ABSTRACT
A pot experiment was conducted to study chemical speciations and bioavailability of cadmium (Cd) and lead (Pb) in the gray calcium soil from northwestern China, and accumulation and translocation of Cd and Pb in wheat (Triticum aestivum L.) grown on the soil was also studied. The results showed that significant changes of Cd and Pb fractions in the soil and Cd and Pb concentrations in the wheat were observed with increasing soil Cd and Pb concentrations, and both Cd and Pb in exchangebale fraction in the soil made the greatest contribution to the accumulation of Cd and Pb in the wheat. Higher bio-concentration factors (BCFs) and translocation factors (TFs) for Cd and lower BCFs and TFs for Pb were observed in the wheat, respectively, and Cd had much higher accumulation than Pb in the edible parts of the wheat. Therefore, Cd has higher risk than Pb when people eat the wheat.
Introduction
Heavy metal contamination of soils coming from industry or agriculture, such as smelting industries, residues from nonferrous metal mining, pesticides, fertilizers, municipal composts, are among the major environmental challenges in the world [Citation1–Citation6]. Excessive metal concentrations in the contaminated soils can result in soil quality degradation, crop yield reduction, and poor quality of agriculture products [Citation7]. The subsequently transferred and accumulated heavy metals in human beings’ bodies through the food chain might be a threat to health [Citation8–Citation12].
Several studies have suggested that the toxicity and mobility of heavy metals depends not only on their total amounts but also on their chemical fraction in soil [Citation13–Citation22]. There are many classification methods to assess the chemical fractions of heavy metals in soil [Citation15,Citation23–Citation25]. Tessier’s five-step sequential extraction method has been widely used, in which heavy metals in soils are categorized in the five fractions: exchangeable fraction (F1), carbonate fraction (F2), Fe-Mn oxide fraction (F3), organic matter fraction (F4) and a residual fraction (F5) [Citation15,Citation20,Citation23,Citation26]. F1 is bioavailable fraction; F2, F3 and F4 are potential bioavailable fractions; F5 is non-bioavailable fraction [Citation20,Citation27].
In recent decades, some wheat fields have been irrigated with wastewater from the industrial and agricultural activities in the arid area and therefore have been heavily polluted by heavy metals, especially by Cd and Pb in northwestern China [Citation8,Citation28], which caused a threat to human health. According to reported literature, about 1000 ha of agricultural soils have been polluted with Cd and Pb in Baiyin city of northwestern China [Citation28,Citation29]. In general, many heavy metals including Cd and Pb are nonessential elements with biological toxicity. In order to avoid the pollution of the food chain by Cd and Pb, it is essential to assess their bioavailability in soil [Citation30].Many studies have indicated that the uptake of heavy metals by plants was related not only to the physical and chemical properties of soils and soil types but also plant species [Citation31–Citation33].
Some studies [Citation11,Citation34] have reported chemical fractions of Cd and Pb and their bioavailability to wheat grown in polluted brown forest soil and oasis soil of China and found that both Cd and Pb in F1 in the soils made the greatest contribution to the accumulation of Cd and Pb in wheat, and Cd had higher accumulation than Pb in the edible parts of the wheat.
As crop consumed for their edible parts, wheat (Triticum aestivum L.) has been widely cultivated in northwestern China where individual consumption figures for the wheat by the local population is approximately 0.50 kg average per person per day [Citation35]. People who consume wheat grown in polluted soil by Cd-Pb in the area are at risk of an elevated Cd and Pb exposure. However, the bioavailability of Cd and Pb to wheat grown in gray calcium soil was not reported previously. The proposed work was aimed to study the chemical fraction of Cd and Pb and their bioavailability in the Cd-Pb polluted gray calcium soil in northwestern China using pot experiments. Human health risk from Cd and Pb in wheat was also evaluated.
Materials and methods
Experimental design
Experimental reagents were selected with superior grade (Tianjin Kermel Chemical Reagents Corporation, China) and certified reference material samples, GSS-1 (GBW07401) and GSB-6 (GBW10015) were used. The two parameters bio-concentration factor (BCF) and the translocation factor (TF) were calculated. BCF is the ratio of the total metal concentration in plant tissues to that in their rooted soils [Citation19,Citation36,Citation37], which indicates migration capacity of heavy metals from soil to plant tissues, and TF is the ratio of total metal concentration in other plant parts to that in roots [Citation19,Citation37], which indicates migration capacity of heavy metals from plant roots to the aboveground parts.
Pot experiment was conducted under open-air conditions in April 2018 at Baiyin city in northwestern China. This area belongs to a semi-arid region with low precipitation (180–450 mm annually), high evapotranspiration (1500–1600 mm annually), and high temperatures in the summer (16–28ºC). The soil used is gray calcium soil, excavated from an unpolluted wheat field at 0–20 cm depth with a spade in the suburb of Baiyin city, and sub-samples collected were mixed to obtain a composite sample in accordance with the standard method [Citation38]. The average concentration levels of Cd and Pb in the soil samples are 0.13 and 18.22 mg kg−1 dry weight (DW), respectively. The soil textures are mainly clay loam, and other soil properties are as follows (): pH (in H2O) 8.00, organic matter (OM) content 13.45 g kg−1, CaCO3 content 9.32%, and cation exchange capacity (CEC) 8.78 cmol kg−1 [Citation38].
Table 1. Chemical and physical properties of the soil used in pot experiment.
The soil sample was air-dried and ground, then passed through a 2 mm sieve to remove large stones and grass debris. Nine treatments including one control (CK) and eight amendments (T1-T8) were replicated three times in a randomized block design, which represented soils ranging from unpolluted to heavily polluted by Cd and Pb. The control was not amended with inorganic Cd and Pb. The others, one by one, were spiked together with different solutions of compound cadmium nitrate and lead nitrate to elevate soil, respectively, and the same time, sheep mature was added in the soils as fertilizer. Treatment levels of Cd and Pb in tested soil were listed in , which was designed based on our field pollution investigation in the Cd-Pb polluted soil [Citation8]. The heavy metal was added by spraying a solution of metal salt over relatively dry soil spread out on a large tray. The soil was turned over and sprayed several times, and watered and left for enough equilibration outdoors for 4 weeks, then 1.5 kg soil was transferred to each plastic pot (20 cm in diameter and 15 cm in height) before planting crops. The wheat plants were seeded directly into the soil in the pots, and 2 weeks after sowing, the number of seedlings was thinned to four per pot for wheat. During the experimental period, tap water was added to compensate for evaporation and transpiration and soil moisture content was maintained at approximately 60% of water-holding capacity.
Table 2. Treatment levels of Cd and Pb in tested soil (mg kg−1 DW).
Whole plants were harvested after 120 days of growth and cut with scissors into separate parts grown under and above the soil. Shoots (leaves + stems) and grains were washed three times using de-ionized water. Roots were washed thoroughly with tap water to eliminate soil particles, and rinsed three times with de-ionized water. Roots and shoots were dried in an oven at 70℃ to constant weight, and the grains were air-dried to constant weight. The grain chaff was removed with the machine (OHYA-25, Japan), and the grain was oven-dried at 70℃ to constant weight. The oven-dried samples were ground with a stainless steel grinder (FW-100, China), passed through 2 mm nylon sieve for the further experiment, and the dry weight (DW) of roots, shoots and grains were recorded.
Composite soil samples were collected from the pots after harvesting the crops, air-dried at room temperature, ground with pestle and mortar, passed through 100 mesh (φ = 0.149 mm) nylon sieve.
Plant samples and soil samples were stored in plastic bottles, respectively, before analyses.
Determination of Cd and Pb in soils and wheats
Total Cd and Pb in soils were extracted using the acid digestion mixture (HNO3-HClO4-HF) according to the national standards of China [Citation39]. Total Cd and Pb in wheat were extracted using the acid digestion mixture (HNO3-HClO4) [Citation40,Citation41]. The clear solutions obtained from the digestion were filtered and reconstituted to the desired volume for analysis using an atomic absorption spectrometer (AAS, M6MK2, Thermo Electron Corporation, USA), respectively.
Determination of Cd and Pb fraction in soil using Tessier’s sequential extractions
Tessier’s sequential extraction procedure was used in this experiment ().
Table 3. The sequential extraction procedure of metal fractions in the tested soil (1.0 g soil DW).
Cd and Pb were extracted by MgCl2 solution in F1; by HAc-NaAc solution in F2; by NH2OH•HCl solution in F3; by HNO3-H2O2-NH4Ac solution in F4; and by HNO3-HClO4-HF solution in F5 [Citation23]. Cd and Pb in soil solutions obtained from Tessier’s sequential extraction procedure were also determined by an atomic absorption spectrometer (AAS, M6MK2, Thermo Electron Corporation, USA).
Quality assurance of data generation
Replicates, blanks and certified reference materials, GSS-1 (GBW07401) and GSB-6 (GBW10015), were included for quality assurance. The total and various speciation Cd and Pb concentrations in soils and wheats were determined in triplicate, and the coefficient of variation ranged between 5% and 10% in the triplicate analysis. The percentage recovery from certified reference materials were from 90% to 110% throughout the analysis procedures.
Statistical analysis
The means and standard deviations (SD) which showed deviations of three replicates were calculated by Excel2016 for windows. One-way analysis of variance was carried out with SPSS19.0. The significant (p < 0.05) differences of metals concentration in soils and plant tissues were observed between treatments and multiple comparisons were made by the LSD test.
Results and discussion
Chemical fractions of Cd and Pb in the soil
The experiment results in and showed that with increasing Cd and Pb concentrations in soils, the concentrations of both Cd and Pb in F1, F2 and F3 increased significantly (p < 0.05) probably because of the addition of Cd and Pb in the form of a solution, which was highly bioavailable. The parameter fraction distribution coefficient (FDC) usually is defined as the percentage of each heavy metal fraction, and the FDCs of Cd and Pb increased significantly in F1 and F2 (p < 0.05) with increasing soil Cd and Pb concentration, however, those of Cd in F3 and Pb in F5 (p < 0.05) reduced significantly, respectively (). And the FDCs of Cd in F1 were much higher (or even more than 40%) than Pb, probably because Cd is more easily adsorbed by the gray calcium soil than Pb, and indicating greater mobility of Cd in the soil than Pb [Citation8].
Table 4. The concentration of Cd fractions in the soil with different Cd-Pb compound treatments (mg kg−1).
Table 5. Concentration of Pb fractions in the soil with different Cd-Pb compound treatments (mg kg−1).
Note: Values of Cd fractions concentration in the soil are presented as mean±SD of three replicates. One-way ANOVA (1 factor: different treatments) was performed for each parameter on Cd fractions concentration. Data in the same column followed by different letters are significantly different from each other (p < 0.05) according to the LSD test.
Note: Values of Pb fractions concentration in the soil are presented as mean±SD of three replicates. One-way ANOVA (1 factor: different treatments) was performed for each parameter on Pb fractions concentration. Data in the same column followed by different letters are significantly different from each other (p < 0.05) according to the LSD test.
Figure 1. Distribution of the FDCs of Cd and Pb in the soil, respectively.
Note: Values are means of three replicates.
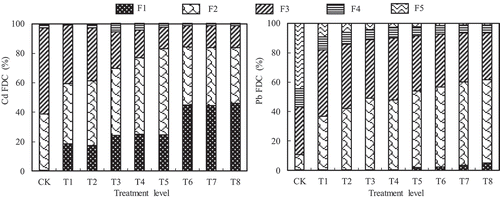
F5 comprises primary and secondary minerals within the crystal structure that is unlikely to be released in the mid-and long-term under the conditions normally found in nature. Previous studies have shown that in unpolluted soils, F5 was the main heavy metals fraction which was not bio-available for the plants [Citation42,Citation43], but the percentage of heavy metals associated with available fraction increased with the increase of total heavy metals in the growth medium [Citation26,Citation42–Citation44]. Bose et al [Citation45]. reported that Cd was bound with F3 in unpolluted garden soil in India. Similar results are observed in the present study. The results suggest that the fraction of both Cd and Pb in unpolluted soil is mainly in F3, however, the bioavailable fraction concentration increase significantly (p < 0.05), and the percentage of Cd and Pb associated with F5 decrease significantly (p < 0.05) with increased soil Cd and Pb concentrations.
Effects of Cd-Pb stress on wheat growth
The dry weight (DW) of roots, shoots and grains of wheat grown with different Cd-Pb treatments are shown in .
Table 6. Effects of Cd-Pb treatments on the biomass of wheat.
Note: Values of the wheat biomasses are presented as mean±SD of three replicates. One-way ANOVA (1 factor: different treatments) was performed for each parameter on wheat biomasses. Data in the same column followed by different letters are significantly different from each other (p < 0.05) according to the LSD test.
The dry biomass of wheat roots and shoots generally increased at first, and reached peak values, then decreased with increased soil Cd-Pb concentrations (). When the treatment level was T2 (0.75 mg kg−1 of Cd and 150 mg kg−1 of Pb), the dry biomass of wheat reached the maximum value,(1.96 ± 0.11 g pot−1 for root, 9.95 ± 1.22 for shoot, and 12.48 ± 2.60 for grain, respectively), increased by 63.33%, 19.02%, and 41.33%, respectively, compared with the control, indicating that this level of Cd-Pb was hazardous threshold of wheat, and beyond it, the growth of wheat would be inhibited.
Some studies [Citation11,Citation34] have reported that the dried weight of wheat increased at first, then decreased under soil Cd-Pb stress. This conclusion was in agreement with the present studies. These results indicated that the toxic effect of Cd and Pb on wheat had threshold values. Wheat growth could be enhanced by lower concentrations of Cd-Pb (≤0.75 mg kg−1 of Cd and 150 mg kg−1 of Pb), but inhibited by higher concentrations. This may be attributed to high concentrations of Cd-Pb that damaged wheat roots [Citation34], and inhibited the uptake of nutrient elements in roots, then inhibited the growth of wheat. Song et al. [Citation46] found lower concentrations of Cd in the soil increased uptake of potassium (K) and zinc (Zn), which usually are important nutrient elements to plants, in the rape root, but higher concentrations of Cd decreased uptake of K and Zn in the rape root. Fan et al. [Citation47] observed low concentrations of Cd in the soil could improve seed germination of wheat, while Cd with high concentration had inhibition on seed germination of wheat, and the content of chlorophyll in leaves of wheat seedlings was reduced, the activities of Superoxide dismutase (SOD) and Peroxidase (POD) in wheat seedling were decreased, and the contents of Malondialdehyde (MDA) and Proline (Pro) were increased, which led to normal metabolism of wheat root cells was destroyed and plant growth was inhibited.
Accumulation of Cd and Pb in wheat
The amounts and distribution of Cd and Pb accumulated in wheat under various treatments are shown in . Both Cd and Pb concentrations in wheat increased significantly (p < 0.05) with increased soil Cd-Pb concentrations. Compared with the control, Cd concentrations of wheat root, shoot and grain increased by 37.74 fold, 54.00 fold, and 32.88 fold, respectively; and Pb concentrations increased by 97.25 fold, 156.40 fold, 9.29 fold, respectively at the treatment level was T8 (7.50 mg kg−1 of Cd and 1350 mg kg−1 of Pb) on the soil, which showed uptake rates of both Cd and Pb in the shoot was more than the root and grain.
Table 7. Concentration (mg kg−1 DW) of Cd and Pb in wheat with different Cd-Pb compound treatments.
Note: Values of Cd and Pb concentration in wheat are presented as mean±SD of three replicates. One-way ANOVA (one factor: different treatments) was performed for each parameter on Cd and Pb concentration. Data in the same column followed by different letters are significantly different from each other (p < 0.05) according to the LSD test.
According to the China Environmental Quality Standard for Soils [Citation48], Cd and Pb limits for the soils used for wheat production are 0.6 mg kg−1 and 350 mg kg−1, respectively where soil pH >7.5. At Cd and Pb level of 0.6 mg kg−1 and 350 mg kg−1, respectively in soil, Cd and Pb concentrations in wheat grain were 0.35 mg kg−1 (calculated by statistical regression equation y= 0.3901x+0.1115, R2 = 0.932, p < 0.01, where y is Cd concentration in wheat, x is the concentration of Cd in soil) and 0.20 mg kg−1 (calculated by y= 0.0005x+0.0272, R2 = 0.963, p < 0.01), respectively. At this level of Cd and Pb addition, the national allowable limit of Cd in wheat was exceeded, but Pb in wheat was not exceeded, compared with maximum levels of contaminants in wheat foods, 0.1 mg kg−1 of Cd and 0.2 mg kg−1 of Pb, respectively [Citation49].
The present study found the order of both Cd and Pb accumulation in wheat was root > shoot > grain, and the concentrations of both Cd and Pb in the wheat grains were significantly lower than those in any other tissues (p < 0.05) (). Similar results were observed in the previous studies [Citation50–Citation52]. Guo et al. [Citation52] observed that Cd and Pb concentrations for different parts of wheat decreased markedly in the order of roots > leaves > stems > grains indicating that the roots act as a barrier for metal accumulation or translocation, and then protect the edible parts from Cd and Pb contamination.
The BCFs of Cd and Pb in wheat roots, shoots and grain under various treatments were shown in . The BCFs of both Cd and Pb for wheat decreased in root > shoot > grain. The BCF values of Cd were ranged from 4.00 to 8.46 for roots, 0.78 to 2.59 for shoots, 0.42 to 0.97 for grains, respectively; those of Pb 0.248 to 0.412 for roots, 0.004 to 0.045 for shoots, 0.000 to 0.000 for grains, respectively. Compared with the oasis soil (pH = 8.47) [Citation10,Citation11], the BCF values of Cd in wheat grown on gray calcium soil (pH = 8.00) were bigger probably due to the lower pH value of the soil, which showed Cd was easier to migrate from soil to wheat grown on gray calcium soil than oasis soil. In general, the BCFs for Cd in wheat were much higher than Pb (p < 0.05). Similar results were observed in the previous studies [Citation50–Citation52].
Table 8. BCFs and TFs of Cd and Pb in wheat with different Cd-Pb compound treatments.
The present study found the BCFs of both Cd and Pb in wheat increased at first, reached a maximum value, then decreased with increasing soil Cd-Pb concentrations (). Similar results were observed in the previous studies [Citation50–Citation52].
The present study found the TFs of both Cd and Pb for wheat decreased in shoot > grain which was probably because of Cd and Pb were mainly trapped in the roots and transportation from root to shoot was limited [Citation52]. The TFs of Cd in wheat under various treatments were ranged from 0.12 to 0.35 for shoots and 0.09 to 0.15 for grains, respectively (); those of Pb 0.013 to 0.109 for shoots and 0.001 to 0.012 for grains (). This is because Pb entering the root hair is easily adsorbed in the form of pyrophosphate, orthophosphate or pectic acid, and Pb of these forms is less active, tends to accumulate in the roots, and less migrates to the shoots. Compared with the oasis soil (pH = 8.47) [Citation10,Citation11], the TF values of Cd in wheat grown on gray calcium soil (pH = 8.00) were bigger probably due to the lower pH value of the soil, which showed Cd is easier to migrate from wheat roots to shoots grown on gray calcium soil than oasis soil. In general, the TFs of Cd in wheat were much higher than Pb, suggesting that Cd had greater mobility than Pb towards uptake by wheat. This conclusion was in agreement with the previous studies [Citation50–Citation52].
However, compared with artificially contaminated soil used in the pot experiment, Pb accumulation in wheat grain was much higher on the field-contaminated soil [Citation50–Citation52] probably due to wheat grains took up Pb coming from both polluted field soil and the atmosphere dusts which were emitted by lead-zinc smelters.
The above-mentioned results showed that the BCFs and TFs of both Cd and Pb in wheat had threshold values, beyond which, the roots of wheat may be damaged by Cd-Pb, although the uptake and accumulation of Cd and Pb in wheat was continuing, but their uptake rates gradually reduced, hence the BCFs of Cd and Pb in wheat increased at first, then decreased. The above results also suggested that wheat accumulated higher Cd concentrations than Pb in its edible parts grown on the polluted soil. Therefore, Cd in wheat grown on the Cd-Pb polluted soil and consumed by humans has a higher risk to human health than Pb.
Interaction between Cd and Pb in soil and cole
Concentrations of both Cd and Pb in wheat under Cd-Pb compound contamination () were lower than those under single Cd or Pb contamination (), which indicated that there was antagonistic effect between Cd and Pb in soil under Cd-Pb compound pollution.
Table 9. Concentrations (mg kg−1 DW) of Cd and Pb in wheat with single Cd or Pb treatment.
Note: Values of Cd and Pb concentrations in wheat are presented as mean±SD of three replicates. One-way ANOVA (1 factor: different Cd or Pb treatment) was performed for each parameter on Cd or Pb concentration. Data in the same column followed by different letters are significantly different from each other (p < 0.05) according to the LSD test.
Yang et al. [Citation53] found that Cd-Pb compound pollution had a synergistic effect on both Cd and Pb, each metal could promote another metal uptake in wheat on loess cinnamon soil. Wu et al. [Citation54] observed that Cd–Pb had a synergistic effect on the Cd and Pb uptake of wheat in brown soil (pH 6.60) and cinnamon soil (pH 8.36) under the lower ratio of Cd and Pb content (Cd: Pb <0.005) condition. The present study (pH 8.00) is inconsistent with previous reports probably due to the different soil types and higher the ratio of Cd and Pb content (Cd: Pb≥0.005) in the soil.
Relationship between the accumulation in wheat and chemical fractions of Cd and Pb in the soil
Significantly positive correlation (p < 0.01) was found between the FDCs of both Cd and Pb in F1 in the soil and Cd and Pb concentrations in wheat roots, shoots and grains, respectively (), the corresponding correlation coefficients (R) of Cd were 0.902 for roots, 0.907 for shoots and 0.933 for grains, respectively; and those of Pb were 0.972 for roots, 0.944 for shoots and 0.970 for grains, respectively. This is because Cd and Pb in F1 adsorbed on the soil are more easily desorbed and enters the soil solution and are absorbed by the wheat.
Table 10. Correlation coefficients (R) between the FDCs of Cd and Pb in the soil and concentrations of Cd and Pb in wheat roots, shoots and grains (n = 9).
Fu et al. [Citation55] reported that there was a significant positive correlation between Cd content in wheat grain and soil exchangeable and carbonate-bound Cd, while Pb content in grain was significantly positively correlated with soil carbonate-bound Pb, and the correlation was not significant between grain and exchangeable Cd from calcareous soil (pH = 7.60, stepwise regression analysis between the concentrations of Cd and Pb fraction in soil and those in wheat grain). This conclusion is not in agreement with the present study (pH = 8.00, stepwise regression analysis between the fraction distribution coefficient of Cd and Pb in soil and the concentrations of Cd and Pb in wheat grain) probably because of different soil properties and regression analysis method. The present study showed that both exchangeable Cd and Pb (F1) were the most available fraction for wheat, which indicated that both Cd and Pb in F1 made the greatest contributions to the accumulation of Cd and Pb in the roots, shoots and grains of wheat ( and ).
Figure 2. Stepwise regression analysis between the concentrations of Cd and Pb in F1 in soil and those in wheat, respectively.
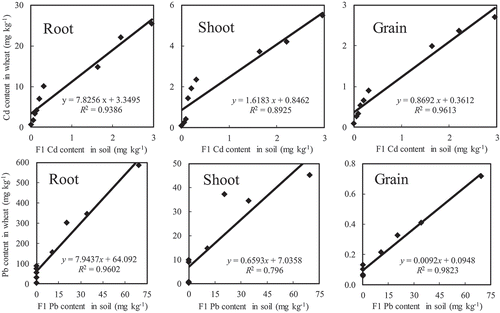
Yang et al. [Citation11] reported that there was a significantly negative correlation between the percentage of Cd in F3 and F5 in the oasis soil and the accumulation of Cd in the roots, shoots and grains of wheat. Fu et al. [Citation55] found that there was a significant negative correlation between Cd and Pb content in wheat grain and soil residual Cd and Pb. In the present study, a significantly negative correlation was also observed between the FDCs of Cd in both F3 and F5 and Pb in both F3 and F4 in the soil and Cd and Pb concentrations in wheat roots, shoots and grains, respectively (). This was because Cd and Pb in F3, F4 and F5 in the soil were not almost absorbed by the wheat, which indicated that Cd in F3 and F5 and Pb in both F3 and F4 were unavailable fraction for wheat.
Conclusions
Cadmium (Cd) in the unpolluted soil was mainly bound to carbonate fraction (F2) and Fe-Mn oxide fraction (F3), and lead (Pb) was mainly bound to carbonate fraction (F2) and a residual fraction (F5). However, the fractional distribution of Cd and Pb changed significantly with increasing both Cd and Pb concentrations in soil, where the concentrations of Cd in F1 (exchangeable fraction), F2 and F3, and Pb in F1, F2, F3 and F4 increased significantly.
Relatively lower concentrations of both Cd and Pb could facilitate the growth of wheat, but their growth was inhibited at higher concentrations. Both Cd and Pb in F1 made the greatest contribution to the accumulation of Cd and Pb in wheat. Both bioconcentration factor and translocation factor of Cd in wheat were higher than Pb, and Cd had higher accumulation in the edible part of wheat.
Therefore, Cd in wheat grown on the Cd-Pb compound polluted soil has a much higher potential of risk to human health than Pb according to individual consumption figures for wheat which is approximately 0.50 kg average per person per day.
Disclosure statement
No potential conflict of interest was reported by the authors.
Additional information
Funding
References
- Li LM, Wu J, Lu J, et al. Distribution, pollution, bioaccumulation, and ecological risks of trace elements in soils of the northeastern Qinghai-Tibet Plateau. Ecotox Environ Safe. 2018;166:345–353.
- Ratnakar A. Shikha.Assessment of co-contamination in soil samples from agricultural areas in and around Lucknow city, Uttar Pradesh, India. Curr Sci India. 2018;115::2267–2274.
- Doyi I, Essumang D, Gbeddy G, et al. Spatial distribution, accumulation and human health risk assessment of heavy metals in soil and groundwater of the Tano Basin, Ghana. Ecotox Environ Safe. 2018;165:540–546.
- Noudeng V, Hoang DT, Tran DX, et al. Heavy metal accumulation in water, soil, and plants of municipal solid waste landfill in vientiane, Laos. Int J Environ Res Public Health. 2019;16:1–13.
- Guo YH, Sun XC, Zhang SB, et al. Pollution characteristics, source analysis and potential ecological risk assessment of heavy metals in soils surrounding a municipal solid waste incineration plant in Shanghai. Huanjing Kexue. 2017;38:5262–5271.
- Liu BL, Ai SW, Zhang WY, et al. Assessment of the bioavailability, bioaccessibility and transfer of heavy metals in the soil-grain-human systems near a mining and smelting area in NW China. Sci Total Environ. 2017;609:822–829.
- Chen HM. Behavior of chemicals in soils and its relation to environmental quality. Beijing: Science Press; 2002.
- Nan ZR, Liu XW, Zhao ZJ, et al. Chemical behavior and ecological risk assessment in soil-crop system in arid oasis region. Beijing: Chinese Environmental Science Press; 2011.
- Yang YM, Nan ZR, Zhao ZJ, et al. Bioaccumulation and translocation of cadmium in cole (Brassica campestris L.) and celery (Apium graveolens) grown in the polluted oasis soil, northwest of China. J Environ Sci. 2011;23::1368–1374.
- Wang ZW, Nan ZR, Wang SL, et al. Accumulation and distribution of cadmium and lead in wheat (Triticum aestivum L.) grown in contaminated soils from the oasis, north-west China. J Sci Food Agric. 2011;91:377–384.
- Yang YM, Nan ZR, Zhao ZJ. Bioaccumulation and translocation of cadmium in wheat (Triticum aestivum L.) and maize (Zea mays L.) from the polluted oasis soil of Northwestern China. Chem Speciation Bioavailability. 2014;26:43–51.
- Yang YM, Li Y, Zhang JH. Chemical speciation of cadmium and lead and their bioavailability to cole (Brassica campestris L.) from multi-metals contaminated soil in northwestern China. Chem Speciation Bioavailability. 2016;28:33–41.
- Chojnacka K, Chojnacki A, GÙrecka H, et al. Bioavailability of heavy metals from polluted soils to plants. Sci Total Environ. 2005;33:175–182.
- Gupta AK, Sinha S. Chemical fractionation and heavy metal accumulation in the plant of Sesamum indicum (L.) var. T55 grown on soil amended with tannery sludge, selection of single extractants. Chemosphere. 2006;64::161–173.
- Silveira ML, Alleoni LRF, O’Connor GA, et al. Heavy metal sequential extraction methods – A modification for tropical soils. Chemosphere. 2006;64:1929–1938.
- Kartal S, Aydın Z, Tokaliog LS. Fractionation of metals in street sediment samples by using the BCR sequential extraction procedure and multivariate statistical elucidation of the data. J Hazard Mater. 2006;132:80–89.
- Li MS, Luo YP, Su ZY. Heavy metal concentrations in soils and plant accumulation in a restored manganese mineland in Guangxi, South China. Environ Pollut. 2007;147:168–175.
- Tandy S, Healey JR, Nason MA, et al. Heavy metal fractionation during the co-composting of biosolids, deinking paper fibre and green waste. Bioresource Technol. 2009;100:4220–4226.
- Wang QY, Zhou DM, Cang L, et al. Solid/solution Cu fractionation of a Cu contaminated soil after pilot-scale electrokinetic remediation and their relationships with soil microbial and enzyme activities. Environ Pollut. 2009;157:2203–2208.
- Rodríguez L, Ruiz E, Alonso-Azcárate J, et al. Heavy metal distribution and chemical speciation in tailings and soils around a Pb-Zn mine in Spain. J Environ Manage. 2009;213:533–540.
- Tan D, Long JM, Li BY, et al. Fraction and mobility of antimony and arsenic in three polluted soils: A comparison of single extraction and sequential extraction. Chemosphere. 2018;90:1106–1116.
- Pavlovic D, Pavlovic M, Cakmak D, et al. Fractionation, mobility, and contamination assessment of potentially toxic metals in urban soils in four industrial Serbian cities. Arch Environ Con Toxi. 2018;75:335–350.
- Tessier A, Campbell PGC, Bisson M. Sequential extraction procedure for the speciation of particulate tracemetals. Anal Chem. 1979;51:844–851.
- Ahnstrom ZS, Parker DR. Development and assessment of a sequential extraction procedure for the fractionation of soil cadmium. Soil Sci Soc Am J. 1999;63:1650–1658.
- Qiao XL, Luo YM, Christie P, et al. Chemical speciation and extractability of Zn, Cu and Cd in two contrasting biosolids-amended clay soils. Chemosphere. 2003;50:823–929.
- Lucho-Constantino CA, Prieto-García F, Del Razo LM, et al. Chemical fractionation of boron and heavy metals in soils irrigated with wastewater in central Mexico. Agric Ecosys Environ. 2005;108:57–71.
- He ZL, Yanga XE, Stoffellab PJ. Trace elements in agroecosystems and impacts on the environment. J Trace Elements Med Biol. 2005;19:125–140.
- Chen HM. Environmental soil. Beijing: Science Press; 2005.
- Lei SW, Wu GZ, Wang XF. Investigation and analysis on the distribution of heavy metals in soil and spring wheat in Baiyin city of China. Gansu Metallurgy. 2007;4:87–88.
- Cornu JY, Parat C, Schneider A, et al. Cadmium speciation assessed by voltammetry, ion exchange and geochemical calculation in soil solutions collected after soil rewetting. Chemosphere. 2009;76:502–508.
- McBride MB. Toxic metals in sewage sludge-amended soils, has promotion of beneficial use discounted the risks? Adv Environ Res. 2003;8:5–19.
- Sun JL, Wu WJ, Zhao RX, et al. Studies on pollution of heavy metals in soils and technology of plant remediation. J Changchun Univ Sci Technol. 2003;26:46–48.
- Kidd PS, Domínguez-Rodríguez MJ, Díez J, et al. Bioavailability and plant accumulation of heavy metals and phosphorus in agricultural soils amended by long-term application of sewage sludge. Chemosphere. 2007;66:1458–1467.
- Chen S, Sun L, Sun TH, et al. Influence of urea fertilization on the speciation distribution and phytoavailability of cadmium and lead in soil. Chinese J Ecolog. 2010;29:2003–2009.
- Chinese Nutrition Society. Chinese dietary guidelines. Lasa: Tibet People Press; 2007.
- Yang Y, Zhang FS, Li HF, et al. Accumulation of cadmium in the edible parts of six vegetable species grown in Cd-contaminated soils. J Environ Manage. 2009;90:1117–1122.
- Sun YB, Zhou QX, Wang L, et al. Cadmium tolerance and accumulation characteristics of Bidens Pilosa L. as a potential Cd-hyperaccumulator. J Hazard Mater. 2009;161:808–814.
- MEPPRC (Ministry of Environmental Protection of the People’s Republic of China). The technical specification for soil environmental monitoring. 1st ed. Beijing: China Standard Press; 2004. p. p. 1–44.
- MEPPRC-GAQSIQPRC (Ministry of Environmental Protection of the People’s Republic of China and General Administration of Quality Supervision, Inspection and Quarantine of the People’s Republic of Chin). Soil quality determination of lead, cadmium-graphite furnace atomic absorption spectrophotometry. 1st ed. Beijing: China Standard Press; 1997. p. p. 1–5.
- MEPPRC-SAC (Ministry of Health of the People’s Republic of China and Standardization Administration of China). Determination of cadmium in foods. 1st ed. Beijing: China Standard Press; 2003. p. 1–10.
- MEPPRC-SAC (Ministry of Health of the People’s Republic of China and Standardization Administration of China). Determination of lead in foods. 1st ed. Beijing: China Standard Press; 2003. p. 85–98.
- Gao YZ, He JZ, Ling WT. Fractionation of heavy metal cadmium and copper in some soils in Hubei province. J Huazhong Agric Univ. 2001;20:143–147.
- Wang X, Zhou QX. Distribution of forms for cadmium, lead, copper and zinc in soil and its influences by modifier. J Agro Environ, Sci. 2003;22:541–545.
- Zhou GH, Huang HC, He HL. Behavior and fate of cadmium in natural and CdCl2-spiked soils in southeastern suburb of Beijing. J Agro Environ, Sci. 2003;22:25–27.
- Bose S, Jain A, Rai V, et al. Chemical fractionation and translocation of heavy metals in Canna indica L. grown on industrial waste amended soil. J Hazard Mater. 2008;160:187–193.
- Song ZG, Xu MG, Liu P, et al. Effect of coexistence of potassium and zinc at different ratios on the cadmium bioavailability in lateritic red soil. Eco Environ Sci. 2009;18:904–908.
- Fan MQ, Li YY, Miao J, et al. Influence of Cd2+ stress on germination and seeding growth of wheat. J Anhui Agri Sci. 2010;38:14239–14241.
- SEPAC (State Environmental Protection Administration of China). Environmental quality standard for soils. 1st ed. Beijing: China Standard Press; 1995. p. 1–11.
- MEPPRC-SAC (Ministry of Health of the People’s Republic of China and Standardization Administration of China). Maximum levels of contaminants in foods. 1st ed. Beijing: China Standard Press; 2005. p. 1–11.
- Nan ZR, Cheng GD. Accumulation of Cd and Pb in spring wheat (Triticumaestivum L.) grown in calcareous soil irrigated with wastewater. Environ Contam Toxicol. 2001;66:748–754.
- Wang XR, Zhou SL, Wu SH. Accumulation of heavy metals in different parts of wheat plant from the Yangtze River Delta, China. Int J Agric Biol. 2016;18:1242–1248.
- Guo GH, Lei M, Wang YW, et al. Accumulation of As, Cd, and Pb in sixteen wheat cultivars grown in contaminated soils and associated health risk assessment. Int J Environ Res Public Health. 2018;15:2601–2617.
- Yang JZ, Feng LR. Effects of combined pollution stress of cadmium, chromium and lead in soil on heavy metal content in different organs of wheat and corn. Proc 21st Century Crop Sci Technol Develop Symp. 2002;4:152–158.
- Wu YY, Wang X, Liang RL, et al. Ecological effect of compound pollution of heavy metals in soil-plant system Ⅱ. Effect on element uptake by crops, alfalfa and tree. Chinese J Applied Eco. 1997;8:545–552.
- Fu HC, Zhang B, Yang J, et al. Cadmium and lead speciation as affected by soil amendments in calcareous soil. Environ Eng Sci. 2018;35:937–942.