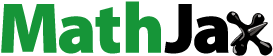
ABSTRACT
This study investigates the pollution status, spatial distribution of heavy metals including Cr, Mn, Ni, Cu, Zn, As, Cd and Pb, in sediments from the Longhu Lake, and evaluates possible ecological risk of these metals. Average concentrations of Cu, Zn, Cd and Pb were 2.1, 3.5, 8.0 and 2.5 times higher than Grade I Chinese soil quality standard respectively. The geo-accumulation index indicates that the lake sediments are moderate to heavily contaminated by Zn, Cd and Pb, which may have higher toxic effects than other metals. The potential ecological risk factor (Eir) suggests low risk to aquatic organisms except for Cd which has a considerable risk to aquatic biota. Spearman correlation and principal component analysis suggest that Mn, Zn, Cd and Pb possibly having similar anthropogenic sources and behaviors in the lake. Overall, this study suggests that anthropogenic inputs are from local point and non-point sources, runoff and atmospheric deposition.
1. Introduction
In the aquatic ecosystems especially in the freshwater systems (e.g. lakes and rivers), heavy metals are considered as predominant pollutants due to their persistence, toxicity, accumulation and bio-magnification ability in the food webs [Citation1,Citation2]. Over the last decades due to unprecedented economic development, heavy metals pollution has increased in the aquatic systems because of industrial activities, urbanization, agricultural activities, mining and burning of fossil fuels [Citation3–5]. In the aquatic ecosystems, heavy metals entered through surface runoff, fluvial transport, atmospheric deposition; after that accumulate and sink in sediment through the process of adsorption, co-precipitation and hydrolysis [Citation6,Citation7].
Sediments act as a salient sink of heavy metals in the aquatic environment and higher accumulation and concentration than overlying water [Citation8]. Besides, sediment is the carrier of heavy metals and these metals can be released to the overlying water due to changes of surrounding physical (e.g. disturbance, floods, temperature, salinity) and chemical factors (e.g. pH, redox potential and dissolved oxygen) of sediments [Citation8]. As a result, sediments are considered as sources of secondary pollution of heavy metals in the aquatic ecosystems [Citation3]. Sediment is the primary habitat for benthic/filter-feeding organisms, and metal pollution has a detrimental effect on them. Due to the bioaccumulation capacity of heavy metals in tissues, these pollutants have a further effect on other aquatic organisms as well as terrestrial organisms and ultimately on humans [Citation3]. So, monitoring of sediment pollution is an important tool to evaluate the pollution status and potential toxicity of heavy metals in the aquatic ecosystems which are essential for the protection of aquatic ecosystems and human health [Citation3,Citation9]. Accessible freshwater is only 0.3% of global water and according to the United Nations, about 2.8 billion people will be facing scarcity of freshwater access during 2025 (https://www.unwater.org/water-facts/scarcity/). So, freshwater lakes are precious resources which should be monitored to keep them free from pollution for current as well as future generations.
Heavy metals are a serious threat to Chinese lake sediments due to rapid industrialization and urbanization [Citation5]. In recent times, lake sediment pollution has attained much attention from the scientific community due to the lack of effective management and supervision policy [Citation10–13]. In 2019, China legislated the land-based soil management policy to reduce metals pollution [Citation14,Citation15]. A recent review suggests that 20.6% of Chinese lakes sediment metals concentrations are greater than the Chinese grade II soil quality standard [Citation5]. In terms of potential ecological risk 21.8%, 10.9%, and 12.7% of lakes are in the category of moderate risk, high risk and very high risk of heavy metals, respectively, [Citation5].
Lake Longhu is the second largest natural lake in Fujian province. This lake is an important freshwater source for the Jinjiang city and Jinmen island people. This lake is surrounded by industrial and agricultural activities and several cyanobacterial events have been recorded due to eutrophication [Citation16]. To our knowledge, there are no recent data available of heavy metals in sediments of this lake except one Chinese article [Citation17] published more than 15 years ago. This lake is very important for drinking water and natural fisheries for about 300 thousand local people. So, monitoring and assessment of heavy metals pollution are very crucial for the health of residents. In addition, this lake is now used for natural fisheries activities (without adding any artificial food supply) and these fish are very popular in local areas. As a result, these fish may be another health risk for local people. Heavy metal pollution levels in sediment can predict the ecological risk of heavy metals to biota. These results could be useful for management authority and policymakers in deciding to protect this lake from pollution.
The objectives of the present study are to (1) investigate the spatial distribution of heavy metals in the surface sediment of Longhu Lake, (2) investigate the pollution status, sources and potential effect on biota through different geochemical and sediment quality indices. This study would also provide the baseline information about the heavy metals pollution and potential risk of Longhu Lake sediments which could be beneficial for the policymakers for future management strategy.
2. Materials and methods
2.1. Study area and sediment sampling
Longhu Lake is situated in Jinjiang City (24°38´N, 118°36´E) of Fujian Province in the southeast of China (). This Lake is one of the largest natural freshwater lake and fish farm in Fujian Province. The total surface area of this Lake is 1.62 km2 with watershed areas of 9.6 km2. This is a shallow lake with an average depth of 2.5 m having a water storage capacity of 4 million m3. The Longhu Lake is the primary drinking water source for local people as well as Jinmen island people that belongs to Taiwan, China. Besides, this lake contributes fisheries for local people with an annual production of about 146tons. Eutrophication events have been occurred due to an increase in nutrients load from agricultural and industrial activities from the surrounding areas [Citation16].
Figure 1. Sediment sampling areas of Longhu Lake at Quanzhou in China (S1: inlet; S2: upstream; S3: upstream; S4: mid – stream; S5: mid – stream; S6: downstream; S7: downstream)
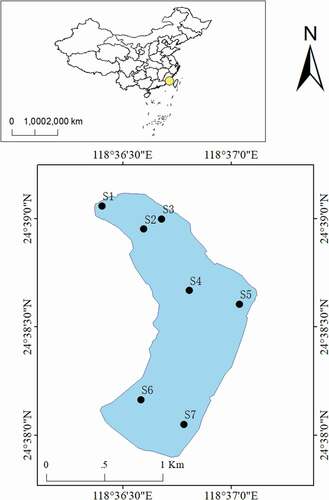
Sediment samples were collected from seven sampling sites () of Longhu Lake including inlet mouth (S1), upstream (S2, S3), mid-stream (S4, S5) and downstream (S6, S7) area. Bottom surface sediment (0–10 cm) was accumulated by the Peterson grabber and 100 gm of sediment was collected in an acid-washed Ziplock plastic bag by plastic spoon and stored at 4°C until they arrived at the laboratory. From each location, triplicate samples were collected and stored at −18°C until further analysis.
2.2. Samples preparation, digestion and quantification
Before starting the sample preparation, all sampling equipments were soaked with 10% Nitric acid for more than 24 h and washed with Milli-Q (18.2 MΩ, Millipore, USA) water, and oven-dried to remove all contaminates. All chemicals used for sample preparations were analytical grade. Sediment samples were oven-dried at 80°C for more than 72 h. After getting the constant mass, samples were homogenized, grounded with agar mortar and pestle, and sieved by 160 mesh nylon fiber [Citation18,Citation19]. Sediment samples were kept in a dry place until further analysis. They were then digested according to the method of Citation20,where 6 ml of Nitric acid, 2 ml of hydrochloric acid and 1 ml of hydrogen peroxides was added sequentially into a Teflon vessel with 0.25 gm of the dried sediment sample. The microwave digestion system (Coolpex, Preekem, China) was used for digestion. The digestion process and parameters are presented in Table S1. After the digestion of the sediment sample, the digested solution was put into a 50 ml centrifuge tube and washed with Milli-Q water three times and filled up to the 40 ml mark with Milli-Q water. From each sampling, location triplicate was digested and the solution was kept at 4°C for further analysis. After that, the total concentration of heavy metals (Cr, Mn, Ni, Cu, Zn, As, Cd, and Pb) were quantified by Inductively Coupled Plasma Mass Spectrometer (ICP-MS, Agilent, 7500ce, USA). To evaluate the accuracy of the method, standard reference materials (GBW07314, National Institute of Standard and Technology, China) were used. In addition, reagent blank, triplicate measurement was taken into account to verify and evaluate the accuracy of the method. The standard reference material was digested by the same methods and the recovery rate was from 90% to 106% of their designated values.
2.3. Assessment of sediment pollution and ecological risk indexes
2.3.1. Geo-accumulation index assessment
The geo-accumulation index (Igeo) is a widely used criterion to evaluate sediment contamination. Besides, Igeo indicates whether the enrichment of metals from the natural or anthropogenic origin. Igeo was proposed by Muller in 1969, to quantify the pollution level of metals in sediments and to compare the present concentration with geochemical background value. The Igeo was calculated using the following equation,
Here, Cn is the measured concentration of metal in the study area and Bn is the average geochemical background value of shale globally. The constant value of 1.5 is a correction factor to minimize the lithogenic variation of metals [Citation21]. The Igeo values were divided into seven categories such as Igeo < 0, Uncontaminated (UC); 0 ≤ Igeo < 1, Uncontaminated to moderately contaminate (UMC); 1 ≤ Igeo < 2, Moderately contaminated (MC); 2 ≤ Igeo < 3, Moderately to heavily contaminated (MHC); 3 ≤ Igeo < 4, Heavily contaminated (HC); 4 ≤ Igeo < 5, Heavily to extremely contaminated (HEC) and Igeo ≥ 5, Extremely contaminated (EC).
2.3.2. Pollution load index assessment
The pollution load index (PLI) assesses the estuarine/lagoon pollution quality with the combination of zones and site pollution data from the contamination factor (CF) [Citation22]. It can only assess the multi-elemental pollution level of certain ecosystems and their pollution trend. The PLI calculation formula is as follows:
Here, CF is the contamination factor of each metal and n is the number of metals studied in the sampling sediment. The CF is the ratio between the measured concentration of metal in sediment and the given background concentration of the same metal [Citation23–25] (Table S2). The PLI values can be classified as perfect site quality or no pollution (PLI < 1), baseline level of pollution (PLI = 1), deterioration of site quality (PLI > 1) [Citation22].
2.3.3. Potential ecological risk assessment methods
The potential ecological risk indexes (Eir, RI), adverse effect index (AEI) and effect range median (ERM) quotient were used to evaluate the possible adverse effect of heavy metals in sediment of Longhu Lake. AEI and mean EMR quotient are compared with the present concentration with Sediment Quality Guidelines (SQGs) data [Citation26; Citation1]. The potential ecological risk indexes indicate the sedimentological risk of heavy metals under the consideration of migration, transformation and toxicity of each or combined metals [Citation24]. The potential ecological risk factor (Eir) and risk index (RI) was calculated using the following equations,
where Tri is the toxic response coefficient of the given metals (e.g. Ni = Cu = Pb = 5, Mn = Zn = 1, Cd = 30, Cr = 2 and As = 10) and CF = contamination factor. The Eir was divided into the following categories: Eir < 40: low risk, 40 ≤ Eir < 80: moderate risk, 80 ≤ Eir < 160: considerable risk, 160 ≤ Eir < 320: high risk and Eir ≥ 320: very high risk [Citation24]. RI demonstrates the sum of all Eir in the studied ecosystem. RI was categorized into the following four levels: RI < 150: low risk, 150 ≤ RI < 300: moderate risk, 300 ≤ RI < 600: considerable risk and RI ≥ 600: Very high risk [Citation24].
Adverse effect index (AEI) is determined by the ratio of metal content in sediment and the value of same the metals in the SQGs. AEI can evaluate how much metals level exceed in the sediment samples compared with SQGs values [Citation1]. The AEI was calculated using the following equation:
where MCs is the concentration of metal in the sediment of the sampling areas, SQGs is any Sediment Quality Guideline value, such as threshold effect level (TEL), effect range low (ERL) etc. In this present study, ERL of SQGs values [Citation27,Citation28] were used to evaluate the AEI of heavy metals in the studied areas. ERL value of Mn was unavailable but still, this method remains the strongest index to identify the potential adverse effect of heavy metals to biota in the aquatic environment sediment [Citation1]. According to the classification, AEI was classified into two categories, AEI < 1; sediment metals concentration is not high enough to produce adverse effects on biota, AEI > 1; which means the concentration of metals could produce adverse effect on sediment-dwelling organisms [Citation1].
Adverse effect index (AEI) and SQGs evaluate the single metal impact on the aquatic ecosystems. But, in sediment, metals form in a mixture of heavy metals. So, it is very crucial to evaluate the combined effect of heavy metals on biota in aquatic environments/ecosystems [Citation26]. In this study, mean ERM quotient has been conducted to evaluate to the combined adverse effect on biota by using effect range median (ERM) values [Citation28] of SQGs. The mean EMR quotient was calculated using the following equation,
Where Cs is the metals concentration of sediment in the studied areas, ERMs is the ERM value of metal (s) and n is the number of metals. Seven metals were evaluated in this method. The mean ERM quotient value less than 0.1, 9% probability ability to be toxic, quotient value 0.11–0.5, 21% toxic probability, quotient value 0.51 − 1.50, 49% toxic probability and quotient greater than 1.50 suggest 76% probability ability to be toxic [Citation1,Citation26,Citation29,Citation30].
2.4. Total organic carbon (TOC) analysis
Dried sediment sample of 10 gm was placed into 50 ml of a glass beaker and soaked with 5% hydrochloric acid and stirred 24 repeatedly at the interval of every 6 h. Then, the samples were oven-dried at 80°C for 48 h. Drying sediment was grounded with mortar and pestle and sieved through 100 mesh nylon fiber [Citation18]. After that, the sediment TOC percentage was analyzed by the Elemental Analyzer (Elemental tar Vario, EL III, Germany).
2.5. Statistical analysis
The relationship between heavy metals and TOC was determined by Spearman correlation coefficient analysis. The multivariate principal component analysis was conducted to evaluate the common behaviour and sources of heavy metals. Statistical analyses were conducted by SPSS for Windows (SPSS, USA).
3. Results and discussion
3.1. Spatial distribution of heavy metals concentration in sediments
Considering all sampling locations, the average total concentration of heavy metals in Longhu was in the following decreasing order: Mn > Zn > Pb > Cu > Cr > As > Cd, respectively (Table S3). The concentration of total heavy metals was in the range of 36 mg/kg to 66.32 mg/kg for Cr, 1141 mg/kg to 2804.9 mg/kg for Mn, 19 mg/kg to 53.02 mg/kg for Ni, 60 mg/kg to 91.85 mg/kg for Cu, 290 mg/kg to 454.4 mg/kg for Zn, 8 mg/kg to 10.5 mg/kg As, 1.30 mg/kg to 2.30 for Cd, and 70 mg/kg to 107.16 for Pb, respectively (Table S3). The heavy metals concentration in different sampling sites were not distributed homogenously ().
The concentration of Cr, Cu, Ni, and As was greater in the upstream sampling site (S3) and was lowest in the inlet sampling site (S1), which was 1.84, 1.53, 2.76, and 1.31 times lower than the upstream concentration (). But, the highest concentration of Mn, Zn, Pb, and Cd was found in the inlet sampling site (S1) and the lowest concentration was found in the mid-stream sampling site (S5) and their concentration was 2.45, 1.56, 1.52, and 1.76 times lower than inlet site (). The concentration of Cu, Zn, Cd, and Pb exceeded in this lake in comparison with other lakes in China (Table S3) whereas the concentration of Cr, As and Ni was lower (Table S3). The average concentration of Mn, Zn, Cu and Cd was considerably higher than the world average shale, upper continental crust and the USEPA screening benchmarks of freshwater sediments (Table S3). The mean concentrations of Cu, Zn, Cd and Pb were about 2.10, 3.51, 8.00 and 2.50 times greater than the Chinese soil quality standard [GB 15,618–Citation28] (Table S3). Moreover, a comparison with China safe agricultural production standard suggests that Cr, Cu, Zn and Pb concentrations were greater in the studied sediment. This result indicates that this lake may not be ecologically safe for fisheries productions due to lower sediment quality [Citation18]. We recommend more research on the accumulation of heavy metals in fish tissues and trophic transfer in food webs.
3.2. Identification sources and pollution assessment of heavy metals
3.2.1. Assessment of geo-accumulation of heavy metals in sediments
The Igeo values of heavy metals of Longhu Lake are presented in . The negative Igeo values for (Igeo < 0) Cr, Ni and As of all the sampling sites () suggest that sediments of Longhu Lake are not contaminated by these metals and these metals are likely from the lithogenic sources [Citation13]. These Igeo values of Cr, Ni and As (Igeo < 0) are similar to the average values published in the recent review of Chinese lake sediment contamination [Citation5]. The Igeo values for Cu were less than 1 (0 ≤ Igeo < 1) of all sampling sites except S1 (Igeo < 0) indicates that sediments were uncontaminated to moderately contaminated by Cu and minimal anthropogenic input in this study area. A similar result was reported in Hongfeng Lake but lower Igeo class of Cu (Class 0 and 1) than average lake sediment pollution (Igeo class 0 to 3) in China [Citation5,Citation31]. In the lake sediments, the possible anthropogenic sources of Cu are from wastewater discharge, fungicides, boat hull paint, construction activities and industrial machinery [Citation5,Citation32]. Besides, metals melting factory emission was the possible sources of anthropogenic Cu in lake sediments [Citation31]. The Igeo values for Mn are in the class of 0 ≤ Igeo < 1 for most of the sampling sites except S3 (Igeo < 0) and S1 (1 ≤ Igeo < 2) indicates that sediments were uncontaminated to moderately contaminated by Mn and greater variation of their anthropogenic sources due to three Igeo classes. Similar anthropogenic input of Mn was reported in Dongting Lake China [Citation33]. In the Temsah lake, Mn pollution was due to the repairing and construction of boats [Citation34] but domestic wastage and sewage sludge are the main anthropogenic sources of Mn in Poyang Lake, China [Citation35]. Zn and Pb has similar Igeo class (1 ≤ Igeo < 2) of all the sampling sites indicates sediment was moderately contaminated by Zn and Pb. Similar result was reported in Poyang Lake sediments [Citation26]. But the average Igeo values for Zn (1.29) and Pb (1.53) were significantly greater than the average Chinese lake sediments Igeo values of Zn (−0.22) and Pb (−0.16) suggesting a considerable anthropogenic input of Zn and Pb in the studied areas sediments [Citation5].
In the urban areas, fungicides, painting agents, combustion of fossil fuels and industrial emissions are the major sources of the aquatic ecosystems [Citation13,Citation26]. In Lake Laihu, the discharge of industrial wastewater and fertilizer was the possible source of Zn and Pb in sediments [Citation32]. The Igeo values of Cd in the class of 1 ≤ Igeo < 2 and 2 ≤ Igeo < 3 indicates that the sediments were moderate to heavily contaminated by Cd. The heavily Cd contaminated sampling sites of S1 and S2 suggest that the main anthropogenic input of Cd in the sediments of Longhu Lake. Several studies reported similar geo-accumulation of Cd in lake sediments in China [Citation26; Citation31]. But, the average Igeo value of Cd (1.81) was higher than the average Chinese lake sediments Igeo value (1.64) suggesting a considerable contamination level of Cd in the studied area [Citation5]. In the urban aquatic ecosystems, the major possible sources of Cd may be industrial discharge, discarded domestic electronics, pesticides, and colour pigment etc [Citation18,Citation36].
Overall, in the study areas, the average Igeo values of heavy metals were in the order of Cd>Pb>Zn>Mn>Cu>As>Cr>Ni, respectively. However, it can be concluded that sediments of the Longhu are moderate to heavily contaminated by Zn, Cd and Pb.
3.2.2. Pollution load of heavy metals in sediments
The Pollution load index (PLI) indicates the multi-elemental pollution load in sediments to evaluate the quality of polluted sites, zones, or whole ecosystems [Citation22,Citation37]. In this study, PLI values are >1 in all sampling areas () which indicate that sediments are polluted with heavy metals and there has a ‘deterioration in site quality’ [Citation22,Citation38]. The highest PLI observed in S4 (mid-stream area) suggests greater anthropogenic load and poor site quality than other study sites (). Overall, the PLI for Longhu Lake (the nth root PLI of all studied sites) is 1.67 (PLI >1) suggests the considerable anthropogenic existence of heavy metals in these areas [Citation22]. The result provides information regarding the greater pollution load sites, which would be beneficial for the future management strategies for improving the quality of ecosystems [Citation22].
3.2.3 Identification of similar behaviour and sources of heavy metals
Principal component analysis (PCA) is a widely used multivariate analysis to evaluate the potential similarities, sources (anthropogenic and natural) and characteristics of heavy metals in sediments [Citation13,Citation17,Citation26,Citation33]. In this study, PCA was used to evaluate the possible sources (anthropogenic and natural) and behaviour of heavy metals. Before PCA, Kaiser-Meyer-Olkin (KMO) and Bartlett’s sphericity tests were conducted to identify the validity/adequacy of PCA [Citation39]. The KMO was 0.772 and the significance of Bartlett’s sphericity was 0 (less than 0.05) suggesting the analysis of PCA was suitable and there is a significant relationship among variables [Citation33]. PCA was conducted with varimax rotation and extracted the components that’s eigenvalue is greater than 1 and presented in and loading plots in .
Table 1. Component matrix data of first two components. In bolds, the highest loading
PCA extracts two components with eigenvalue >1 and which explained 91.50% of the total variance of the data sets (). It shows that 51.43% of the total variance was explained by the first component (PC1) and 40.06% was explained by the second component (PC2). Mn, Zn, Cd and Pb had greater positive loading indicating that these metals could be similar in behaviour and anthropogenic sources in Longhu sediments. This is also supported by the higher contamination factor (CF) of Mn (1.46–3.08), Zn (3.14–4.67), Cd (4.47–7.26), and Pb (3.66–5.21) in the studied sediments (Table S2). Several studies indicate that in the urban areas, these metals mostly input from the local point and non-point sources, wastewater discharge, runoff and atmospheric discharges [Citation13,Citation18,Citation36]. These metals are highly enriched in inlet areas which also indicate considerable point sources in Longhu sediments. After the treatment of wastewater, discharge of this water through the inlet area to Longhu suggests the main source of pollutants into this lake. Therefore, Cr, Ni, Cu and As shows higher positive loading in the second component. This suggests that these metals may be imported from natural sources in the study area [Citation39]. This result is supported by the geo-accumulation index values where Cr, Ni and As showed >0 and for Cu >1 () in the study area. Overall, PCA analysis suggests that Mn, Zn, Cd, and Pb are the main anthropogenic input of heavy metals in the sediments of Longhu Lake.
3.4. Assessment of ecological risk of heavy metals
3.4.1. Potential ecological risk
Potential ecological risk factor (Eir) and risk index (RI) are the widely used methods to evaluate the risk of each metal/multiple metals in the aquatic environment [Citation31,Citation35]. These indexes are considered as the toxic response of biological communities to toxic substances in sediments and their possible combined effects on ecosystems [Citation31]. The Eir and RI were used to evaluate the possible ecological risk and possible regulatory attention for heavy metals in Lonhgu Lake. The average of Eir was in the order of Cd > Pb > Cu > As > Zn > Ni > Mn > Cr, respectively (). Pb, As, Cu, Zn, Ni, Mn, and Cu Eir values were less than 40 at all sampling sites of the study area () suggesting the low ecological risk of these metals to aquatic organisms [Citation24]. Similar ecological risks of these metals were reported in Hongfeng and Liuye Lake in China [Citation26; Citation31]. In the comprehensive overview of Chinses lake sediments ecological risk factor was similar (Eir < 40) with the results of the present study [Citation5]. In addition, it is well known that sediment residual fraction of metals are less bioavailable and toxic to aquatic biota [Citation21].
Table 2. Potential ecological risk indexes (Eir and RI) values of heavy metals in sediments of Longhu Lake China
Eir values for Cd showed 134.15 to 217.81 indicating considerable ecological risk to aquatic organisms. Several studies reported that Cd is the higher risk metals among studied metals in lake sediments [Citation31,Citation32]. The average Cd Eir value (218.1) for Chinese lake sediments was higher than that in this study [Citation5], but similar with the maximum Eir value (217.81) of the sampling site S1.
Metals are present in a combination of mixtures in sediment. As a result, RI method has been conducted to evaluate the sensitivity of aquatic organisms/community to mixture of metals in sediment [Citation24]. It also indicates the overall ecological risk of metals in the specific environment/sites. The mean RI values were in the range of 178.46 to 267.53 in all sampling sites suggesting the considerable risk of heavy metals in terms of combination effects of metals mixtures [Citation24]. According to average RI values, the sampling sites risk is in the order of S1> S2> S6> S4> S7> S3> S5, respectively ().
The contribution of Cd Eir values in RI was in the range of 75% to 81% suggesting that Cd was the major risk to sediment biota as well as the surrounding aquatic environment in Longhu Lake. This result also indicates that Cd should be given special attention in Longhu Lake [Citation24]. The Cd emission from coal combustion has increased from 31.14 tons to 261.52 tons from 2008 to 2014 in China [Citation5]. In addition, the higher RI values of Cd were due to higher sink and sedimentology toxic factor [Citation24]. The Eir values of Pb were contributed to RI values in the range of 9–10% in the sampling areas, whereas all other metals contributed less than 5%. Overall, Eir values showed the low ecological risk of studied metals to sediment dweller organisms as well as in the surrounding aquatic environment except for Cd which contributed to high ecological risk. But the combined mixtures of heavy metals (RI) suggest a considerable effect on benthic organisms [Citation24]. So, Cd pollution should receive more attention than other metals.
3.4.2. Adverse effect index and mean ERM quotient
Adverse effect index (AEI) was used to evaluate how many times of sediment metals concentration were exceeded to the Sediment Quality Guidelines (SQGs) such as threshold effect (TEL), which indicates the toxic effect on biota in the aquatic ecosystems [Citation1]. In this study, AEI> 1 for all the studied metals suggests that these metals are likely to have a toxic effect on the sediment dwellers organisms. In addition, Cd, Zn and Pb have higher AEI values than other metals which () suggests that these three metals need to be given more attention for regulation and management of Longhu Lake [Citation1]. Besides, higher AEI values of Cd, Zn and Pb in the inlet areas sediments suggest that this area could be the possible source of these metals. Therefore, the mean ERM quotient values are in the range of 0.55 to 0.59 in all the sampling areas () indicates 49% probability of these seven metals to have toxic biological effects in this ecosystem [Citation1]. And the maximum biological effect was observed in S2 sampling site indicating that inlet areas of Longhu could be possible hotspots of heavy metals.
3.5. TOC in sediments and their relationship with heavy metals
In the aquatic ecosystems, total organic carbon (TOC) is the crucial component of sediments which provides the binding sites of heavy metals. In addition, TOC has a significant effect on the adsorption and desorption of heavy metals including reducing bioavailability and toxicity through the formation of organometallics complexes [Citation21]. This analysis shows that TOC concentration fluctuated from 1.63% to 2.82%, with a mean value of 2.36% (Table S4). The highest TOC (2.82%) was observed in the mid-stream sampling site S5 (Table S4). Yangtze flood plain (1–2%) [Citation40,Citation41] and Tibetan Plateau lakes (0.22–2.2%) TOC [Citation42] are relatively similar with these results, but relatively lower than Yunnan–Guizhou Plateau (5–13%) lakes [Citation41,Citation43]. In the shallow lakes, frequent resuspension and turbulence lead to less accumulation of TOC in sediments. Therefore, the warm and humid climate could enhance the decomposition of POC in water column and TOC in sediments, resulting in less accumulation in surface sediments [Citation41]. Several studies indicated that TOC in shallow lakes are mostly from the allochthonous sources [Citation41,Citation44] whereas, in deep lakes, TOC is attributed from autochthonous sources such as planktonic remains and faces [Citation41,Citation45]. In the current lake, planktonic sources of TOC are mostly captured as food by fishes, therefore resuspension of sediments by filter-feeding (e.g. Silver Carp and Bighead Carp) and omnivorous fishes (e.g. Mud Carp and Common Carp) could be another reason for less accumulation of TOC in surface sediments. In these circumstances, terrestrial and submerged plants are most likely the sources of TOC in sediments [Citation41]. Therefore the inlet area has the lowest TOC in sediments due to the higher energy of flowing water [Citation46]. Better hydrodynamic conditions of the lake such as resuspension, waves, and higher energy (as drinking water pumping) could be the possible reasons of lower TOC accumulation in sediments [Citation41,Citation46].
To understand the relationship between heavy metals and TOC in sediments, a spearmen correlation was conducted, and the correlation coefficient of TOC and studied heavy metals are presented in . A significant positive correlation was observed between heavy metals pairs, Ni – Cr, Cu – Cr, Cu – Ni, Zn – Mn, As – Cr, As – Ni, As – Cu, Cd – Mn, Cd – Zn, Pb – Mn, Pb – Zn and Pb – Cd (). Several studies [Citation32,Citation35] indicate that the factors of positive correlations between heavy metals, these positive factors probably derived from the same sources, identical characteristics, similar transport and geochemical behaviours. The higher positive correlation of Zn, Cd and Pb may suggest that these metals are probably similar anthropogenic sources in the study area [Citation39]. This is confirmed by greater Igeo values (1.07–2.28) of these metals. Combustion of fossil fuel, painting of boats, wastewater discharge, fungicides, and atmospheric deposition, could be the possible sources of these metals in the studied areas. Higher positive correlation of Mn suggests anthropogenic input in the study area but may be from different sources than Zn, Cd and Pb. The significantly higher positive correlation of Cr, Ni, As, and Cu suggest that these metals could be from the natural sources in the studied sediment [Citation39]. Igeo values of these metals suggest that these metals are geogenic input in the sediments of Longhu. In addition, these metals showed a significant negative correlation with Mn, Zn, Cd and Pb which indicates that they may be from different sources. Furthermore, the coefficient has showed that there is no significant positive correlation between heavy metals and TOC in sediments (). This result suggests that TOC is not the controlling factor of heavy metals accumulation in sediments of Longhu Lake [Citation47].
Table 3. Spearman correlation coefficient values of heavy metals and TOC in Longhu Lake
4. Conclusions
The study aimed to identify sources, distribution, pollution status and risk of heavy metals in lake sediments based on different metal geochemical indexes and sediment quality guidelines (SQGs) criteria. The concentration results suggested that sediments in the studied areas were polluted by heavy metals and there is strong variation in concentration pattern in different sampling areas. The highest concentrations of the metals were in the inlet (S1) and upstream areas (S3). The concentration of Cu, Zn, Cd and Pb were considerably higher than Chinese sediment quality standards. According to Igeo, sediments were moderate to heavily polluted by Zn, Cd and Pb. There was a 49% incidence rate of adverse biological effects of lake sediments according to mean EMR quotient. The potential ecological risk indicates that Cd was associated with high risks and other metals had considerable risk. These metals can accumulate in the lake food chain and serious threats to the aquatic ecosystems. The PCA analysis indicates that Mn, Zn, Cd and Pb were originated mainly from anthropogenic sources such as urban domestic sewage, runoff, boat riding and construction activities. In order to prevent heavy metals pollution in lake Longhu, further study should be conducted for the source identification and implement measures to reduce wastewater discharge into this lake. Therefore, speciation of heavy metals and their risk in aquatic biota should be conducted. In the future, more attention should be given to the management and pollution control of heavy metals especially Zn, Cd and Pb. The study highlights the sources, distribution, pollution status and risk of heavy metals and can be used as a future reference for the management of heavy metals pollution of lakes.
Supplemental Material
Download MS Word (24.4 KB)Acknowledgments
“This study was funded by the National Key Research and Development Program of China (Grant no: 2016YFCO502904 and 2018YFC1406306)”. The authors wish to thank the Longhu Lake administration office for assistance during sampling work.
Disclosure statement
No potential conflict of interest was reported by the authors.
Supplementary material
Supplementary data for this article can be accessed here.
Additional information
Funding
References
- Saher NU, Siddiqui AS. Occurrence of heavy metals in sediment and their bioaccumulation in sentinel crab (Macrophthalmus depressus) from highly impacted coastal zone. Chemosphere. 2019;221:89–98.
- Wang S, Wang W, Chen J, et al. Geochemical baseline establishment and pollution source determination of heavy metals in lake sediments: a case study in Lihu Lake, China. Sci Total Environ. 2019;657:978–986.
- Li Y, Zhou S, Zhu Q, et al. One-century sedimentary record of heavy metal pollution in western Taihu Lake, China. Environ Pollut. 2018;240:709–716.
- Uddin MM, Huang L, Bin X. Mini review spatial and temporal changes of heavy metals in coastal mangrove sediment in China: review of present status. J Biol Sci. 2019;19(4):314–322.
- Xu Y, Wu Y, Han J, et al. The current status of heavy metal in lake sediments from China: pollution and ecological risk assessment. Ecol Evol. 2017;7(14):5454–5466.
- Guo B, Liu Y, Zhang F, et al. Heavy metals in the surface sediments of lakes on the Tibetan Plateau, China. Environ Sci Pollut Res. 2018;25(4):3695–3707. .
- Saeedi M, Hosseinzadeh M, Rajabzadeh M. Competitive heavy metals adsorption on natural bed sediments of Jajrood River, Iran. Environ Earth Sci. 2011;62(3):519–527.
- Lin Q, Liu E, Zhang E, et al. Spatial distribution, contamination and ecological risk assessment of heavy metals in surface sediments of Erhai Lake, a large eutrophic plateau lake in southwest China. Catena. 2016;145:193–203.
- Fang T, Lu W, Li J, et al. Levels and risk assessment of metals in sediment and fish from Chaohu Lake, Anhui Province, China. Environ Sci Pollut Res. 2017;24(18):15390–15400. .
- Jiang Y, Ye Y, Guo X. Spatiotemporal variation of soil heavy metals in farmland influenced by human activities in the Poyang Lake region, China. Catena. 2019;176:279–288.
- Karthikeyan P, Vennila G, Venkatachalapathy R, et al. Assessment of heavy metals in the surface sediments of the Emerald Lake using of spatial distribution and multivariate techniques. Environ Monit Assess. 2018;190(11):668. .
- Li HB, Gu Y, Wang R, et al. Contamination, fractionation and biological risk related to metals in surface sediments from the largest deep freshwater Lake in China. Arch Environ Contam Toxicol. 2017;72(1):78–87.
- Uddin MM, Xie B, Peng G, et al. Heavy metal pollution status, spatial distribution and associated ecological risks within sediments of yundang lagoon catchment in xiamen, china, after 30 years continuous ecological rehabilitation. Hum Ecol Risk Assess An Int J. 2020;27(2):1–18.
- Li T, Liu Y, Lin S, et al. Soil pollution management in china: a brief Introduction. Sustain. 2019;11:1–15.
- Li Y, Liu F, Zhou X, et al. Distribution and ecological risk assessment of heavy metals in sediments in chinese collapsed lakes. Polish J Environ Stud. 2017;26(1):181–188. .
- Lu H, Zhuang H. The study of phytoplankton and the evaluation of water quality of the jinjiang long lake. J Fujian Teach Univer. 1997;13:86–90.
- Zhang Z, Huang Z, Li J, et al. Evolution of the environmental geology of longhu lake in fujian province during the past forty-odd years. Acta Geol Sinia. 2004;78:569–574.
- Chen C, Lu Y, Hong J, et al. Metal and metalloid contaminant availability in yundang lagoon sediments, xiamen bay, china, after 20 years continuous rehabilitation. J Hazard Mater. 2010;175(1–3):1048–1055. .
- Devarajan N, Laffite A, Graham ND, et al. Accumulation of clinically relevant antibiotic-resistance genes, bacterial load, and metals in freshwater lake sediments in Central Europe. Environ Sci Technol. 2015;49(11):6528–6537. .
- USEPA 1996. Microwave assisted acid digestion of siliceous and organically based matrices OHW, Method 3052.
- Guan J, Wang J, Pan H, et al. Heavy metals in yinma river sediment in a major phaeozems zone, northeast china: distribution, chemical fraction, contamination assessment and source apportionment. Sci Rep. 2018;8(1):12231. .
- Tomlinson DL, Wilson JG, Harris CR, et al. Problems in the assessment of heavy-metal levels in estuaries and the formation of a pollution index. Helgoländer Meeresuntersuchungen. 1980;33(1–4):566–575.
- Huang L, Pu X, Pan JF, et al. Heavy metal pollution status in surface sediments of swan lake lagoon and rongcheng bay in the northern yellow sea. Chemosphere. 2003;93(9):1957–1964.
- Hakanson L. an Ecological Risk Index for Aquatic Pollution Control. A Sedimentological Approach. Water Res. 1980 September 18;14(8):975–1001. 2020
- Javed T, Ahmad N, Mashiatullah A. Heavy metals contamination and ecological risk assessment in surface sediments of namal lake, pakistan. Polish J Environ Stud. 2018;27(2):675–688.
- Dai L, Wang L, Li L, et al. Multivariate geostatistical analysis and source identification of heavy metals in the sediment of Poyang Lake in China. Sci Total Environ. 2018;621:1433–1444.
- Liu Y, Liu G, Wang J, et al. Spatio-temporal variability and fractionation of vanadium (V) in sediments from coal concentrated area of Huai River Basin, China. J Geochem Explor. 2017;172:203–210.
- Long ER, MacDonald DD, Smith SL, et al. Incidence of adverse biological effects within ranges of chemical concentrations in marine and estuarine sediments. Environ Manage. 1995;19(1):81–97.
- Long ER, MacDonald DD, Severn CG, et al. Classifying probabilities of acute toxicity in marine sediments with empirically derived sediment quality guidelines. Environ Toxicol Chem. 2000;19(10):2598–2601.
- Müller G. Index of geoaccumulation in sediments of the Rhine River. Geojournal. 1969;2:108–118.
- Wang G, Hu X, Zhu Y, et al. Historical accumulation and ecological risk assessment of heavy metals in sediments of a drinking water lake. Environ Sci Pollut Res. 2018;25(25):24882–24894. .
- Yang JF, Xu P, Wu SL, et al. Distribution, sources and ecological risk assessment of heavy metals in the surface sediments of lake liuye and its adjacent waters, China. J Radioanal Nucl Chem. 2018;318(2):1131–1142. .
- Zhang Y, Tian Y, Shen M, et al. Heavy metals in soils and sediments from dongting lake in China: occurrence, sources, and spatial distribution by multivariate statistical analysis. Environ Sci Pollut Res. 2018;25:13687–13696.
- Soliman NF, Younis AM, Elkady EM. An insight into fractionation, toxicity, mobility and source apportionment of metals in sediments from el temsah lake, suez canal. Chemosphere. 2019;222:165–174.
- Zhang H, Jiang Y, Ding M, et al. Level, source identification, and risk analysis of heavy metal in surface sediments from river-lake ecosystems in the Poyang Lake, China. Environ Sci Pollut Res. 2017;24(27):21902–21916.
- Yan C, Li Q, Zhang X, et al. Mobility and ecological risk assessment of heavy metals in surface sediments of Xiamen Bay and its adjacent areas, China. Environ Earth Sci. 2010;60(7):1469–1479.
- Taylor SR, McLennan SM. The continental crust: its composition and evolution. USA; 1985.
- Turekian KK, Wedepohl KH. Distribution of the elements in some major units of the earth’s crust. Geol Soc Am Bull. 1961;72(2):175–192.
- Jamshidi-Zanjani A, Saeedi M. Metal pollution assessment and multivariate analysis in sediment of Anzali international wetland. Environ Earth Sci. 2013;70(4):1791–1808.
- Dong X, Anderson NJ, Yang X, et al. Carbon burial by shallow lakes on the Yangtze floodplain and its relevance to regional carbon sequestration. Glob Change Biol. 2012;18(7):2205–2217.
- Yu ZT, Wang XJ, Zhang EL, et al. Spatial distribution and sources of organic carbon in the surface sediment of Bosten Lake, China. Biogeosciences. 2015;12(22):6605–6615. .
- Wang Y, Zhu L, Wang J, et al. The spatial distribution and sedimentary processes of organic matter in surface sediments of nam co, central tibetan plateau. Chin Sci Bull. 2012;57(36):4753–4764.
- Wu F, Xu L, Sun Y, et al. Exploring the relationship between polycyclic aromatic hydrocarbons and sedimentary organic carbon in three Chinese lakes. J Soil Sediment. 2012;12(5):774–783.
- Sifeddine A, Meyers PA, Campello R, et al. Delivery and deposition of organic matter in surface sediments of Lagoa do Caco.. J Paleolim. 2011;45(3):385–396. .
- Shanahan TM, McKay N, Overpeck JT, et al. Spatial and temporal variability in sedimentological and geochemical properties of sediments from an anoxic crater lake in West Africa: implications for paleoenvironmental reconstructions. Palaeogeogr Palaeocl. 2013;374:96–109.
- Shi X, Zhang W. Experimental study on release of heavy metals in sediment under hydrodynamic conditions. IOP Conf Ser Earth Environ Sci. 2018;208:12040.
- Chakraborty P, Sarkar A, Vudamala K, et al. Organic matter — a key factor in controlling mercury distribution in estuarine sediment. Mar Chem. 2015;173:302–309.