ABSTRACT
CuO particles can be used as an agrochemical with slow and controlled release of micronutrients but, their size-specific effects have not been well elucidated. In this study, soil-grown lettuce was exposed to CuO nanoparticles (nCuO) and CuO microparticles (μCuO) at 0, 200, and 400 mg Cu/kg for 60 days. At harvest, nCuO released 82.2% and 117.7% more Cu ions, as well as 48.7% and 75.3% more DTPA-extractable Cu than μCuO at 200 and 400 mg/kg, respectively. CuO treatments induced higher copper accumulated in lettuce roots but did not change shoot’s Cu content or cause oxidative stress. nCuO treatments increased shoot’s biomass by 16.3–19.1%, while μCuO showed no improvement to the biomass. The beneficial effects on lettuce by nCuO may be attributed to its modification in plant photosynthesis system with increased transpiration rate and higher stomatal conductance. Results indicate that nCuO can be a promising agrochemical fertilizer to increase crop production.
1. Introduction
Copper is an essential crop micronutrient, participating directly in redox enzymes and involving in the synthesis of chlorophyll and metabolism of protein and carbohydrate [Citation1]. Copper deficiency plants have reduced disease resistance and decreased crop yields [Citation2,Citation3]. However, Cu excess can also lead to negative effects on plant development and production, even leading plant withering to death [Citation4,Citation5]. Consequently, it is at a high risk to use soluble CuSO4 as a micronutrient fertilizer to soils. Compared with such salt products with high solubility, safe agrochemicals with slower and controlled nutrient release characteristics are needed.
Copper-based nanoparticles have been already presented on agrochemical market as pesticides or fungicides, entering into agroecosystem and affecting soil-plant systems [Citation6–Citation8]. There have been studies regarding the fate and bioavailability of Cu compounds in soils [Citation9,Citation10]. However, there are limited studies on divergence in effects of bulk and nano-particulate Cu compounds on soil-plant systems. It is, therefore, necessary to determine whether the effects of CuO nanoparticles (nCuO) are different from those of CuO microparticles (μCuO) in order to assess the impacts of Cu-based nanoparticles on agroecosystems.
Lettuce (var. ramosa Hort.) is a vegetable consumed fresh worldwide. In the current study, the physiological and biochemical responses of soil-grown lettuce to CuO nanoparticles and microparticles were evaluated. The copper fractionation in soil, biomass, contents of chlorophyll and malondialdehyde, as well as photosynthetic index and copper accumulation in harvested lettuce were determined to compare effects of nCuO with those of μCuO.
2 Materials and methods
2.1 Characterization of nCuO and μCuO
Copper oxide nanoparticles and microparticles were purchased from Sigma Aldrich Co., St. According to previous studies, the primary particle sizes are 10–100 nm and 100–10,000 nm, and zeta potential are −34.4 ± 0.5 mV and −42.7 ± 0.2 mV for nCuO and μCuO, respectively [Citation11,Citation12].
2.2 Preparation of suspensions/solutions and soil
The soil was collected from a vegetable field in Ningbo, Zhejiang Province. The pH value of the soil was 7.38 ± 0.05 and the concentration of elemental Cu in soil was 52.5 ± 2.6 mg/kg. nCuO and μCuO suspensions were prepared in millipore water and homogenized using ultrasonic in a 5°C water bath for 30 min and mixed with 1 kg soil to have 0 (control), 200 and 400 mg Cu per kg soil (dry weight). The spiked soil was placed in plastic pots and seeded 24 h after spiking. There were four replicates for each treatment.
2.3 Plant growth and harvest
There were 10 lettuce seeds per pot. Lettuce seeds were purchased online from Shanghai City. They were planted at 1 cm depth from the surface and placed into a greenhouse with temperature at 25°C, relative humidity of 55%, light intensity of 200 μmol m−2 s−1 and a 14 h photoperiod. The plants were watered with 100 ml tap water (no Cu detected) per pot every day without fertilizer application. The lettuce was thinned to 5 plants per pot 10 days after germination and harvested 60 days after germination. The plants were rinsed with tap water to remove excess soil, soaked in 0.01 M HNO3 for about 1 min, and rinsed for three times with millipore water.
2.4 Copper sequential extraction of soil and concentration in lettuce
Two standard extraction fluids were used in this study according to Gao et al. [Citation13]. 0.35 g of soil was extracted with 3.5 ml of CaCl2 (0.01 M), while 2.5 g of soil was extracted with 5 ml of DTPA (0.01 M CaCl2, 0.005 M DTPA, and 0.1 M triethanolamine (pH 7.6)). All extractions were put in a reciprocal shaker at 200 rpm for 2 h and then centrifuged at 3000 rpm for 10 min. The supernatant samples were filtered by a 0.45 μm filter and analyzed for copper concentration. For copper analysis in lettuce roots and shoots, samples of 100 mg of oven dried tissues were digested with HNO3 and HClO4 (4:1, v/v) and adjusted to 10 ml with millipore water. Element copper was analyzed using an inductively coupled plasma-optical emission spectroscopy (ICP-OES, Optima 5300 DV, Perkin-Elmer, USA).
2.5 Malondialdehyde and oxidative stress detection
Contents of malondialdehyde (MDA) were determined in fresh lettuce leaves at harvest as per Ohkawa et al. (Citation14), using kit purchased from Nanjing Jiancheng Bioengineering Institute. MDA contents were monitored at 532 nm using a Microplate Reader (M200, Tecan Infinite, SUI) and calculated as nmol/mg fresh weight. Reactive oxygen species (ROS) were measured according to Dai et al. (Citation15). Briefly, 0.1 g fresh leaves were grounded and incubated in 10 μmol/L 2ʹ,7ʹ-dichlorofluorescin diacetate (H2DCFDA, prepared in 20 mM PBS, pH 7.8) with fluorescent probes for 30 min and then centrifuged at 3000 rpm for 10 min. The supernatant was quantified for the fluorescence intensity (FI) using the Microplate Reader. The excitation and emission wavelengths were set at 485 and 525 nm, respectively.
2.6 Photosynthetic index
Prior to harvest, the photosynthetic rate (Pn), stomatal conductance (Gs), intercellular CO2 concentration (Ci), and transpirationrate (Tr) were measured by a Li-Cor 6800 portable photosynthesis system (Li-Cor Inc., Lincoln, NE, USA). The measurements were carried out with a light intensity of 200 μmol m−2 s−1, an airflow of 700 μmol s−1 through the sample chamber, and a CO2 concentration at 400 μmol mol−1 in the sample chamber. Chlorophyll content was measured according to Lichtenthaler and Welburn (Citation16) at 645, and 663 nm using a spectrophotometer (UV-1800, Shimadzu, Kyoto, Japan).
2.7 Statistical analyses
The data were expressed as mean ± standard deviation (n = 4). Statistical significance of differences among treatments was determined using one-way analysis of variance and covariance (ANOVA), followed by Turkey’s pair-wise comparisons at a significance level of 0.05.
3. Results and discussion
3.1 Copper fractionation in the soil
The fractionations of copper in the soil after harvest are shown in . All CuO treatments underwent dissolution releasing soluble copper, but very limited (<0.05% of total Cu applied) was found as Cu ions for nCuO or μCuO. nCuO released 47.4% and 76.8% more Cu ions in the soil than μCuO, at 200 and 400 mg/kg, respectively. In addition, the DTPA-extractable Cu in the soil amended with nCuO was 53.2% and 70.1% higher at 200 and 400 mg/kg than that of μCuO, respectively. Results showed that nCuO behaved differently from μCuO in the soil with a higher release of Cu ions over time and higher potential bioavailability. CaCl2 extractable Cu ions in soil pore water are considered ”readily available”, while DTPA extracts the ”labile” fraction including dissolved Cu in soil pore water and the Cu2+ associated with soil solid phases, which is so-called ”potentially available” fraction [Citation13]. By providing a continuous release of Cu, nCuO is a promising candidate for agrochemical use.
Table 1. Copper fractions in soil amended with CuO nanoparticles (nCuO) or microparticles (μCuO) at 0 (control), 200, and 400 mg/kg. Values represent means ± standard after lettuce cultivation for 60 days. Different letters among columns indicate statistically significant differences at p ≤ 0.05.
3.2 Copper accumulation in lettuce
Copper concentrations in roots and shoots of harvest lettuces applied with nCuO/μCuO are shown in . All CuO treatments increased root Cu by 67.7–128.0% when compared with control, with 85.7, 157.7, and 195.4 mg/kg for control, 200, and 400 mg/kg, respectively. The trend is consistent with that of CaCl2-extractable and DTPA-extractable copper in soil (). This indicates that, for nCuO, uptake of Cu depends on the released Cu ions in soils [Citation17]. However, there was no such trend for μCuO treatments, because there was no significant difference between 200 and 400 mg/kg in released Cu ions (). In addition, CuO treatments increased Cu concentrations in shoots (by 3.6–13.8%) compared with control, except for μCuO at 200 mg/kg (). But there was no significant difference between CuO treatments at 200 and 400 mg/kg in shoot Cu, although higher CuO in soil led to more copper in roots. However, compared with μCuO, nCuO showed its improvement in nutrient uptake efficiency as a nano-enabled fertilizer. In addition to higher dissolution rate and solubility in soil, higher reactivity and more reaction sites of nanoparticles than bulks may explain their different accumulations and transformations in plants [Citation18].
Figure 1. Copper concentrations in shoots and roots of lettuce plants grown for 60 days in soil amended with CuO nanoparticles (nCuO) or microparticles (μCuO) at 0 (control), 200 and 400 mg/kg. Data are means of four replicates ± standard deviation. Different letters among columns indicate statically significant differences at p ≤ 0.05.
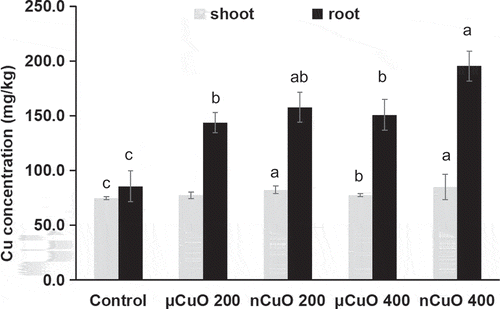
3.3 Biomass, MDA, and ROS production
shows the biomass production of harvested lettuce. CuO treatments caused no significant change in root biomass except for nCuO at 200 mg/kg. It seems that the reduction in root exposed to nCuO at 200 mg/kg was not associated with Cu ion release or bioavailable Cu in the soil. However, nCuO treatments increased shoot biomass by 16.3–19.1%, when compared to control. Dimpka et al. [Citation19], also reported an increased wheat biomass when exposed to nCuO but no such increase was observed when exposed to bulk CuO. Besides, such different effects on biomass were thought associated with the particle size rather than Cu ions releasing, because there were similar Cu ions released by CuO bulk and nCuO in the conditions. However, in our study conditions, nCuO had significantly higher release than μCuO, so the key role of releasing ions cannot be excluded. The size-dependent physiochemical properties of nanoparticles in soils, including higher release of ions and higher bioavailability than bulk, boost their potential to maximize crop production [Citation20].
Figure 2. Biomass (dry weight (DW)) of lettuce roots and shoots harvested after 60 days grown in soil amended with CuO nanoparticles (nCuO) or microparticles (μCuO) at 0 (control), 200 and 400 mg/kg. Data are means of four replicates ± standard deviation. Different letters among columns indicate statistically significant differences at p ≤ 0.05.
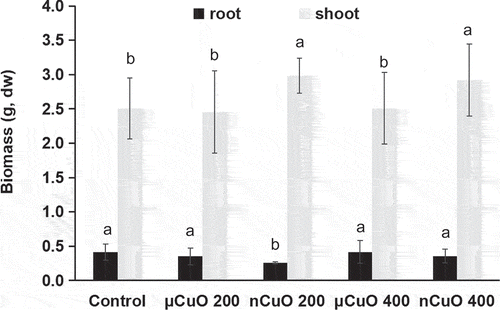
shows MDA and ROS produced in lettuce leaves. There was no significant change found in either MDA contents or relative ROS, indicating limited oxidative stress caused by the CuO treatments. Previous studies have shown that nCuO at different concentrations can increase the ROS production and MDA contents [Citation21]. However, such studies were carried out in hydroponic conditions with high dose applied. In our study, the CuO samples were applied according to Environmental Quality Standard for Soils of China (GB15618–1995). Results indicate that nCuO can be used under control as a safer fertilizer in agriculture with low toxicity comparing to copper salts such as CuSO4.
Table 2. Chlorophyll (Chl, mg/g fresh weight), malondialdehyde (MDA, nmol/mg fresh weight), and relative reactive oxygen species (ROS) level (%) in lettuce leaves amended with CuO nanoparticles (nCuO) or microparticles (μCuO) at 0 (control), 200, and 400 mg/kg. Values represent means ± standard after lettuce cultivation for 60 days. Different letters among columns indicate statistically significant differences at p ≤ 0.05.
3.4 Photosynthesis index
shows chlorophyll contents in lettuce leaves. No significant change was found in Chl a content caused by the CuO treatments. Only Chl b in lettuce exposed to nCuO at 200 mg/kg decreased when compared with control. No significant change was found in intercellular CO2 concentration (Ci; ) or photosynthetic rate (Pn; ) in lettuce leaves exposed to the CuO treatments. However, the exposures to nCuO at 200 and 400 mg/kg resulted in increased transpiration rate (Tr; ), and significantly higher stomatal conductance (Gs; ) compared with the control or μCuO treatments. Hence, increased biomass by nCuO can be explained by its modification in plant photosynthesis system. In previous studies, heavy metals of excessive dose can damage photosynthesis in terms of chlorophyll degradation []. Cao et al. [Citation22], also reported that excessive Cu (120 μM as CuSO4) can severely reduce chlorophyll pigments, resulting in lower Gs and Pn. As a micronutrient, Cu at lower critical concentration is required to improve the plant development [Citation24]. Our study embraces the argument that when applied at reasonable doses in actual soil conditions, bioavailability may be more important than other size-dependent properties of nanoparticles [Citation25].
Figure 3. Photosynthetic rate (A; Pn; µmol m−2 s−1), intercellular CO2 concentration (B; Ci; µmol mol−1), transpiration rate (C; Tr; mmol m−2 s−1), and stomatal conductance (D; Gs; mmol m−2 s−1) in leaves of lettuce plants exposed to CuO nanoparticles (nCuO) or microparticles (μCuO) at 0 (control), 50, 100 and 200 mg/kg for 60 days. Data are means of four replicates ± standard deviation. Different letters among columns indicate statistically significant differences at p ≤ 0.05.
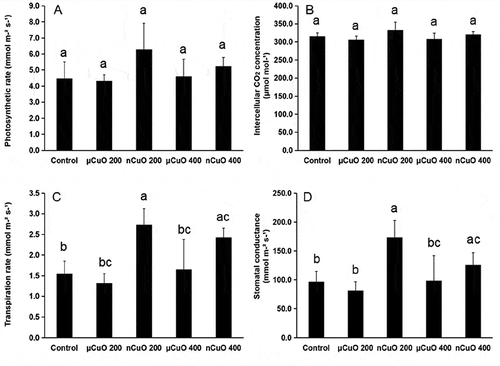
4. Conclusions
This study compared the effects of copper oxide nanoparticles and microparticles in lettuce plants. Results show that nCuO behaved differently from μCuO in the soil with a higher release of Cu ions and potential bioavailability. nCuO is a promising candidate for agrochemical fertilizer to increase plant production. Thus, nano-enabled slow-release fertilizers can be designed with low toxicity which can last over years without reapplication. More studies with different types of soils and edible plants are needed to assess the nutrient effects of nanoparticles to promote their applications in agriculture.
Disclosure statement
No potential conflict of interest was reported by the authors.
Additional information
Funding
References
- Fageria NK, Baligar VC, Clark RB. Physiology of crop production. Binghamton New York, U.S.A.: Food Products Press; 2006.
- Krupinsky JM, Bailey KL, McMullen MP, et al. Managing plant disease risk in diversified cropping systems. Agron J. 2002;94(2):198−209.
- Alloway BJ, Tills AR. Copper deficiency in world crops. Outlook Agric. 1984;13(1):32−42.
- Nagajyoti PC, Lee KD, Sreekanth TVM. Heavy metals, occurrence and toxicity for plants: a review. Environ Chem Lett. 2010;8(3):199−216.
- Rooney CP, Zhao F, McGrath SP. Soil factors controlling the expression of copper toxicity to plants in a wide range of European soils. Environ Toxicol Chem. 2006;25(3):726−732.
- Liu R, Lal R. Potentials of engineered nanoparticles as fertilizers for increasing agronomic productions. Sci Total Environ. 2015;514:131–139.
- Tegenaw A, Tolaymat T, Al-Abed S, et al. Characterization and potential environmental implications of select Cu-based fungicides and bactericides employed in U.S. markets. Environ Sci Technol. 2015;49(3):1294−302.
- Lee WM, An YJ, Yoon H, et al. Toxicity and bioavailability of copper nanoparticles to the terrestrial plants mung bean (Phaseolus radiatus) and wheat (Triticum aestivum): plant agar test for water -insoluble nanoparticles. Environ Toxicol Chem. 2008;27:1915–1921.
- Gao X, Spielman-Sun E, Rodrigues SM, et al. Time and nanoparticle concentration affect the extractability of Cu from CuO NP-amended soil. Environ Sci Technol. 2017;51(4):2226–2234.
- Brennan RF, Gartrell JW, Robson AD. Reactions of copper with soil affecting its availability to plants. I. Effect of soil type and time. Aust J Soil Res. 1980;18(4):447.
- Hong J, Rico CM, Zhao L, et al. Toxic effects of copper-based nanoparticles or compounds to lettuce (Lactuca sativa) and alfalfa (Medicago sativa). Environ Sci Process Impacts. 2015;17:177–185.
- Ochoa L, Zuverza-Mena N, Medina-Velo IA, et al. Copper oxide nanoparticles and bulk copper oxide, combined with indole-3-acetic acid, alter aluminum, boron, and iron in pisum sativum seeds. Sci Total Environ. 2018;634:1238−1245.
- Gao X, Avellan A, Laughton S, et al. Cuo nanoparticle dissolution and toxicity to wheat (Triticum aestivum) in rhizosphere soil. Environ Sci Technol. 2018;52(5):2888–2897.
- Ohkawa H, Ohishi N, Yagi K. Assay for lipid peroxides in animal tissues by thiobarbituric acid reaction. Anal Biochem. 1979;95(2):351−358.
- Dai Y, Wang Z, Zhao J, et al. Interaction of cuo nanoparticles with plant cells: internalization, oxidative stress, electron transport chain disruption, and toxicogenomic responses. Environ Sci Nano. 2018;5:2269–2281.
- Lichtenthaler HK, Welburn AR. Determination of total carotenoids and chlorophyll a and b leaf extracts in different solvents. Biochem Soc Trans. 1983;603:591–592.
- Ando Y, Nagata S, Yanagisawa S, et al. Copper in xylem and phloem saps from rice (Oryza sativa): the effect of moderate copper concentrations in the growth medium on the accumulation of five essential metals and a speciation analysis of copper-containing compounds. Funct Plant Biol. 2013;40:89–100.
- Peng C, Xu C, Liu Q, et al. Fate and transformation of cuo nanoparticles in the soil-rice system during the life cycle of rice plants. Environ Sci Technol. 2017;51(9):4907−4917.
- Dimkpa CO, Mclean JE, Latta DE, et al. CuO and ZnO nanoparticles: phytotoxicity, metal speciation, and induction of oxidative stress in sand-grown wheat. J Nanopart Res. 2012;14(9):1−15.
- Apodaca SA, Medina-Velo IA, Lazarski AC, et al. Different forms of copper and kinetin impacted element accumulation and macromolecule contents in kidney bean (Phaseolus vulgaris) seeds. Sci Total Environ. 2018;636:1534–1540.
- Du WC, Tan WJ, Peralta-Videa JR, et al. Interaction of metal oxide nanoparticles with higher terrestrial plants: physiological and biochemical aspects. Plant Physiol Bioch. 2017;110:210−225.
- Cao Y, Zhang Y, Ma C, et al. Growth, physiological responses, and copper accumulation in seven willow species exposed to Cu-a hydroponic experiment. Environ Sci Pollut Re. 2018;25:19875–19886.
- Dhindsa RS, Dhinsa PP, Thorpe TA. Leaf senescence correlated with increased levels of membrane permeability and lipid peroxidation and decreased levels of superoxide dismutase and catalase. J Exp Bot. 1980;32:127−132.
- Apodaca SA, Tan W, Dominguez OE, et al. Physiological and biochemical effects of nanoparticulate copper, bulk copper, copper chloride, and kinetin in kidney bean (Phaseolus vulgaris) plants. Sci Total Environ. 2017;599–600:2085–2094.
- Qiu H, Smolders E. Nanospecific phytotoxicity of Cuo nanoparticles in soils disappeared when bioavailability factors were considered. Environ Sci Technol. 2017;51(20):11976–11985.