, 56% and 44% of the variances in microbial biomass, bacteria and fungi, respectively, while soil NO3–-N was positively correlated with microbial biomass, bacteria and fungi (r2 = 0.53, 0.51 and 0.54, respectively).
Introduction
Biosolids are treated sewage sludge which is the major by-product of the wastewater treatment and characterized by the nutrient-rich organic materials. With rapid population growth and industrial development, we are confronting with the astonishing increases in municipal sewage sludge generation [Citation1]. The total output of dewatered sewage sludge reached 6.25 million tons in 2013 in China, with an average annual growth of 13% from 2007 to 2013 [Citation2]. However, the environment-friendly disposal approaches for sewage sludge remain a challenge worldwide.
Agricultural land application of treated sewage sludge, i.e. biosolids, as soil conditioner and nutrient source for plants, is one of the most promising options for dealing with the waste [Citation3–Citation5], since it has high contents of organic matter, nitrogen (N), phosphorus (P) and potassium (K) with great potentials of improving the quality and yield of crops as a good alternative to mineral fertilizers and upgrading soil conditions especially for fixing land degradation problems [Citation3,Citation6–Citation8]. In addition, biosolids are of other advantages over the traditionally inorganic fertilizer, for example, low cost and the nutrient slow-release that keeps nutrients constantly available and consequently promotes its efficiency for plants and soils [Citation9], may reduce the potential environmental pollution caused by leaching of excess nutrients that often happens in mineral fertilizer added soils [Citation10]. Nevertheless, sewage sludge is produced through different stages of wastewater treatment process [Citation11]; thus, it is not hard to imagine that sewage sludge as well as its subsequent product biosolids may still contain some pollutants such as heavy metals, toxic organic compounds and pathogens [Citation12–Citation14]. And the ‘safety’ issue has been always the highest priority for the agricultural application of biosolids made of sewage sludge [Citation12,Citation15,Citation16].
One of the key sticking points toward the agricultural application of biosolids is heavy metal pollution, which would negatively affect the quality of the amended soil and plants [Citation15]. Therefore, the influences of soil heavy metals already become one of the major topics in studies regarding biosolids application [Citation4,Citation16,Citation17]. Soil microbes are generally sensitive and quickly responsive to disturbances (for instance, environmental changes, agricultural practices, contamination) [Citation18,Citation19], and microbial parameters are often used as biological indicators to evaluated changes of soil in response to biosolids amendments [Citation20–Citation22]. However, there still has been disagreement on the responses of soil microbes to biosolids application in agricultural lands. For examples, some studies have documented that soil microbial activity and biomass increased following biosolids application [Citation23–Citation25], which could be interpreted by the improvement in available C sources without the N limitation (the C:N ratio of the SS was 5.5) [Citation26], while Epelde et al. [Citation27] and Burges et al. [Citation28] reported that biosolids negatively altered soil microbial communities and subsequently soil functionality because of heavy metals. Furthermore, the studies evaluating the influence of biosolids amendment on agricultural land often compare biosolids-amended soils with non-amended soils and often based on short period surveys [Citation3,Citation29]. Nowadays, croplands without adding any fertilizer can hardly be found, and croplands are commonly fertilized with mineral fertilizer. Therefore, the implication of the related research for agricultural application of biosolids should be emphasized, and to best protect both human health and the environment from the potential hazards, it is necessary to update technologies monitoring soil in response to biosolids amendments in a long term [Citation30].
In this study, the paired agricultural fields with normal mineral fertilizer application vs. biosolids amendment were selected for comparisons. We aimed to qualify the changes in soil microbial biomass and communities in response to the long-term biosolids amendment relative to the mineral fertilizer application using PLFAs profile.
Materials and methods
Field sites and fertilizers applied
The study area is located in a suburb of Tangshan City, Hebei Province, China. The area is featured by a continental warm and semi-humid monsoon climate. The mean annual temperature is 11°C, with minimum in January (−5.8°C) and maximum in July (25.4°C). The mean annual precipitation is 650 mm, approximately 60% of which falls during July and August. The soil is classified as fimiorthic anthrosols (loamy texture in the 0–15 cm-depth layer) in the Chinese soil taxonomic system or as Ustalfs in the USDA Soil Taxonomy. The study field, which was long fertilized with mineral fertilizer before, has been applied with commercial biosolids for 7 consecutive years. As a comparison, the adjacent field has been applied with mineral fertilizer all along. The two fields were mainly planted with ginger (Zingiber officinale) with the same agricultural management. The rhizome of ginger is a dominant produce in the local area because of its high economic value in China. According to the interview with the land growers, the annual yields in the field before and after the biosolids application were similar, which was one of the main reasons for continuous application of the biosolids in this field. Another interest for the growers was the low cost of the biosolids.
The commercial biosolids applied in the study field was originated from municipal household wastewater and was produced by the Municipal Wastewater Treatment Plant in Tangshan City. The wastewater treatment plant adopts traditional activated sludge treatment on a basis of impeller surface aeration to get purified water and simultaneously produce sludge. The activated sludge technology is one of the most widely applied methods in the field regarding wastewater aerobic bio-chemical technology at present, and it mainly consists of aeration tank, primary and secondary sedimentation tank, aeration system and sludge return system. Through the procedures of aerobic fermentation, dehydration and pelletizing, the sludge was eventually produced into the biosolids completely safe for agriculture.
The mineral fertilizer consisted of N, P2O5 and K2O, which are greater than or equal to 150 g kg−1, respectively. The concentrations of N, P and K in the biosolids were 25.8, 37.6 and 28.3 g kg−1, respectively. The concentrations of heavy metal in the biosolids were 34 mg lead kg−1, 1 mg cadmium kg−1, 424 mg zinc kg−1 and 61 mg copper kg−1 [Citation13]. The fertilization rates were 1.5 t ha−1 year−1 for mineral fertilizer and 4.5 t ha−1 year−1 for biosolids.
Soil sampling and laboratory analysis
Soil samples were collected at 0–15 cm depth prior to the ginger harvest. Six random sampling sites were identified to serve as replicates within each field. The sites were at least 20 m apart from each other and 20 m away from the boundary. All the soil samples were immediately transported to the laboratory, and sieved through a 2-mm mesh in order to remove visible plant materials, small insect and stones. Of the sieved fresh soil, one subsample was immediately used to measure water content by oven-drying at 105°C for 24 h, one subsample was air-dried and ground (<0.15 mm) for the analyses of soil organic carbon (SOC) and total nitrogen (TN), and the rest was stored at 4°C for the analyses of soil pH, nitrate nitrogen (NO3–-N), ammonium nitrogen (NH4+-N), dissolved organic carbon (DOC), dissolved nitrogen (DN) and microbial PLFAs within two weeks.
SOC and TN were determined by a CHNOS Elemental Analyzer (vario El III, Elementar Analysensysteme GmbH, Germany) [Citation31]. Soil pH was measured in a 1:5 (w/v) soil:water suspension by a pH meter. For the measurements of soil NO3–-N, NH4+-N, DOC and DN, the field-moist soil (equivalent to 10 g dry weight) was mixed with 50 ml of 2 M KCl in a 100 ml polypropylene bottle and shaken on a reciprocating shaker at 200 rev min−1 for 30 min [Citation32]. The soil extract was then centrifuged at 8000 × g for 10 min, and the supernatant was recovered and stored in a polypropylene bottle in a −20°C freezer before analysis. The concentrations of NO3–-N and NH4+-N in the supernatant were analyzed with an autoanalyzer (AA3, SEAL Analytical GmbH, Germany). The concentrations of DOC and DN in the supernatant were determined by a TOC/TNb analyzer (Liqui TOC II, Elementar Analysensysteme GmbH, Germany).
We used phospholipid fatty acid (PLFA) analysis to determine changes in the soil microbial biomass and microbial community structure in response to the shift of soil amendment. PLFA analysis included lipid extraction and fractionation and GC-MIDI analysis. The PLFAs were extracted using the method suggested by Zelles and Bai [Citation27]. PLFA profiles were prepared following the MIDI protocol, analyzed through the MIDI Sherlock® Microbial Identification System (MIDI, Newark, DE), and separated by gas chromatography (Agilent 6850, Agilent Technologies, USA) with flame ionization detector on capillary column ultra 2, 4μl injection, with a split mode (1:20). All the results were reported on a dry weight basis. The nomenclature of the fatty acid is based on the description of the associated studies [Citation33–Citation36]. Fatty acid biomarker groups are shown in . Some individual fatty acids were used as signatures for various microbial functional groups [Citation35–Citation38].
Table 1. PLFA markers used for taxonomic microbial groups in this study.
Statistical analysis
Means and standard errors were calculated for all the measured variables. Student’s t test was employed to compare means. Pearson’s correlation coefficients were calculated to correlate soil properties with soil microbial community structure. All statistical analyses were performed using the SAS 9.2 for windows (SAS Institute Inc. Cary, NC).
Results
Soil physiochemical characteristics in response to the shift of soil amendment
The shift in soil amendment from mineral fertilizer to biosolids had significant effects on soil properties (). Soil pH value in the field amended with biosolids was greater than that in the field with mineral fertilizer (p = 0.003), and the opposing patterns were observed on other soil chemical properties. The concentrations of SOC, TN and DOC decreased in response to the shift of soil amendment (p = 0.003, p < 0.001 and p = 0.019, respectively). Meanwhile, the shift of soil amendment substantially affected NO3–N rather than NH4+-N. Soil NO3–N concentration in the biosolids-amended soil decreased by approximately 66% compared with that in the mineral fertilizer added soil. No significant differences were found in soil moisture and DN between the soils amended with biosolids and mineral fertilizer.
Table 2. Comparison of soil properties (0–15 cm depth) between mineral fertilizer application (MFA) and biosolids application (BSA). Values are means ± SE (n = 6). SOC: soil organic carbon; TN: total nitrogen; NH4+-N: ammonium nitrogen; NO3–-N: nitrate nitrogen; DOC: dissolved organic carbon; DN: dissolved nitrogen.
Changes in microbial community compositions in the soils with different amendments
As expected, the biosolids application altered the structure of soil microbial community not only in quantity but also in composition, in comparison with the mineral fertilizer application (). In the mineral fertilizer added soil, a total of 31 individual microbial PLFAs with 14- to 24-carbon chain lengths were identified. However, in the biosolids-amended soil, approximately 74% of these microbial PLFAs recorded significant decreases in amount, and in particular, some PLFAs were even not detectable (e.g. PLFAs 17:0, 20:4ω6c, 20:1ω9c and 20:0).
Table 3. Absolute amounts (nmol g−1) of all identified PLFAs in soils with mineral fertilizer application (MFA) and biosolids application (BSA). Values are means ± SE (n = 6). n.d. indicates that the PLFA was not detectable.
Variations in soil microbial biomass and community structure
The replacement of mineral fertilizer with biosolids in an agricultural land led to significant changes in soil microbial groups (). The biosolids application altered the amount of the total PLFA, down 18% (p < 0.01), relative to the mineral fertilizer application. The total PLFA was used as a measure of microbial biomass. Bacterial and fungal groups also presented a significant downtrend in both absolute amount and relative amount in response to the shift of soil amendment. The ratio of Gram-positive bacteria to Gram-negative bacteria increased (p < 0.001) but the ratio of fungi to bacteria did not show a significant change in the biosolids-amended soil, compared with those in the mineral fertilizer added soil ().
Figure 1. Amounts (a) and percentages (b) of PLFAs of different microbial groups in soils with mineral fertilizer application (MFA) and biosolids application (BSA). GP, GN, SP and AM represent Gram-positive bacterial PLFAs, Gram-negative bacterial PLFAs, saprophytic fungal PLFAs and arbuscular mycorrhiza fungal PLFAs, respectively. Values are means ± SE (n = 6). *,** and *** denote significant differences between MFA and BSA at p < 0.05, p < 0.01 and p < 0.001, respectively.
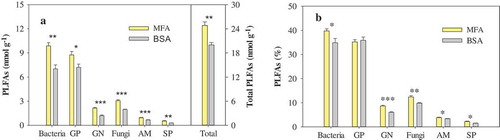
Figure 2. The ratios of fungal PLFAs to bacterial PLFAs (F/B) and Gram-positive bacterial PLFAs to Gram-negative bacterial PLFAs (GP/GN) in the soils with mineral fertilizer application (MFA) and biosolids application (BSA). Values are means ± SE (n = 6). *** denotes significant differences between MFA and BSA at p < 0.001.
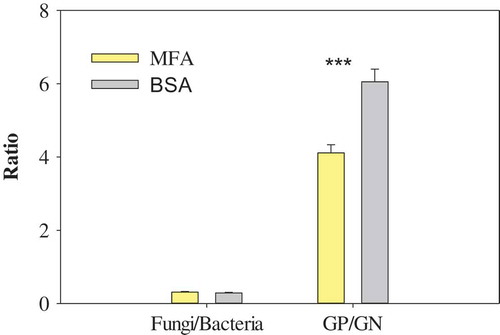
Correlations of soil properties with soil microbial community
Soil pH value was negatively correlated with the total PLFA (r2 = 0.64, p < 0.01) and all the microbial communities except saprophytic (SP) fungal group (). In contrast, soil organic carbon and total nitrogen presented significant positive correlations with the Gram-negative bacteria, fungi, arbuscular mycorrhizal (AM) fungi and SP fungi. Soil NH4+-N was only negatively correlated with bacteria; however, soil NO3–-N showed significant positive correlations with the total PLFA (r2 = 0.53, p < 0.01) and all the microbial communities. Similarly, soil DOC was significantly correlated with the total PLFA, Gram-negative bacteria and fungal groups. No significance was found in the correlations of soil moisture and dissolved nitrogen with the soil microbial community.
Table 4. Pearson correlation coefficients between soil properties and microbial communities. SOC: soil organic carbon; TN: total nitrogen; DOC: dissolved organic carbon; DN: dissolved nitrogen; NH4+-N: ammonium nitrogen; NO3–-N: nitrate nitrogen; PLFA: phospholipid fatty acids; GP: Gram-positive bacteria; GN: Gram-negative bacteria; AM: arbuscular mycorrhiza fungi; SP: saprophytic fungi; F/B: fungi/bacteria.
In addition, the ratio of fungi to bacteria was only correlated with SOC, whereas the ratio of Gram-positive bacteria to Gram-negative bacteria had significant correlations with soil pH, SOC, TN, NO3–-N and DOC (r2 = 0.42, 0.67, 0.65, 0.41 and 0.57, respectively).
Discussion
In organic-fertilizer-amended soils, organic matter inputs build the mineralizable organic N pool, which contributes to the soil N supply. The advantage of organic fertilizer amendment is expected to reduce mineral N fertilizer inputs, resulting in more economic crop production and environmental protection. In this study, we replaced mineral fertilizer with biosolids in an agricultural field to investigate the response of soil microbial community with implications for soil quality and environmental safety.
Soil properties and microbes in response to the shift of soil amendment
The shift in soil amendment from mineral fertilizer to biosolids made significant differences to the soil properties. This biosolids amendment had adverse influences on the soil properties, with an increase in soil pH value and a drop in soil NO3–-N (). Effects of biosolids application on soils were usually driven by a wide variety of factors, such as climate characteristics, vegetation community, soil type and texture and differences in biosolids properties [Citation3,Citation5,Citation11,Citation39]. Therefore, it is not unexpected that effects of biosolids on soils came out with different results from other related studies [Citation16,Citation40,Citation41]. In this study, it is particularly noteworthy that the NO3–-N concentration in the biosolids-amended soil was only approximately one-third of that in the mineral fertilizer treated soil. As described in Materials and Methods, the initial nitrogen input in the biosolids-amended soil was approximately half of that in the mineral fertilizer added soil, which did not consequently lower the annual yield of the sludge field. Furthermore, different from mineral fertilizer, biosolids slowly releases nutrients into soils [Citation10]. Therefore, soil NO3–-N concentration in the biosolids soil was much lower than that in the mineral fertilizer soil. More importantly, the lower NO3–-N concentration in the biosolids treated soil might decrease the amount of N leaching from the soil and subsequently reduce the risk of water pollution [Citation11].
The biosolids amendment resulted in a decrease in the soil microbial biomass (i.e. the total PLFA) (). It suggested that the soil microbes that were habituated to the soil with long-term mineral fertilizer appeared to be susceptible to the biosolids as a substitute for the mineral fertilizer. Overall, the application of biosolids had a marked negative effect on the composition of soil microbial community (), which was consistent with the results of other related studies [Citation41]. Noticeably, some of the soil microbial PLFAs were not detectable in the soil amended with biosolids, for instance, saturated PLFA17:0, a bacterial biomarker [Citation36], and PLFA 20:4ω6c, an indicator of protozoa [Citation37]. This phenomenon indicated that these specific populations of soil microorganisms were not able to adapt to the new soil substrate with biosolids. There were exceptions against the decreasing tendency of individual PLFA amounts in response to the change in soil amendment from mineral fertilizer to biosolids application, i.e. the increases in certain microbial PLFAs (e.g. C16N Alcohol and 21:1ω3c) in the biosolids amendment, suggesting that some microbes to some extent could benefit from biosolids application, but their influences on the entire soil microbial community were minor. In addition, no significant effects were found on several microbial PLFAs, particularly on ubiquitous PLFA 16:0, an indicator of pseudomonas (aerobic Gram-negative bacteria) [Citation35].
Dominant microbes tolerant to change in soil amendment
There were four microbial PLFAs predominant in the mineral fertilizer soil, i.e. 16:0, i17:1G, i15:0 and16:1ω7c, which accounted for 48% of the total PLFA. Likewise, these microbial PLFAs still remained the most abundant in the biosolids-amended soil, accounting for 51% of the total PLFA (). The results suggested these microbes could gradually adapt to the change in soil amendment and subsequently keep living with the most numerous in the biosolids-amended soil, even though the biosolids amendment, in general, gave rise to relative decreases in soil microbial PLFAs. Therefore, these microbes were the major contributors to the soil quality during the biosolids amendment.
Soil bacterial and fungal groups
The soil amendment shift to the biosolids greatly stimulated the two principal decomposer groups in soils, i.e. bacteria and fungi. The ratio of fungi to bacteria demonstrated that the two communities presented proportional decreases in size (). The total bacteria, Gram-positive and Gram-negative bacteria displayed a downward trend in absolute amount in response to the biosolids application. Nevertheless, Gram-positive bacteria did not significantly change in proportion while percentages of the total bacteria and Gram-negative bacteria decreased in the biosolids-amended soil ()). These results implied that Gram-positive bacteria were less sensitive than Gram-negative bacteria in response to the biosolids application. Among all the detected microbial PLFAs, some specific PLFAs presented stronger responses to the biosolids amendment. Microbial PLFA i17:1 G contributed 53% to the decrease in the amount of Gram-positive bacterial group [Citation26], while PLFA 16:1ω7c and cy19:0 were the major contributors to the decrease in Gram-negative bacterial group [Citation33]. Similarly, the biosolids application reduced both amounts and percentages of the soil fungal, AM fungal and SP fungal groups. Soil microbial monounsaturated PLFA 18:1ω9c, a fungal PLFA biomarker [Citation36], accounted for 54% of the decrease in the fungal group.
Influential factors in changes of soil microbial community structure due to biosolids application
It is well documented that soil physical and chemical properties are crucial variables controlling soil microbial community in an agricultural system [Citation42,Citation43]. Undoubtedly, the effects of agricultural management practices (e.g. fertilization) on soil microorganisms also count [Citation44,Citation45]. Fertilizer, which largely shapes the quality of soil substrate interacting with soil physiochemical factors, can become a vital driver in modifying soil microbial community [Citation46,Citation47]. In our study, the agricultural soil that long applied with mineral fertilizer before has been amended with biosolids for 7 consecutive years. On the one hand, the results indicated that the soil microbial community exhibited to be susceptible to the biosolids amendment. On the other hand, the changes of soil properties in response to the shift of soil amendment might be interactional with the differences in the composition of soil microbial community utilizing the nutrients between mineral fertilizer and biosolids. As a matter of fact, most of soil microorganisms can only live and reproduce within favorite soil environmental conditions, such as the contents of nutrients [Citation48], pH [Citation49,Citation50], temperature and moisture [Citation43]. In this study, we found that two key factors were responsible for the changes in soil microbial communities. One was soil pH. Generally, microbes are sensitive to the change in hydrogen ion concentration in the living environment, and thereby soil pH is considered to be one of the most influential factors to soil microbial community [Citation51,Citation52]. In our study, soil pH displayed significant negative correlations with microbial communities and explained 64%, 56% and 44% of the variances in microbial biomass, bacterial group and fungal group, respectively (). The other key factor was soil NO3–-N. Soil NO3–-N was positively correlated with soil microbial communities, accounting for 53%, 51% and 54% of the variances in soil microbial biomass, bacteria and fungi, respectively. Nitrogen is the primary limiting nutrient to plants as well as microbes in most terrestrial ecosystems [Citation53]. The substantial decrease in soil NO3–-N in the biosolids-amended soil implied that the competition for N could be more intensive between plants and microbes [Citation54], and soil microbial growth might be consequently suppressed by plant (ginger) utilization of N [Citation55]. It would eventually lead to the decrease in the size of microbial community [Citation48].
The changes in soil microbial community structure and the decrease in soil microbial biomass after the seven-year biosolids amendment, provide important insights regarding the sensitivity of soils microbes to biosolids amendment. Some studies have reported increases or non-changes in soil microbial biomass in response to biosolids amendment [Citation3,Citation29], which creates an interpretational dilemma for our results. One best explanation is the duration of biosolids amendment. For example, in the investigation of Zoghlami, Hamdi [Citation3], following the two successive annual amendments with sewage sludge, the microbial communities were enhanced and however soil pH was unchanged. Nevertheless, in our study, after the longer period (7 years) of biosolids amendment, the increase in soil pH can explain the decrease in microbial biomass. The other interpretation for the decrease of the soil microbial biomass, at least in part, is differences in the associated vegetation and agricultural management among studies. The comprehensive assessment including soil quality, crop yield and environmental safety determined the feasibility of long-term biosolids amendment. Our findings facilitate modifying the corresponding agricultural management and achieving long-term sustainability of an agroecosystem amended with biosolids.
Conclusions
The 7-year amendment of biosolids altered the microbial community structure with downtrends in absolute and relative amounts of bacterial and fungal communities. This finding suggests that excessive biosolids application may pose risks on soil microbes. However, the microbes predominant in the mineral fertilizer soil remained the most abundant in the biosolids-amended soil. The lower nitrate concentration observed in the biosolids added soil indicates that application of biosolids as a substitute for inorganic fertilizer can mitigate the environmental pollution caused by excessive N leached from soils. Therefore, together with the low cost of biosolids and the crop yield that matched up to the yield from the mineral fertilizer added field, it is convincible that biosolids amendment in the arable land studied has distinctive advantages and feasibility of long-term application, especially with mineral fertilizers.
Data availability
All of the data that support the findings of this study can be requested from J.C.
Acknowledgments
We thank Dr. Kai Gao for his help in field sampling and Dr. Qibing Wang for his help in data analyses and interpretation.
Disclosure statement
No potential conflict of interest was reported by the authors.
Additional information
Funding
References
- He PJ, Lü F, Zhang H, et al. Sewage sludge in China: challenges toward a sustainable future. Water Pract Technol. 2007;2:wpt2007083.
- Yang G, Zhang G, Wang H. Current state of sludge production, management, treatment and disposal in China. Water Res. 2015;78:60–73.
- Zoghlami RI, Hamdi H, Mokni-Tlili S, et al. Changes in light-textured soil parameters following two successive annual amendments with urban sewage sludge. Ecol Eng. 2016;95:604–611.
- Borjesson G, Kirchmann H, Katterer T. Four Swedish long-term field experiments with sewage sludge reveal a limited effect on soil microbes and on metal uptake by crops. J Soils Sediment. 2014;14(1):164–177.
- Tontti T, Poutiainen H, Heinonen-Tanski H. Efficiently treated sewage sludge supplemented with nitrogen and potassium is a good fertilizer for cereals. Land Degrad Dev. 2016. DOI:10.1002/ldr.2528
- Jakubus M. Estimation of phosphorus bioavailability from composted organic wastes. Chem Speciation Bioavailability. 2016;28(1–4):189–198.
- Cogger CG, Bary AI, Kennedy AC, et al. Long-term grop and soil response to biosolids applications in dryland wheat. J Environ Qual. 2013;42(6):1872–1880.
- Roig N, Sierra J, Martí E, et al. Long-term amendment of Spanish soils with sewage sludge: effects on soil functioning. Agr Ecosyst Environ. 2012;158:41–48.
- Gil MV, Carballo MT, Calvo LF. Modelling N mineralization from bovine manure and sewage sludge composts. Bioresour Technol. 2011;102(2):863–871.
- Ghazy M, Dockhorn T, Dichtl N. Sewage sludge management in Egypt: current status and perspectives towards a sustainable agricultural use. World Acad Sci Eng Technol. 2009;57:299–307.
- Tarrasón D, Ojeda G, Ortiz O, et al. Differences on nitrogen availability in a soil amended with fresh, composted and thermally-dried sewage sludge. Bioresour Technol. 2008;99(2):252–259.
- Krzyzanowski F Jr., Lauretto M, Nardocci AC, et al. Assessing the probability of infection by Salmonella due to sewage sludge use in agriculture under several exposure scenarios for crops and soil ingestion. Sci Total Environ. 2016;568:66–74.
- Wu L, Cheng M, Li Z, et al. Major nutrients, heavy metals and PBDEs in soils after long-term sewage sludge application. J Soils Sediment. 2012;12(4):531–541.
- Assis Rodrigues Nogueira T, José de Melob W, Rodrigues OL, et al. Nickel in soil and maize plants grown on an oxisol treated over a long time with sewage sludge. Chem Speciation Bioavailability. 2009;21(3):165–173.
- Ahmadpour P, Nawi AM, Abdu A, et al. Uptake of heavy metals by Jatropha curcas L. planted in soils containing sewage sludge. Am J Appl Sci. 2010;7(10):1291–1299.
- Fließbach A, Martens R, Reber HH. Soil microbial biomass and microbial activity in soils treated with heavy metal contaminated sewage sludge. Soil Biol Biochem. 1994;26(9):1201–1205.
- Anderson IC, Parkin PI, Campbell CD. DNA- and RNA-derived assessments of fungal community composition in soil amended with sewage sludge rich in cadmium, copper and zinc. Soil Biol Biochem. 2008;40(9):2358–2365.
- Barrutia O, Garbisu C, Epelde L, et al. Plant tolerance to diesel minimizes its impact on soil microbial characteristics during rhizoremediation of diesel-contaminated soils. Sci Total Environ. 2011;409:4087–4093.
- Pardo T, Clemente R, Epelde L, et al. Evaluation of the phytostabilisation efficiency in a trace elements contaminated soil using soil health indicators. J Hazard Mater. 2014;268:68–76.
- Wang M, Xue J, Horswell J, et al. Long-term biosolids application alters the composition of soil microbial groups and nutrient status in a pine plantation. Biol Fertili Soils. 2017;53:799–809.
- Cogger CG, Bary AI, Kennedy AC, et al. Long-term grop and soil response to biosolids applications in dryland wheat. J Environ Qual. 2013;42:1872–1880.
- Kelly JJ, Policht K, Grancharova T, et al. Distinct responses in ammonia-oxidizing archaea and bacteria after addition of biosolids to an agricultural soil. Appl Environ Microbiol. 2011;77:6551–6558.
- Carbonell G, Pro J, Gómez N, et al. Sewage sludge applied to agricultural soil: ecotoxicological effects on representative soil organisms. Ecotoxicol Environ Saf. 2009;72:1309–1319.
- Mattana S, Petrovicová B, Landi L, et al. Sewage sludge processing determines its impact on soil microbial community structure and function. Appl Soil Ecol. 2014;75:150–161.
- Xue D, Huang X. The impact of sewage sludge compost on tree peony growth and soil microbiological, and biochemical properties. Chemosphere. 2013;93:583–589.
- Urra J, Alkorta I, Mijangos I, et al. Application of sewage sludge to agricultural soil increases the abundance of antibiotic resistance genes without altering the composition of prokaryotic communities. Sci Total Environ. 2019;647:1410–1420.
- Epelde L, Becerril JM, Kowalchuk GA, et al. Impact of metal pollution and Thlaspi caerulescens growth on soil microbial communities. Appl Environ Microbiol. 2010;76:7843–7853.
- Burges A, Epelde L, Garbisu C. Impact of repeated single-metal and multi-metal pollution events on soil quality. Chemosphere. 2015;120:8–15.
- Debosz K, Petersen SO, Kure LK, et al. Evaluating effects of sewage sludge and household compost on soil physical, chemical and microbiological properties. Appl Soil Ecol. 2002;19(3):237–248.
- Lowman A, McDonald MA, Wing S, et al. Land application of treated sewage sludge: community health and environmental justice. Environ Heal Perspect. 2013;121:537–542.
- Sollins P, Glassman C, Paul EA, et al. Soil carbon and nitrogen: pools and fractions. In: Robertson GP, Coleman DC, Bledsoe CS, et al., editors. Standard soil methods for long-term ecological research. Long-term ecological research network series. New York (NY): Oxoford University Press; 1999. p.89–105.
- Jones DL, Willett VB. Experimental evaluation of methods to quantify dissolved organic nitrogen (DON) and dissolved organic carbon (DOC) in soil. Soil Biol Biochem. 2006;38(5):991–999.
- Frostegård A, Bååth E. The use of phospholipid fatty acid analysis to estimate bacterial and fungal biomass in soil. Biol Fert Soils. 1996;22(1–2):59–65.
- Ippolito JA, Barbarick KA, Stromberger ME, et al. Water treatment residuals and biosolids long-term co-applications effects to semi-arid grassland soils and vegetation. Soil Sci Soc Am J. 2009;73(6):1880–1889.
- Marshall CB, McLaren JR, Turkington R. Soil microbial communities resistant to changes in plant functional group composition. Soil Biol Biochem. 2011;43(1):78–85.
- Zhang W, Parker KM, Luo Y, et al. Soil microbial responses to experimental warming and clipping in a tallgrass prairie. Global Change Biol. 2005;11(2):266–277.
- Ringelberg DB, Stair JO, Almeida J, et al. Consequences of rising atmospheric carbon dioxide levels for the belowground microbiota associated with white oak. J Environ Qual. 1997;26(2):495–503.
- Pankhurst CE, Pierret A, Hawke BG, et al. Microbiological and chemical properties of soil associated with macropores at different depths in a red-duplex soil in NSW Australia. Plant Soil. 2002;238(1):11–20.
- Fernandes SAP, Bettiol W, Cerri CC. Effect of sewage sludge on microbial biomass, basal respiration, metabolic quotient and soil enzymatic activity. Appl Soil Ecol. 2005;30(1):65–77.
- Cherif H, Ayari F, Ouzari H, et al. Effects of municipal solid waste compost, farmyard manure and chemical fertilizers on wheat growth, soil composition and soil bacterial characteristics under Tunisian arid climate. Eur J Soil Biol. 2009;45(2):138–145.
- Kelly JJ, Haèggblom M, Tate RLI. Effects of the land application of sewage sludge on soil heavy metal concentrations and soil microbial communities. Soil Biol Biochem. 1999;31:1467–1470.
- Jangid K, Williams MA, Franzluebbers AJ, et al. Relative impacts of land-use, management intensity and fertilization upon soil microbial community structure in agricultural systems. Soil Biol Biochem. 2008;40(11):2843–2853.
- Kechavarzi C, Dawson Q, Bartlett M, et al. The role of soil moisture, temperature and nutrient amendment on CO2 efflux from agricultural peat soil microcosms. Geoderma. 2010;154(3–4):203–210.
- Berthrong S, Buckley D, Drinkwater L. Agricultural management and labile carbon additions affect soil microbial community structure and interact with carbon and nitrogen cycling. Microb Ecol. 2013;66(1):158–170.
- Tian J, Fan M, Guo J, et al. Effects of land use intensity on dissolved organic carbon properties and microbial community structure. Eur J Soil Biol. 2012;52:67–72.
- Kallenbach C, Grandy AS. Controls over soil microbial biomass responses to carbon amendments in agricultural systems: a meta-analysis. Agr Ecosyst Environ. 2011;144(1):241–252.
- Bonanomi G, De Filippis F, Cesarano G, et al. Organic farming induces changes in soil microbiota that affect agro-ecosystem functions. Soil Biol Biochem. 2016;103:327–336.
- Wang J, Bakken LR. Competition for nitrogen during mineralization of plant residues in soil: microbial response to C and N availability. Soil Biol Biochem. 1997;29:163–170.
- Aciego Pietri JC, Brookes PC. Substrate inputs and pH as factors controlling microbial biomass, activity and community structure in an arable soil. Soil Biol Biochem. 2009;41(7):1396–1405.
- Rousk J, Brookes PC, Bååth E. The microbial PLFA composition as affected by pH in an arable soil. Soil Biol Biochem. 2010;42(3):516–520.
- Fernández-Calviño D, Martín A, Arias-Estévez M, et al. Microbial community structure of vineyard soils with different pH and copper content. Appl Soil Ecol. 2010;46(2):276–282.
- Aciego Pietri JC, Brookes PC. Relationships between soil pH and microbial properties in a UK arable soil. Soil Biol Biochem. 2008;40(7):1856–1861.
- Vitousek PM, Howarth RW. Nitrogen limitation on land and in the sea: how can it occur? Biogeochem. 1991;13:87–115.
- Kaye JP, Hart SC. Competition for nitrogen between plants and soil microorganisms. Trends Ecol Evol. 1997;12(4):139–143.
- Hu S, Chapin FS, Firestone MK, et al. Nitrogen limitation of microbial decomposition in a grassland under elevated CO2. Nature. 2001;409:188–191.