ABSTRACT
The objective of this study was to investigate the bioavailability of iodine (I) after applying different soil amendments. The effectiveness of organic and inorganic I sources on I concentration of mint was compared. Soil was amended with sawdust, rice husk, charcoal, wood ash and gypsum at the rate 10 t ha-1. Results showed that the use of soil amendment significantly enhanced mint biomass. The biomass yield among amendments was in the order of charcoal > wood ash > sawdust > rice husk > gypsum > no-amendment. Amendments also significantly increased I concentration in plants. Iodine concentrations in mint shoot were higher in wood ash and charcoal than other treatments. Iodine contents in plants were higher with inorganic iodine than organic source. Iodine concentrations in shoot were associated with soil CEC and organic matter. This study indicated that a suitable soil amendment can be used for better I bioavailability.
KEYWORDS:
1. Introduction
Iodine (I) is an important element for the proper thyroid functioning, development of nervous tissues and brain development. It is also involved in the mechanism of resistance to ionizing radiation [Citation1,Citation2]. Despite the broad application of domestic salt iodization, pathological symptoms related to inadequate I intake were diagnosed in 30–38% of the population [Citation3]. Iodine deficiency is still a major problem especially in the third world countries and with almost 1 billion people are at the risk of iodine deficiency [Citation4]. Iodized salt is currently the most prevalent food supplement to control Iodine deficiency disorders (IDD) [Citation5]. However, inorganic I (KI or KIO3) from salt was reported less bioavailable than I from plant (or animal) sources [Citation6]. Iodine was reported to be readily volatilized during the process of production, storage and transport of iodized salt [Citation7].
Biofortification of crops with I was proposed as an alternative method of introduction of this element into the food chain [Citation3]. Before its promotion, optimal agro-technical methods of I application need to be developed, which is particularly important as this element is not a plant nutrient. Numerous studies on the bio-fortification of I have been conducted in soil-less and hydroponic systems. With these methods, plants take up and accumulate I to a considerably greater extent than when grown in soil. Irrespective of the chemical form of I, it is strongly fixed in the soil after its application. Mineral elements are responsible for I sorption, e.g. Fe and Al sesquioxides – essentially with pH <6.0–6.9 [Citation8] and Cu/Al or Cu/Cr hydroxides [Citation9]. A substantial amount of I in soils is fixed with soil organic matter [Citation10]. Iodine fixation occurs through the formation of covalent bonds with aromatic compounds containing double bonds (e.g. phenolic and polyphenolic compounds), which are part of humified organic matter, including fulvic acids and humins [Citation11]. On the basis of such a mechanism, I is strongly fixed with soil humus [Citation12]. It needs to be underlined that I desorption in soil is a slow process [Citation13]. Smoleń et al. [Citation10] reported that plant fertigation with KI or KIO3 solution is a more efficient method for I biofortification of spinach than pre-sowing introduction of these compounds into the soil.
Eighty percent of I in the human body and animal original comes from edible vegetable food under natural condition [Citation14], and the bioavailability of I in food can be achieved up to 99%. Due to the fact that I in edible plant food originates from soil, the background concentration of I in soil as well as its bioavailability determines whether the consumed I can meet the need. Iodine concentration in soil was estimated to be between 1.55 and 12.93 mg kg−1 in China [Citation15]. Generally, I is one of the less abundant elements in the environment and soils contain 3 mg kg−1 of total iodine [Citation16]. Iodine concentrations in soils and vegetables in inland are usually too low to meet the need of the human body. Crops grown in iodine deficient soils are low in iodine concentration and man and animals consuming these crops become deficient in iodine. Therefore, a relationship between nutrient deficiencies in soils, crops, animals, food and human nutrition exists. Apparently, to elevate the concentration of I in soil and thus enrich I in edible plant food using I fertilizers is an attractive approach for producing I supplementation and eliminating IDD because of its natural prevention of overdose and readily fitting in local food chain. Weng et al. [Citation13] reported that crop root can assimilate exogenous I effectively in some concentrations after hydroponics and pot studies. Research reports on the uptake of I by edible plants have been poorly documented. The aim of this research was, therefore, to investigate the influence of organic and inorganic source of I on its bioavailability to mint (Mentha longifolia L.) after application of different soil amendments.
2. Materials and methods
2.1. Soil sampling and analysis
For this experiment, soil samples were collected from local agricultural fields of Abbottabad, Pakistan. The samples were air-dried, sieved via a 2-mm sieve and characterized for their physicochemical properties (pH, electrical conductivity [EC], texture [sand, silt and clay], total C, Ca, Mg and K) (). Soil texture was determined using pipette method [Citation17]. Total C in soil was determined by dry combustion [Citation18]. The pH of soil suspension with soil:water ratio of 1:5 was determined using a pH meter (Model: HANNA HI 8520). EC in the same soil suspension was measured with an EC meter (Model: 4320 JENWAY). Calcium (Ca), magnesium (Mg) and potassium (K) were leached down from the soil using ammonium acetate (NH4OAc) (pH 7) and determined using an atomic absorption spectrophotometer (Model: AAnalyst 700, Perkin Elmer) ().
Table 1. Relevant properties of soil used for the experiment.
In the spring season of 2018, mint was cultivated in the Polyvinyl chloride (PVC) column (size: 10 cm diameter and 60 cm height). The columns were packed with 5 kg of soil. Amendments namely sawdust, rice husk, charcoal, wood ash and gypsum were applied to the soil at the rate of 10 t ha−1 (based on 2 million kg soil per plow layer in ha). There was also a control treatment, where there was no amendment as well as no iodine treatment. Amendments were mixed on the top soil (0–20 cm) of the column. Iodine was applied in the form of inorganic source as potassium iodide (KI) and organic source (extracted from alfalfa [Medicago sativa] and dulse [Rhodymenia palmata]). This product was commercially available. The iodine sources were fertigated to soil at the rate of 50 mg kg−1. Mint plants (M. longifolia) were grown for 8 weeks. Mint was selected due to its common use in the daily food. Each replicate consisted of six plants and irrigated twice the daily pan evaporation with distilled water. Prior to the cultivation, soil was fertilized with urea at the rate of 200 kg ha−1. The experiment was a factorial (6 × 2) (6 soil amendments and 2 sources of iodine) resulting in 12 experimental units, arranged into a completely randomized design replicated three times. After harvesting, plants were washed thoroughly with tap water and then distilled water. Plants shoots were cut into small pieces, dried for 48 h at 60°C and then determined shoot dry biomass.
2.2. Plant analyses
The chemical composition of the shoot was determined and the dried samples were milled and digested in a mixture (3:1) of nitric acid (HNO3) and perchloric acid (HClO4) [Citation19]. Iodine was assessed by using Kesari et al. [Citation20] method by taking 0.5 g of dry mass, ground and sieved. Double acid digestion was carried out at the temperature of 120°C. It was then diluted and filtered and reagent such as 0.5 mL of bromine water, one drop of formic acid was added and mixed thoroughly. After mixing, 1 mL of leuco crystal and 1 mL of 0.1% of KI solution were added and pH was maintained between 4.4 and 5.4 by using 0.01 M NaOH. Samples were diluted to 25 mL and left for 30 min and I concentration was determined colorimetrically using a UV spectrophotometer (Model: LI-UV-7000) at 591 nm.
2.3. Soil analyses
Postharvest soil was also sampled from column at three depths (i.e. 0–20, 20–40, 40–60 cm) and analyzed for chemical composition. pH and EC of the soil were determined. Iodine concentration was determined using the method of Kesari et al. [Citation20]. Iodine translocation factor (TF) was calculated by the formula: TF = I concentration in shoot (µg kg−1)/I concentration in soil (0–20 cm).
2.4. Data analyses
Data were statistically analyzed using Statview software [Citation21] and means were separated using least significant difference test at 5% level of probability. Relationship of dry biomass and I concentration of mint versus organic matter and cation exchange capacity (CEC) of soil was also determined.
3. Results and discussion
3.1. Dry biomass
Mint growth was significantly affected by the soil amendments (). The application of amendments improved biomass yield of both mint irrespective of the iodine (I) treatments. Soil amendments differed for biomass production in the order of charcoal > wood ash > sawdust > rice husk > gypsum. However, I sources did not differ for biomass production of mint. The application of material like charcoal, wood ash, sawdust and rice husk gave superior dry matter yield in mint possibly due to the supply of essential plant nutrients. The increased biomass apparently resulted from the addition of some essential nutrients to the soil after decomposition of waste materials. Soil amendment enhanced mint biomass by 23% in sawdust, 22% in rice husk, 38% in charcoal and 36% in wood ash treated soil after supplementation with KI salt.
3.2. Shoot iodine concentration
Iodine (I) contents in the plants were governed by the type of amendment applied. Amendments also significantly increased I concentration in plants as compared to the no amendment. The incorporation of wood ash and charcoal showed a remarkable increase in I concentration over the control. Iodine concentrations in mint shoot were apparently higher in wood ash and charcoal treatments followed by sawdust and rice husk. Gypsum treatment gave the lower I concentration. As expected, inorganic form of I application increased I concentration in mint plants than its organic source. Mint plants grown in wood ash soil showed higher I concentration in the shoot (420 µg kg−1). Shoot of mint fertigated with KI in charcoal and sawdust-amended soil fortified 336 and 325 µg kg−1 of I, respectively. Iodine concentrations in shoot were increased by 73%, 70%, 74% and 79% in sawdust, rice husk, charcoal and wood ash, respectively, after KI application as compared to control (where there was no KI treatment and no soil amendment). Among the soil amendments, wood ash after supplementation with KI showed higher I bio-fortification in mint plants. Sawdust, charcoal and rice husk-amended soil exhibited significantly higher I uptake than control. There is a consensus that I content of soils has been governed not only by its supply but also by the ability of the soil to retain the added I in the soil column. Changes in I concentrations in plants could be related to soil chemical composition. Higher concentration of I in plants may be attributed to the addition of organic matter inputs from waste material in soil. Uptake of I by mint species depended on the soil parameters. These properties have a significant impact on the composition of mint shoot. Plant biomass found linearly related with the CEC (R2 = 0.65) and organic matter of soil (R2 = 0.56) (). Iodine concentrations in mint shoot were also found dependent on CEC (R2 = 0.67) and organic matter of soil (R2 = 0.52) ().
Figure 2. Relationship of dry biomass of mint versus organic matter and cation exchange capacity (CEC) of soil.
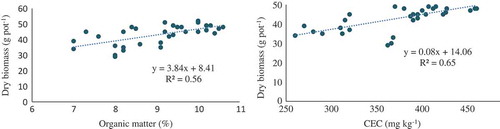
TF of mint plant fertilized with KI showed significantly higher values than organic I. The TF values for mint plant applied with KI were 1.15, 1.29, 1.24, 1.35 and 0.84 for sawdust, rice husk, charcoal, wood ash and gypsum, respectively. These values were slightly lower in plants supplemented with organic I.
3.3. Shoot mineral composition
Concentrations of elements in plant tissue were the function of soil amendments (). Mixing of soils with selected amendment altered the availability of I. Soil amendment increased shoot contents of elements, irrespective of I treatments. The contents of these elements in the mint shoot varied in the order of Ca > P > K > Mg > Na > Zn > Fe. Calcium contents in mint shoot increased by 28%, 16%, 37% and 40% in the rice husk, charcoal, wood ash and gypsum treatments, respectively. Sawdust could not enhance Ca in mint plants. When compared with the control, the amendment application enhanced Mg contents in mint from 15 to 21 mg kg−1 in rice husk, 18 mg kg−1 in charcoal, 24.8 mg kg−1 in wood ash and 25.5 mg kg−1 in gypsum treatment. Organic I reduced the concentration of Mg in plants. Phosphorus concentrations in the mint shoots were 20, 31, 38, 31 and 42 mg kg−1 in control, sawdust, rice husk, charcoal and wood ash treatments, respectively. Gypsum treatment reduced the concentration of P in mint shoot. The application of these amendments relatively improved K uptake by mint. Mineral composition in mint plants could be associated with the physicochemical changes that have been occurred in soil due to the application of soil amendment. It has been reported that soil properties like CEC, organic matter and clay minerals affected I adsorption and bioavailability [Citation22].
Table 2. Mineral composition of mint shoot as affected by soil amendment and source of iodine.
3.4. Postharvest soil composition
The application of amendment in soil significantly affected the extractability of I from postharvest soil (). Chemical constituents in soil layers have been influenced by soil amendments applied. The CEC and organic matter of soil were positively associated with the amendment. Iodine content in the top soil layer (0–20 cm) was found less than the lower soil layer. This could be associated with the plant uptake or leaching of I from the top soil layer. Soil amendments like gypsum significantly raised the pH. The value of EC was found higher in the upper soil column. CEC was enhanced with the application of soil amendment especially in the upper soil surface, where the amendments were mixed in the soil. Iodine content in the postharvest soil has been observed depending on the ability of the amendment to retain the added I. All amendments substantially retained I contents in soil. Higher I concentration and lower EC, organic matter (OM) and pH values were observed in the sawdust-amended soil supplemented with KI salt. Wood ash and sawdust-amended soil supplemented with KI retained higher I in the upper soil than other amendments. Generally, I concentrations were found higher in the lower zone of the soil column. This could be attributed to the plant uptake and leaching of I from the upper soil after the process of irrigation. The least I retention was shown by gypsum-amended soil. The higher concentration of I in the soil column indicated that the application of material like charcoal, wood ash, rice husk and sawdust can viably be used to reduce I losses from soil. Organic source of I gave higher concentration of I in the postharvest soil than KI, irrespective of the soil amendment. Johanson [Citation16] reported the highest amount of I loss from soils with pH below 5 and organic content below 3%. Factors that decrease the sorption of I in soils include anoxic redox conditions, increasing salinity and the concentration of competing anions, increasing pH and clay mineral content [Citation22,Citation23]. Knowles et al. [Citation24] reported reduced nitrate leaching from biochar plus biosolid-amended soils than control treatments. Earlier researches showed that microorganism present in the soil readily consumes the organic I as compared to inorganic I. Dai et al. [Citation8] found a positive relationship of I sorption and CEC of soil. Chlorine fixation by soil organic matter has also been reported [Citation25].
Table 3. Chemical composition of postharvest soil sampled from three layers of column applied with amendments.
4. Conclusions
This study concluded that soil amendments significantly increased I concentration in mint plants as compared to control. Iodine concentrations in mint shoot were higher with wood ash and charcoal application followed by sawdust and rice husk. Iodine fertigation in the form of KI particularly increased I concentration in plant tissue. Plant biomass and I concentration in shoot were found associated with the CEC and organic matter of soil. This study indicated that a suitable soil amendment could prove to be a better management option for I biofortification.
Acknowledgments
We highly appreciate and acknowledge the funding of COMSATS University Islamabad for the continuation of this research study.
Disclosure statement
No potential conflict of interest was reported by the authors.
References
- Charlton KE, Gemming L, Yeatman H, et al. Suboptimal iodine status of Australian pregnant women reflects poor knowledge and practices related to iodine nutrition. Nutrition. 2010;26(10):963–968.
- Melse-Boonstra A, Jaiswal N. Iodine deficiency in pregnancy, infancy and childhood and its consequences for brain development. Best Pract Res Clin Endocrinol Metab. 2010;24(1):29–38.
- White PJ, Broadley MR. Biofortification of crops with seven mineral elements often lacking in human diets–iron, zinc, copper, calcium, magnesium, selenium and iodine. New Phytol. 2009;182(1):49–84.
- Zimmermann MB, Andersson M. Assessment of iodine nutrition in populations: past, present, and future. Nutr Rev. 2012;70:553–570.
- Vejbjerg P, Knudsen N, Perrild H, et al. Effect of a mandatory iodization program on thyroid gland volume based on individuals’ age, gender, and preceding severity of dietary iodine deficiency: a prospective, population-based study. J Clin Endocrinol Metab. 2007;92(4):1397–1401.
- Zheng BS, Wang BB, Zhu GW. Environmental geochemistry of iodine in atmosphere and plant-review and a hypothesis. Earth Sci Front. 2001;8(2):359–366.
- Longvah T, Toteja GS, Bulliyya G, et al. Stability of added iodine in different Indian cooking processes. Food Chem. 2012;130(4):953–959.
- Dai JL, Zhang M, Hu QH, et al. Adsorption and desorption of iodine by various Chinese soils: II. Iodide and iodate. Geoderma. 2009;153(1–2):130–135.
- Pless JD, Chwirka JB, Krumhansl JL. Iodine sequestration using delafossites and layered hydroxides. Environ Chem Lett. 2007;5(2):85–89.
- Smoleń S, Ledwożyw-Smoleń I, Sady W. The role of exogenous humic and fulvic acids in iodine biofortification in spinach (Spinacia oleracea L.). Plant Soil. 2016;402(1–2):129–143.
- Unno Y, Tsukada H, Takeda A, et al. Soil-soil solution distribution coefficient of soil organic matter is a key factor for that of radioiodide in surface and subsurface soils. J Environ Radioact. 2017;169:131–136.
- Söderlund M, Virkanen J, Aromaa H, et al. Sorption and speciation of iodine in boreal forest soil. J Radioanal Nucl Chem. 2017;311(1):549–564.21.
- Weng H, Hong C, Xia T, et al. Iodine biofortification of vegetable plants- An innovative method for iodine supplementation. Chin Sci Bull. 2013;58(17):2066–2072.
- DeLong GR, Leslie PW, Wang SH, et al. Agriculture: the real nexus for enhancing bioavailable micronutrients in food crops. J Trace Elem Med Biol. 2005;18(4):299–307.
- Welch RM, Graham RD. Agriculture: the real nexus for enhancing bioavailable micronutrients in food crops. J Trace Elem Med Biol. 2005;18(4):299–307.
- Johanson KJ. Iodine in soil-A technical report TR-00-21, Svensk Kärnbränslehantering AB. Swedish Nuclear Fuel and Waste Management Co Box 5864 SE-102 40. Stockholm Sweden; 2003.
- Gee GW, Bauder JW. Particle-size analysis. In: Klute A, editor. Methods of soil analysis, part 1. Physical and mineralogical methods, agronomy monograph No. 9. 2nd ed. Madison, WI: American Society of Agronomy/Soil Science Society of America; 1986. p. 383–411.
- Nelson DW, Sommers LE. Total carbon, organic carbon and organic matter. In: Page AL, Miller RH, Keeney DR, editors. Methods of soil analysis. Part 2. Chemical and microbiological properties. Madison, WI, USA: ASA, SSSA; 1982. p. 539–580.
- Miller RO. Nitric-perchloric acid wet digestion in an open vessel. In: Kalra Y., editor. Handbook of reference methods for plant analysis. Washington, DC: CRC Press. 1998;57-61.
- Kesari R, Rastogi R, Gupta VK. A simple and sensitive spectrophotometric method for the determination of iodine in environmental samples. Chem Anal. 1998;43(2):201–207.
- SAS. StatView reference. third ed. Cary, NY, USA: SAS Institute Inc.; 1999.
- Yoshida S, Muramatsu Y, Uchida S. Studies on the sorption of I-(Iodide) and IO3-(Iodate) onto Andosols. Water Air Soil Pollut. 1992;63:321–329.
- Muramatsu Y, Yoshida S. Effects of microorganisms on the fate of iodine in the soil environment. Geomicrobiol J. 1999;16:85–93.
- Knowles OA, Robinson BH, Contangelo A, et al. Biochar for the mitigation of nitrate leaching from soil amended with biosolids. Sci Total Environ. 2011;409:3206–3210.
- Öberg G, Sandén P. Retention of chloride in soil and cycling of organic matter‐bound chlorine. Hydrol Process. 2005;19(11):2123–2136.