ABSTRACT
The Cd(II) adsorption of kiwi biochar functionalized with Mg-Fe (3:1) layered double hydroxides (LDH) was studied. And the biochar was got via liquid-phase deposition, by incorporating Mg-Fe layered double hydroxide (Mg-Fe LDH) particles into kiwi biochar (KB/LDH). The batch of sorption experiments were studied, including adsorption kinetics and isotherm and the effects of pH, ion strength (IS) and co-existing ions. The KB/LDH composite showed a strong sorption ability to Cd(II) in aqueous solutions with the adsorption capacity of 25.6 mg g−1, compared with pristine biochar of 1.78 mg g−1. The adsorption of Cd(II) on KB/LDH composite increased with the decreasing of IS, decreased first and then grew larger with the increase of pH and was observed irrespective of the co-existence of K+, Cl− and NO3−. To sum up, the KB/LDH can be potentially used as an alternative adsorbent to amend the water and soil contaminated by Cd(II).
1. Introduction
A number of conventional treatment methods, such as chemical precipitation [Citation1], ultrafiltration [Citation2],and electrodialysis [Citation3], were developed for the removal of heavy metals in contaminated water. Compared with other reported methods, adsorption technology costs less money in recycling for reuse and needs fewer processes in treatment, which has been widely studied and applied in removal of mixed heavy metal pollutants in the past decades [Citation4]. One recommended approach is to combine different adsorption materials to get more efficient adsorbents so that it can reduce competitions for free sorption sites [Citation5,Citation6].
Biochar (BC) is biomass-derived black C that has been recently recognized as a multifunctional material related to C sequestration, metal immobilization and fertilization in soils [Citation7]. Biochar is produced by thermal decomposition of biomass under a negligible or limited supply of oxygen [Citation8]. Various types of biomass including poultry litter [Citation9], dairy manure [Citation10], sewage sludge [Citation11] and paper sludge [Citation12] have been used to produce BCs. The diverse natured BCs are being commonly applied to soils as conditioners, and has received extensive attention for the removal of contaminants from wastewater as a renewable, low cost and sustainable adsorbent [Citation13]. However, kiwi biochar have not been investigated in the removal of water environment with heavy metal ions. kiwi fruit is the plant which greenish-brown in appearance, covered with thick fluff, with bright green flesh and a row of black seeds of its interior. And Shaanxi province is the largest kiwi fruit growing province in China. Nevertheless, Kiwi fruit produce a lot of waste during planting, such as kiwi fruit branches and leaves. If this waste is taken good use, let it become Kiwi biochar which deal with the heavy metals pollution in water and soil, and the environment will be protected greatly. But the restrictions on interfacial mechanism and sorption capacities are unclear for using the kiwi biochar widely.
LDHs are a class of ionic lamellar compounds made up of brucite-like layers with an interlayer region containing easily exchangeable intercalated anions and solvation molecules with hydrogen bonds, which show the diversity and functionality for various contaminants [Citation14]. Such structures and characteristics provide LDHs wide applications, such as energy storage [Citation15] and environmentally friendly materials which are used for removing toxic ions from polluted water and soil, and has lately been studied for the removal of nitrate [Citation16–Citation18], phosphate [Citation19,Citation20] and some heavy metals [Citation21], however, the LDH has some shortcomings such as difficult regeneration processes and formation of aggregates which restrict its‘ use widely [Citation22]. According to these features of LDH, we can combine LDH with Kiwi biochar to take advantages of two different materials and enhance specific adsorption properties for Cd(II) contaminants. During the adsorption process, Kiwi biochar can serve as an effective matrix to support LDH flakes, producing a KB/LDH assembly for a potential adsorbent to improve the ability of adsorption and prevent the aggregation of LDHs [Citation23]. Composite materials with colloidal and/or LDHs have been developed to maximize the sorption ability of LDHs to various aqueous contaminants [Citation24,Citation25], whereas little research has been conducted on the combining biochar with colloidal and/or LDHs for pollutant removal from contaminated water. Yu et al. [Citation21], found that heavy metals were successfully removed by Mg-Fe-LDH-RHA. But until to now, none of the previous studies have accurately studied the sorption ability and the influence factors of LDH-kiwi biochar composites to Cd(II) in aqueous solutions.
The object of this study is to evaluate the potential application and the influence factors of KB/LDH composite in the removal of Cd(II) from aqueous solutions. Biochar was derived from kiwi branch through slow pyrolysis and then modified with Mg-Fe LDH through liquid-phase deposition. Series of laboratory experiments were then conducted to characterize the physicochemical properties and adsorption ability of the Mg-Fe LDH modified biochar to Cd(II) under various conditions. The specific objects were as follows: (1) to synthesize and characterize KB/LDH composite; (2) to study the Cd(II) adsorption characteristics of the KB/LDH composite; and (3) to evaluate the efficiency and influence factors of the KB/LDH composite in the removal of Cd(II).
2. Experimental
2.1 Materials and chemicals
Cd(II) chloride hemipentahydrate (CdCl2 · 2.5H2O) was purchased from the Aladdin Industrial Corporation (Shanghai, China). Analytical grade magnesium chloride hexahydrate (MgCl2 · 6H2O), iron(III) chloride hexahydrate (FeCl3 · 6H2O), sodium hydroxide (NaOH) were purchased from Guangdong Guanghua Chemical Factory Company, Ltd. All experimental solutions were prepared using deionized (DI) water (18.2 MO), which was also used to rinse and clean the samples. Cd(II) standard solutions were prepared by dissolving CdCl2 · 2.5H2O in DI water.
2.2 Preparation of samples
Kiwi branch was collected from a kiwi grove in Yangling (108.1°E, 34.3°N), Shaanxi Province, China, for biochar production. The kiwi branches were separated from kiwi fruit and chopped into about 1 cm length, washed with DI water and dried at 120 °C overnight. Then, it was pyrolyzed at 500 °C under N2 flow conditions in a SG-GL1200K Vacuum tube furnace (Shanghai Institute of Optics and Fine mechanics, the Chinese Academy of Sciences, Shanghai, China) with a heating rate of approximately 9.5 °C min−1. The biochars produced were ground and passed through a 0.25–1 mm sieve and stored in air-tight plastic bags. Mg-Fe LDH deposited biochar composite was synthesized by the liquid-phase deposition method according to Zhang et al. [Citation19]. Briefly, the pH of 100 mL aqueous solution containing MgCl2 (0.03 mol), FeCl3(0.01 mol) and biochar (10.0 g) was adjusted to about 10.0 ± 0.1 using 1 mol/L NaOH solutions. The obtained slurry was aged for 3 days at 80 °C, then filtered and washed with DI water. KB/LDH sample was then obtained by drying the filtrate at 80 °C in an oven.
2.3 Adsorption kinetics
Adsorption kinetics of Cd(II) onto pristine biochar and KB/LDH were examined by mixing 0.02 g of the KB/LDH with 25 mL Cd(II) solutions of 50 mg L−1 in 50 mL digestion vessels (Environmental Express) at a room temperature (30 ± 2 °C). The pH and IS were then adjusted to 6.5 ± 0.2 and 0.01M NaCl, respectively, prior to the measurements of the adsorption kinetics. The vessels were then shaken at 200 rpm in a mechanical shaker. At appropriate time intervals (5 min-24 h for pristine biochar, 5 min-36 h for KB/LDH), the vessels were withdrawn and the mixtures were immediately filtered through 0.22 μm pore size nylon membrane filters (GE cellulose nylon membrane). The Cd(II) concentrations in the liquid phase sample were analyzed with Atomic Absorption Spectroscopy (Z-2000, Hitachi, Japan). Cd(II) concentrations in the aqueous and adsorbed phase were calculated based on the initial and final aqueous concentrations. Samples were studied in duplicates.
2.4 Adsorption isotherm
Adsorption isotherm of Cd(II) onto pristine biochar and KB/LDH were determined similarly by mixing 0.02 g KB/LDH with 25 mL Cd(II) solutions of different concentrations ranging from 0.5 to 100 mg L−1 in 50 mL digestion vessels. After pH and IS adjustment to 6.5 ± 0.2 and 0.01 M NaCl, the vessels were shaken in the mechanical shaker at 200 rpm for 24 h at room temperature (30 ± 2 °C), this time period having been previously determined by kinetic experiments as sufficient for adsorption equilibrium to be established. The samples were then withdrawn, and the mixtures were immediately filtered through 0.22 μm pore size nylon membrane filters to determine adsorbed Cd(II) concentrations by the same method. Cd(II) concentrations in the aqueous and adsorbed phase were calculated based on the initial and final aqueous concentrations. Samples were studied in duplicates. Following the experiments, the post-adsorption KB/LDH samples were collected, rinsed with DI water and dried at 80 °C in an oven for further characterizations.
2.5 Effect of pH, IS and coexisting anions
The effect of initial solution pH on Cd(II) removal was studied over a range of 3.5–7.5 (i.e., 3.5, 4.5, 5.5, 6.5 and 7.5). The effect of IS on Cd(II) removal was studied over a range of 0.001–0.1 M (i.e., 0.001, 0.005, 0.01, 0.05 and 0.1 M) by adding NaCl solutions. What is more, the effect of the common coexisting ions was also investigated by adding 0.01 M of KCl, NaCl, NH4Cl, CaCl2, or NaNO3 to the 25 mL Cd(II) solutions of 50 mg L−1 into separate 50 mL digestion vessels. The vessels were shaken in the mechanical shaker at 200 rpm for 24 h at room temperature (30 ± 2 °C), and the samples were then withdrawn and the mixtures were immediately filtered through 0.22 μm pore size nylon membrane filters. The same procedures were then used to determine aqueous and adsorbed Cd(II) concentrations which were calculated based on the initial and final aqueous concentrations. Samples were studied in duplicates.
2.6 Characterizations
The characterization experiment was conducted with the pristine biochar and selected pre- and post-adsorption KB/LDH composites. Brunauer-Emmett-Teller (BET) specific surface area of pristine biochar and KB/LDH were determined using a V-Sorb 2800P surface area and pore size analyzer (GAPP-spectrum, Beijing, China). X-ray diffraction (XRD) analysis was carried out to identify crystallographic structure using a computer-controlled X-ray diffractometer (D/RAPID II, Rigaku, Japan) equipped with a stepping motor and graphite crystal monochromator. Fourier transform infrared spectroscopy (FTIR) (Tensor27, Bruker, Germany) was performed to identify active functional groups responsible for sorption process. Scanning electron microscopy (SEM) coupled with dispersive Energy dispersive spectrometer (EDS) (S-4800, Hitachi Limited, Japan) was used to examine the morphology, types and content of component elements in microregion of materials, respectively. These characteristics of the pre-and post-sorption KB/LDH composites were compared with those of the pristine biochar [Citation26] to determine the adsorption mechanisms.
3. Results and discussion
3.1 Morphological structure of KB/LDH composite
Specific surface areas were 1.5 m2 g−1 for the pristine biochar and 31.2 m2 g−1 for KB/LDH. Thus, specific surface areas were considered as the key parameter in determining Cd(II) sorption onto pristine biochar and KB/LDH.
The morphology of the obtained sorbents was observed using SEM under different magnifications and mainly showed no regular or amorphous characteristics (.). These resulted from the grinding during sample preparation. The SEM images exhibited smooth surfaces of () the pristine biochar in contrast to the significant roughness on the surfaces of ()KB/LDH composites, which was indicative of the formation of LDH flakes on biochar matrix. Thus, the synergetic effect between LDH and biochar in enhancing Cd(II) adsorption () can be understood in a context that the biochar served as an effective matrix in ‘dispersing’ nanosized LDH during the synthesis, and thereby increasing active sorption sites of LDH for aqueous Cd(II). EDS showed a lot of Mg and Fe on the surface of the KB/LDH compared with pristine biochar, and it was further confirmed that the kiwi biochar was modified with Mg-Fe LDH successfully. Fe was not found on the pristine biochar and Cd was not found on the pre-sorption KB/LDH, which were attributed to the low content of Fe and Cd ().
Figure 1. SEM-EDS analysis of pristine biochar (a), pre-sorption KB/LDH (b) and post-sorption KB/LDH (c). XRD patterns (d) and FTIR spectra (e) of pristine biochar and pre- and post-sorption Cd-impregnated KB/LDH samples.
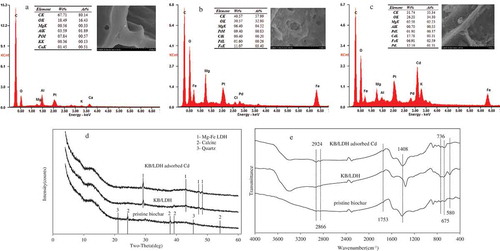
The XRD pattern in the range of 0–60° of the KB/LDH sample is shown in . The pattern shows diffraction peaks of both the LDH structure and the MgFe2O4 (magnesioferrite) spinel structure, which is supported by the results that some of the MgFe-LDH microcrystals were converted into spinel ferrite when the aging temperature was higher than 40 °C. The weaker peak intensity of the Calcite component compared to that of the LDH suggests that LDH was still the dominant component in the sample. This indicates that the sample was a composite of Mg-Fe LDH and Calcite. Some patterns of quartz were also found in the XRD due to the existence of Si in kiwi branches. Instead of these minerals, Mg-Fe LDH was found in KB/LDH both before and after Cd sorption (.). No crystal forms of adsorbed Cd were found, suggesting that Cd only reacted with the KB/LDH’s surface and sorption did not change its crystal structure or form a separate precipitate phase (.).
The differences of FT-IR spectra of the studied biochar samples were mainly focused on the wavenumber interval of 3500–500cm−1. Compared with pristine biochar, new peaks for the pre- and post-sorption KB/LDH samples appeared or strengthened at 3392, 2924–2866, 1753, 1408–1357 and 736–580 cm−1 (.). A peak of -NH2 stretching peak around 3392 cm−1, –COOH stretching peak around 1408–1357 cm−1 and -OH stretching peak around 736–580 cm−1 appeared or strengthened after Mg-Fe LDH impregnation, but weakened greatly after the Cd sorption (.). These peaks changed greatly, and black shifts occurred after the Mg-Fe LDH impregnation and the Cd sorption (.), confirming that Mg-Fe LDH played an important role in Cd sorption. The –CH3 stretching peak around 2924–2866 cm−1 and C=O stretching peak around 1753 cm−1 were similar among the pristine biochar and the pre- and post-sorption KB/LDH. Therefore, both Mg-Fe LDH impregnation and the Cd sorption altered the surface functional groups of the biochar.
3.2 Adsorption kinetics
The adsorption of Cd(II) onto pristine biochar and the KB/LDH composite increased smoothly over time at a room temperature (30 ± 2 °C) using the initial Cd(II) concentration (50 mg L−1), and reached equilibrium after 120 min and 1440 min, respectively, –). The initial high rate of Cd(II) uptake was probably due to the greater ion exchange ability of KB/LDH. The slow phase was perhaps associated with the pore network and surface of the biochar which favored the dispersion of KB/LDH flakes to efficiently increase their reaction with Cd(II). Mathematical models were used to simulate the experimental kinetics. In addition to the commonly used First-order model [Citation27] and Second-order models [Citation28], the Elovich model [Citation29] and Constant Double model [Citation30] were also tested and the equation constant fitted parameters of the adsorption characters are summarized in .
Table 1. Constants and correlation coefficient of kinetic models of Cd(II) adsorption on KB/LDH.
Figure 2. Adsorption kinetic data and modeling for Cd(II) onto pristine biochar (a) and KB/LDH (b), Internal diffusion for Cd(II) onto pristine biochar (c) and KB/LDH (d). IS = 0.01 M NaCl, pH = 6.5 ± 0.2 and T = 30 ± 2 °C.
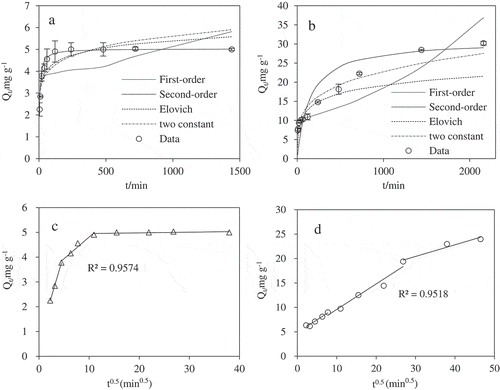
The experimental results of pristine biochar and KB/LDH were fitted to the kinetic models of Second-order model and Elovich defined in . And the Second-order model was the most suitable one in describing the adsorption kinetics of Cd(II) on pristine biochar and the KB/LDH composite as they had the highest R2 (R2 > 0.97), and closest match of Qe with the experimental results (5.03 and 30.96 mg g−1 respectively) (). Second-order model fitted better than other models, assuming that chemisorptions involving valency force were the rate-controlling step of sorption process through sharing or exchanging of electrons between sorbent and sorbate [Citation31]. Data also fitted well with Elovich and Constant Double models with relatively high correlation coefficients (), which confirmed the contribution of chemisorption and complexation with active functional groups (e.g. C=O and -OH) or the exchangeable H+ ions in sorption process [Citation32].
The kinetic behaviors of microporous sorbents showed that intraparticle surface diffusion was important to the adsorption process in previous studies [Citation33,Citation34]. The adsorption of Cd(II) onto pristine biochar and KB/LDH also showed diffusion limitation in this study. The pre-equilibrium Cd(II) adsorption showed a strong linear dependency (R2 > 0.95) on the square root of time ). This result suggested that intraparticle surface diffusion may play an important role in controlling the adsorption of Cd(II) onto the KB/LDH, and the possible reason is its abundance of mesopores.
3.3 Adsorption isotherms
With the maximum observed Cd(II) adsorption of greater than 25.6 mg g−1 (), the KB/LDH showed Cd(II) sorption ability to be superior to pristine biochar of 1.78 mg g−1(). Langmuir [Citation35], Freundlich [Citation36] and Temkin [Citation37] isotherm equations were tested to simulate the Cd(II) adsorption onto the biochar.
Figure 3. Adsorption isotherm for Cd(II) onto pristine biochar (a) and KB/LDH composite (b). IS = 0.01 M NaCl, pH = 6.5 ± 0.2 and T = 30 ± 2 °C.
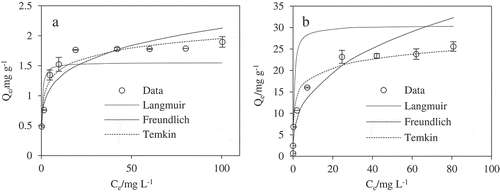
The amount of Cd(II) sorbed onto KB/LDH was calculated from the mass balance: Qe = (Co-Ce)V/m, where Qe is the amount adsorbed (mg g−1), m is the mass of the adsorbent and V is the volume of the solution. Co and Ce are the initial and equilibrium liquid-phase metal concentrations (mg L−1), respectively. To both materials, temkin model reproduced the isotherm data fairly well (.), with correlation coefficients (R2) exceeding 0.93 (). For the KB/LDH, the Temkin maximum capacity of KB/LDH composite was around 24.66 mg g−1. Fittings of the Langmuir matched the experimental data better than those of the Freundlich model. The hypothesis that there was an interaction in the adsorption layer to adsorb a single layer of inorganic molecules on a KB/LDH composite surface was verified. This result was consistent with the proposed predominant adsorption mechanism of Cd(II) removal by the biochar through mononuclear adsorption onto the Mg-Fe LDH particles on KB/LDH composite surface.
Table 2. Constants and correlation coefficient of isotherm models of Cd(II) adsorption on KB/LDH.
3.4 Effect of pH, IS and coexisting ions
The lowest adsorption appeared at pH 5.5 (). Cd(II) adsorption capacity decreased first from pH 7.5 to 5.5 and then increased from pH 5.5 to 3.5 at high concentration of Cd(II). The higher degree of oxidation formed more oxygen-containing functional groups on the biochar surface resulting in a lower pH. Therefore, the Cd(II) adsorption capacity at lower pH was higher than that at higher pH. Also, the hydroxy groups at low pH were deprotonated and the negatively charged organic functional groups became the main adsorption sites for Cd(II) [Citation38].
Figure 4. Effect of pH (a), IS (b) and coexisting ions (c) on Cd(II) adsorption onto KB/LDH composite.
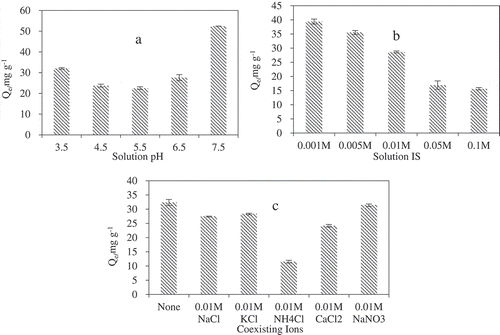
However, as the pH continued to increase to a certain degree, Cd(II) adsorption capacity increased. The reason for this was that it hydrolyzed or combined Cd(II) with hydroxide to form precipitation slightly at a higher pH [Citation39]. So we can know that the pH = 5.5 is unbeneficial for the adsorption of Cd(II).
. shows the effect of solution ionic strength on the sorption of Cd(II) on KB/LDH composite. Changing the ionic strength from 0.001 M to 0.1 M with NaCl had mixed effects on Cd(II) sorption on KB/LDH composite.
Increasing ionic strength can decrease Cd(II) sorption on KB/LDH composite (). With functional group, KB/LDH composite were carrying electronic charges. Because of the electrical double layer compression, increasing solution ionic strength promoted the aggregation of the KB/LDH composite in the solution. As a result, the sorption sites on KB/LDH composite were not completely available for Cd(II) sorption due to the molecular sieving effect. Therefore, the decrease of Cd(II) sorption was observed here.
In addition, ionic strength impeded Cd(II) sorption the most to KB/LDH composite-NH2, due to the fact that ionic strength can affect the strong electrostatic attractions between the KB/LDH composite and Cd(II). When counterions were introduced to the system, they could compete for the charged functional groups (i.e.-NH2) to block the sorption sites, reducing the electrostatic attraction between Cd(II) and KB/LDH composite-NH2.
In real systems, other ions can be present and compete with Cd(II) for adsorption sites. . shows the effects of the coexistence of NaCl, KCl, NH4Cl, CaCl2 and NaNO3 on Cd(II) adsorption in terms of adsorption capacity at equilibrium (Qe). Compared with CK(The solution of Cd(II) without other ions), all ions decrease Cd(II) sorption on KB/LDH composite (.). However, it can be seen that the influence of NaNO3 on Cd(II) removal efficiency is smaller than NaCl and it can be negligible. Mg-Fe LDH can incorporate Cl− ions, but not NO3− ions, into its interlayer space via ion exchange. This was due to the ion-sieve effect produced by the spatial limits between the brucite layers [Citation40]: The large diameter of macromolecular matter, it is not easy to enter into the micropore of brucite layers, but distributes between the brucite layers. In addition to diffusing in the gap of brucite layers, small molecules can also enter the micropores of brucite layers. The ion diameters of nitrate (0.29 nm) were larger than those of Cl (0.099 nm), so the ion of Cl can get into the micropore of brucite layers easier than nitrate [Citation17]. It also suggested that the KB/LDH composite hardly remove NO3− and Cd(II) simultaneously.
In general, ions of higher valence had a more significant interfering effect than monovalent ions in the oxyanion adsorption by LDH [Citation41]. While the interfering effects of competing cations on Cd(II) adsorption by KB/LDH composite followed the order of NH4+>Ca2+ > Na+ > K+, such an unusual ion-exchange behavior cannot be explained simply by the Coulomb interaction between the ion exchange site on Mg-Fe LDH nanosheets [Citation17]. KB/LDH can also effectively remove various positively charged compounds such as ammonia from aqueous solution and the sorption mechanisms including precipitation, surface complexation, isomorphic substitution and chelation with anions in the interlayers of LDHs [Citation42].
3.5 Evaluation of adsorption performance
By comparing with the previously reported biochar adsorbents, we can know that the modified kiwi branch biochar exhibited a higher adsorption capacity of Cd(II) than Pine shavings, Oakwood, and Rice husk and other biocarbon sources (), indicating that kiwi biochar-based Mg-Fe LDH composites have obvious advantages.
Table 3. Comparison of the adsorption capacity of Cd(II) on various low-cost adsorbents.
4. Conclusions
KB/LDH demonstrated a superior ability to remove Cd(II) compared with pristin biochar. The characters of KB/LDH including BET, SEM-EDS, FTIR and XRD analysis were researched to investigate the possible removal mechanism. It was suggested that the efficient removal of Cd(II) included the main ion exchange and the complexation of the abundant functional groups including amino, hydroxyl and carboxyl group with Cd(II). The surface precipitation of metal hydroxides was also a possible contribution to the removal of heavy metal ions. What is more, the efficency of adsorption was influenced by pH, IS and competitive ion conditions. The competitive ions of K+, Cl− and NO3- had an irrespective effect on the adsorption process. But the adsorption efficency was improved with the decreasing of IS and decreased first and then grew larger with the increase of pH. Batch sorption experiments and characterizations suggested that Cd(II) removal was mainly controlled by adsorption onto colloidal and Mg-Fe LDH particles on the KB/LDH surface. The use of KB/LDH has the benefit of additional energy generation and more efficient production (with less CO2 release during production). Thus, KB/LDH should be considered as a promising alternative water treatment or environmental remediation material for Cd(II) removal.
Disclosure statement
No potential conflict of interest was reported by the authors.
Additional information
Funding
References
- Wang T, Cao Y, Qu G, et al. Novel Cu(II)-EDTA decomplexation by discharge plasma oxidation and coupled Cu removal by alkaline precipitation: underneath mechanisms. Environ Sci Technol. 2018;52(14):7884–7891.
- Romero-Dondiz EM, Almazán JE, Rajal VB, et al. Comparison of the performance of ultrafiltration and nanofiltration membranes for recovery and recycle of tannins in the leather industry. J Clean Prod. 2016;135:71–79.
- Luo H, Liu G, Zhang R, et al. Heavy metal recovery combined with H2 production from artificial acid mine drainage using the microbial electrolysis cell. J Hazard Mater. 2014;270:153–159.
- Shaban M, Abukhadra MR. Geochemical evaluation and environmental application of Yemeni natural zeolite as sorbent for Cd2+ from solution: kinetic modeling, equilibrium studies, and statistical optimization. Environ Earth Sci. 2017;76(8):310.
- Gui CX, Wang QQ, Hao SM, et al. Sandwichlike magnesium silicate/reduced graphene oxide nanocomposite for enhanced Pb2⁺ and methylene blue adsorption. ACS Appl Mater Interfaces. 2014;6(16):14653–14659.
- Zhao F, Repo E, Yin D, et al. EDTA-cross-linked β-cyclodextrin: an environmentally friendly bifunctional adsorbent for simultaneous adsorption of metals and cationic dyes. Environ Sci Technol. 2015;49(17):10570–10580.
- Chen B, Chen Z, Lv S. A novel magnetic biochar efficiently sorbs organic pollutants and phosphate. Bioresour Technol. 2011;102(2):716–723.
- Novak JM, Lima I, Xing BS, et al. Characterization of designer biochar produced at different temperatures and their effects on a loamy sand. Ann Environ Sci. 2009;3:195–206.
- Chan KY, Van Zwieten L, Meszaros I, et al. Using poultry litter biochars as soil amendments. Soil Res. 2008;46(5):437–444.
- Cao XD, Ma L, Gao B, et al. Dairy-manure derived biochar effectively sorbs lead and atrazine. Environ Sci Technol. 2009;43(9):3285–3291.
- Mendez A, Gomez A, Paz-Ferreiro J, et al. Effects of sewage sludge biochar on plant metal availability after application to a Mediterranean soil. Chemosphere. 2012;89(11):1354–1359.
- Devi P, Saroha AK. Risk analysis of pyrolyzed biochar made from paper mill effluent treatment plant sludge for bioavailability and eco-toxicity of heavy metals. Bioresour Technol. 2014;162:308–315.
- Mohan D, Sarswat A, Ok YS, et al. Organic and inorganic contaminants removal from water with biochar, a renewable, low cost and sustainable adsorbent–a critical review. Bioresour Technol. 2014;160:191–202.
- Fan G, Li F, Evans DG, et al. Catalytic applications of layered double hydroxides: recent advances and perspectives. Chem Soc Rev. 2014;43(20):7040–7066.
- Zhao J, Chen J, Xu S, et al. CoMn-layered double hydroxide nanowalls supported on carbon fibers for high-performance flexible energy storage devices? J Mater Chem A. 2013;1(31):8836–8843.
- Tezuka S, Chitrakar R, Sonoda A, et al. Studies on selective adsorbents for oxo-anions. NO3− adsorptive properties of Ni-Fe layered double hydroxide in seawater. Adsorpt J Int Adsorpt Soc. 2005;11(1):751–755.
- Sasai R, Norimatsu W, Matsumoto Y. Nitrate-ion-selective exchange ability of layered double hydroxide consisting of MgII and FeIII. J Hazard Mater. 2012;215:311–314.
- Halajnia A, Oustan S, Najafi N, et al. Adsorption–desorption characteristics of nitrate, phosphate and sulfate on Mg–Al layered double hydroxide. Appl Clay Sci. 2013;80:305–312.
- Zhang M, Gao B, Yao Y, et al. Phosphate removal ability of biochar/MgAl-LDH ultra-fine composites prepared by liquid-phase deposition. Chemosphere. 2013;92(8):1042–1047.
- Wan S, Wang S, Li Y, et al. Functionalizing biochar with Mg–Al and Mg–Fe layered double hydroxides for removal of phosphate from aqueous solutions. J Ind Eng Chem. 2017;47:246–253.
- Yu J, Zhu Z, Zhang H, et al. Mg-Fe layered double hydroxide assembled on biochar derived from rice husk ash: facile synthesis and application in efficient removal of heavy metals. Environ Sci Pollut Res. 2018;25(24):24293–24304.
- Lee Y, Choi JH, Jeon HJ, et al. Titanium-embedded layered double hydroxides as highly efficient water oxidation photocatalysts under visible light. Energy Environ Sci. 2011;4(3):914–920.
- Ming Z, Gao B, Fang J, et al. Self-assembly of needle-like layered double hydroxide (LDH) nanocrystals on hydrochar: characterization and phosphate removal ability. RSC Adv. 2014;4(53):28171–28175.
- Wu XL, Wang L, Chen CL, et al. Water-dispersible magnetite-graphene-LDH composites for efficient arsenate removal. J Mater Chem. 2011;21(43):17353–17359.
- Bruna F, Celis R, Real M, et al. Organo/LDH nanocomposite as an adsorbent of polycyclic aromatic hydrocarbons in water and soil–water systems. J Hazard Mater. 2012;225:74–80.
- Yao Y, Gao B, Inyang M, et al. Biochar derived from anaerobically digested sugar beet tailings: characterization and phosphate removal potential. Bioresour Technol. 2011;102(10):6273–6278.
- Ho YS. Comments on using of “pseudo-first-order model” in adsorption. Int J Biol Macromol. 2016;88:505–506.
- Luo X, Jedlicka S, Jellison K. Pseudo-second-order calcium-mediated cryptosporidium parvum oocyst attachment to environmental biofilms. Appl Environ Microbiol. 2017;83(1):e02339–16.
- Peers AM. Elovich adsorption kinetics and the heterogeneous surface. J Catal. 1965;4(4):499–503.
- Luo X, Yu L, Wang C, et al. Sorption of vanadium (V) onto natural soil colloids under various solution pH and ionic strength conditions. Chemosphere. 2017;169:609–617.
- Komy ZR, Shaker AM, Heggy SE, et al. Kinetic study for copper adsorption onto soil minerals in the absence and presence of humic acid. Chemosphere. 2014;99:117–124.
- Zou W, Han R, Chen Z, et al. Kinetic study of adsorption of Cu(II) and Pb(II) from aqueous solutions using manganese oxide coated zeolite in batch mode. Colloids Surf A PhysicochemEng Aspects. 2006;279(1–3):238–246.
- Weerasooriya R, Tobschall HJ, Seneviratne W, et al. Transition state kinetics of Hg(II) adsorption at gibbsite-water interface. J Hazard Mater. 2007;147(3):971–978.
- Axe L, Trivedi P. Intraparticle surface diffusion of metal contaminants and their attenuation in microporous amorphous Al, Fe, and Mn Oxides. J Colloid Interface Sci. 2002;247(2):259–265.
- Rossi CG, Heil DM, Bonumà NB, et al. Evaluation of the Langmuir model in the soil and water assessment tool for a high soil phosphorus condition. Environ Modell Software. 2012;38:40–49.
- Mittal A, Kurup L, Freundlich MJ. Langmuir adsorption isotherms and kinetics for the removal of tartrazine from aqueous solutions using hen feathers. J Hazard Mater. 2007;146(1–2):243–248.
- Johnson RD, Arnold FH. The temkin isotherm describes heterogeneous protein adsorption. Biochim Biophys Acta. 1995;1247(2):293–297.
- Wang Q, Wang B, Lee X, et al. Sorption and desorption of Pb(II) to biochar as affected by oxidation and pH. SciTotal Environ. 2018;634:188–194.
- Tünay O, Kabdaşli NI. Hydroxide precipitation of complexed metals. Water Res. 1994;28(10):2117–2124.
- Tezuka S, Chitrakar R, Sonoda A, et al. Studies on selective adsorbents for oxo-anions. Nitrate ion-exchange properties of layered double hydroxides with different metal atoms. Green Chem. 2004;6(2):104–109.
- Goh K-H, T-T L, Dong Z. Application of layered double hydroxides for removal of oxyanions: A review. Water Res. 2008;42(6–7):1343–1368.
- Liang X, Zang Y, Xu Y, et al. Sorption of metal cations on layered double hydroxides. Colloids Surf A PhysicochemEng Aspects. 2013;433:122–131.
- Zhang Y, Lin J, Liu Y, et al. Adsorption of cadmium ions by chemically modified biochar. J Wuhan Univ Sci Tech. 2016;39(1):48–52.
- Mohan D, Pittman CU Jr., Bricka M, et al. Sorption of arsenic, cadmium, and lead by chars produced from fast pyrolysis of wood and bark during bio-oil production. J Colloid Interface Sci. 2007;310(1):57–73.
- Xiang J, Lin Q, Cheng S, et al. Enhanced adsorption of Cd(II) from aqueous solution by a magnesium oxide–rice husk biochar composite. Environ Sci Pollut Res. 2018;25(14):14032–14042.
- Ding Z, Hu X, Wan Y, et al. Removal of lead, copper, cadmium, zinc, and nickel from aqueous solutions by alkali-modified biochar: batch and column tests. J Ind Eng Chem. 2016;33:239–245.
- Cheng Q, Huang Q, Khan S, et al. Adsorption of Cd by peanut husks and peanut husk biochar from aqueous solutions. Ecol Eng. 2016;87:240–245.
- Li R, Wang Z, Guo J, et al. Enhanced adsorption of ciprofloxacin by KOH modified biochar derived from potato stems and leaves. Water Sci Technol. 2018;77(4):1127–1136.