ABSTRACT
Aniline is a toxic organic pollutant and raw material in industries. In this study, the start-up of sequencing batch reactor for treating aniline wastewater was assessed and functional microbial clusters were analyzed after enrichment of denitrifying phosphate-accumulating organisms (DNPAOs). The aniline degradation and subsequent removal of NH4+-N mainly happened in aerobic condition, and denitrification was conducted in both anaerobic and anoxic conditions. Besides aerobic phosphorus uptake, phosphorus accumulation also occurred via DNPAOs in anoxic condition utilizing nitrate as electron acceptor. The removal efficiencies of COD, NH4+-N, TN, and TP was over 91.6, 67.1, 65.0, and 79.9% respectively. Proteobacteria, Bacteroidetes, and Firmicutes were found to be predominant at phylum level treating aniline wastewater. Blastocatella, Ferruginibacter and Clostridium were predominant strains involved in aniline degradation and Pseudomonas was enriched in the presence of aniline. Aeromonas, Acinetobacter, and Comamonas may play key roles in nitrogen removal and phosphorus accumulation with tolerance of aniline.
Introduction
Removal of aromatic compounds is one of the most important aspects in industrial wastewater treatment. It has also been found that aniline is toxic to living beings since it is a probable human carcinogen and shows inhibition of enzyme activity [Citation1]. Effluents containing aniline are generated through a number of industrial manufacturing activities such as production of plastics, herbicides, isocyanates, rubber processing chemicals, dyes and pigments, agricultural chemicals and pharmaceuticals [Citation2,Citation3]. As an important synthetic intermediate in many different fields of applications, aniline has been increasingly released to natural environments along with the rapid development of these industries. Since aniline has benzyl structure, it can remain in environment for a long period [Citation4]. Several bacteria groups have extraordinary capability of utilizing aniline as the sole source of carbon, nitrogen, and energy [Citation5]. However, the concentration of NH4+-N increases along with the removal of aniline since at the first stage of aniline biodegradation, aniline which contains an amino group is transformed into catechol by aniline dioxygenase with presence of oxygen, and leads to the release of NH4+-N to the wastewater treatment system [Citation6].
Nitrogen and phosphorus are two common contaminants. High concentration of nitrogen can be detrimental to the environment and human health, and the existence of large amounts of ammonium and phosphorus leads to eutrophication [Citation7,Citation8]. Therefore, nitrogen and phosphorus removal from wastewater has long been an important issue. Conventional biological nitrogen removal processes mainly include nitrification and denitrification [Citation9,Citation10]. As for aerobic nitrification, NH4+-N can be transformed to NO2–N by ammonia-oxidizing bacteria and/or be further converted to NO3–N by nitrite-oxidizing bacteria. Denitrification is generally carried out by heterotrophic bacteria with external organic carbon source under anoxic condition. During this process, NO2–N and/or NO3–N can be transformed to nitrogen gas [Citation11,Citation12]. In recent years, several reports have mentioned simultaneous nitrification and denitrification in sequencing batch reactors (SBRs) through successive alternation of aerobic and anoxic reactions [Citation13]. By this way, there is no need to add another anoxic tank as compared with traditional nitrogen removal systems. Conventional phosphorus removal process is performed by anaerobic phosphorus release and aerobic phosphorus uptake since ordinary polyphosphate-accumulating organisms (PAOs) can only use oxygen as electron acceptor [Citation14]. Denitrifying phosphorus removal, which is regarded as one of promising biological nutrient removal technologies, is capable of incorporating nitrogen and phosphorus reduction concurrently [Citation15]. The denitrifying dephosphatation as an alternative to traditional nutrients reduction, is performed by denitrifying phosphate-accumulating organisms (DNPAOs). This group of microorganisms can remove phosphorus in activated sludge systems by utilizing nitrate instead of oxygen as electron acceptor. When nitrite concentration is below inhibition level, it may also be used as electron acceptor in anoxic process. It has been found that denitrifying phosphorus removal systems could not only reduce oxygen requirement and sludge production, but also avoid competition for organic carbon between denitrifiers and PAOs with certain amounts of organics, which are promising in nitrogen and phosphorus removal [Citation16].
Due to biodegradation potential of various substrates, microbial consortia in biological treatment systems are responsible for reduction of contaminants. The study on microbial diversity especially relative abundances of clusters in bioreactors with variation of working conditions can lead to better understanding of particular taxonomic groups involved in pollutants removal. However, to our knowledge, the discussion of microbial community in anaerobic/aerobic/anoxic (A/O/A) bioreactor realizing simultaneous removal of aniline, nitrogen and phosphorus from aniline wastewater has yet to be documented. In this work, the SBR was started with anaerobic/anoxic/aerobic (A/A/O) mode in order to enrich DNPAOs. The aniline-containing wastewater was treated in the SBR under A/O/A condition after cultivation of DNPAOs. During the whole process, the removal performance of nutrients and aniline was investigated. Furthermore, the microbial structure was determined by high-throughput sequencing based on Illumina MiSeq system. This study focused not only on aniline reduction but also on simultaneous removal of nitrogen generated from aniline biodegradation. Phosphorus was also removed in the system. The present study tried to emphasize the promising treatment process for simultaneous removal of aniline, nitrogen and phosphorus, and the key functional microbial groups during the start-up of the system, and to provide new sights in aniline wastewater biological treatment systems.
Materials and methods
Reactor and wastewater
A lab-scale cylindrical SBR system with an effective volume of 9 L and a height/diameter ratio of 6.7 was applied in the present study. The operating temperature of the SBR was controlled by using water bath. The influent was introduced at the top of the reactor during the feeding of each cycle. Aeration was supplied to the central bottom of the SBR. Mechanical stirring was also applied during anaerobic and anoxic phases of each cycle to maintain the sludge under suspension condition. The sequence of the SBR was controlled by programmable digital timers with a volume exchange ratio of 50%.
Seed sludge of the SBR was collected from a local water reclamation plant in Wuhan, China. For DNPAOs enrichment stage, synthetic wastewater was prepared containing the following components: CH3COONa 384.4 mg L−1, NH4Cl 95.54 mg L−1, NaNO3 30.36 mg L−1, KH2PO4 21.9 mg L−1, K2HPO4 · 3H2O 36.8 mg L−1. 1 mL L−1 of trace element solution was also added into the synthetic wastewater, which (per liter) was composed according to a published report [Citation17]. After the SBR showed stable removal performance on nitrogen and phosphorus, the wastewater was shifted to the targeted aniline-rich wastewater investigation of its removal performance. Meanwhile, the concentrations of CH3COONa and NH4Cl in the influent were adjusted accordingly to maintain a designed organic loading rate (OLR) and C/N ratio, respectively, throughout the whole experimental period.
Setup and operation of the SBR
The entire experimental period of the SBR system was assessed for 100 d, which consisted of three stages, namely DNPAOs enrichment stage (stage 1), enhanced nutrient reduction stage (stage 2) and aniline-rich wastewater treatment stage (stage 3), respectively. During stage 1, DNPAOs were enriched for 30 d under A/A/O mode. Subsequently, the operation mode of the SBR was shifted to A/O/A in stage 2, and nutrient (mainly nitrogen and phosphorus) removal performance was investigated during day 31–60 under two OLRs. Further, in stage 3 (day 61 to 100), the SBR performances on both aniline and nutrient removal from aniline wastewater were assessed. The detailed operating conditions of the system is summarized in .
Table 1. Operating conditions of the SBR
During stage 1, each batch cycle of the system contained a total of 6 h, including 5.5 h of reaction time. The operating phase distribution of the batch cycle was 5 min of feeding, 120 min of anaerobic agitation, 90 min of anoxic agitation, 120 min of oxic (aeration), 20 min of settling and 5 min of decant. At the beginning of A/A/O mode, the concentrations of mixed liquor suspended solids (MLSS) and 30 min sludge volume index (SVI30) were 4620 mg L−1 and 58.2 mL g−1, respectively. The operating temperature of the system was kept at 30 ± 2°C, while the pH was maintained between 6.0 and 7.0.
At the beginning of stage 2 (day 31), the operation of the SBR was shifted to A/O/A mode, since anoxic condition is known to be beneficial for denitrifying process and in turn may enhance total nitrogen (TN) reduction. The 6-h batch cycle during stages 2 and 3 consisted of 5 min of feeding, 120 min of anaerobic agitation, 120 min of oxic (aeration), 90 min of anoxic agitation, 20 min of settling and 5 min of decanting. The dissolved oxygen (DO) concentration, pH and temperature of the SBR were kept consistent with stage 1. In addition, during stages 2 and 3, the mixed liquor in the system was sampled on a daily basis, where concentrations of aniline, COD, NH4+-N, NO2–N, NO3–N, TN as well as total phosphorus (TP) were tested. The MLSS of the SBR was adjusted periodically to be kept within a range of 4200 ± 300 mg L−1.
Batch tests for DNPAOs assessment
Phosphorus uptake batch tests were carried out on day 30 (end of stage 1) to determine the proportion of DNPAOs in the sludge. In brief, the sludge was collected from the SBR at end of one cycle and washed with deoxidized water to remove the contaminant residues. The batch tests consisted of either sequential anaerobic and aerobic phases for determination of phosphorus uptake activity caused by PAOs, or sequential anaerobic and anoxic phases for phosphorus uptake activity caused by DNPAOs. The proportion of DNPAOs among PAOs could be estimated by the ratio of the specific anoxic phosphorus uptake rate divided by the specific aerobic phosphorus uptake rate. During anaerobic condition, acetate (initial COD concentration of ~300 mg L−1) were added into the washed sludge to provide organic substrate for phosphorus release. Nitrogen gas purging was supplied in this phase to ensure anaerobic condition. After 120 min, the sludge was settled and rinsed several times with deoxidized water to remove the remaining organics. Subsequently, the sludge was separated into two equal parts. One part was subjected to aerobic phase with DO concentration of 5.5 ± 0.5 mg L−1 and other part was subjected to anoxic phase with supplement of ~30 mg L−1 of NO3–N. Phosphorus was added to both the sludge parts before this phase (initial concentration of 10 mg L−1). The aerobic/anoxic operation lasted for 120 min. During this period, the initial and final mixed liquor samples were collected to calculate the specific phosphorus uptake rates. The batch tests were conducted at the temperature of 30 ± 2°C.
Microbial community analysis
Sludge samples were collected from the SBR during the operation to investigate the microbial community succession in the system. Three samples, namely S1, S2, S3, were obtained from day 1, 60 and 100, representing the sludge from seeding, enhanced nutrient reduction stage and aniline wastewater treatment stage, respectively. Total genomic DNA of the sludge samples was extracted by using PowerSoil DNA extraction kit (MO BIO Laboratories, Inc., USA). The integrity, purity and concentration of the DNA were monitored by Nanodrop® 1000 spectrophotometer (Thermo Fisher Scientific, USA). Universal primers for amplification were 338 F (5ʹ- ACTCCTACGGGAGGCAGCA-3ʹ) and 806 R (5ʹ-GGACTACHVGGGTWTCTAAT-3ʹ). Polymerase chain reaction was conducted according to a published research [Citation17]. The gene amplicons of three sludge samples were sequenced by Illumina MiSeq platform (PE300, USA). The sequences were analyzed on Majorbio I-Sanger Cloud Platform (www.i-sanger.com).
Analytical methods
During the whole experiment, water samples were filtered immediately after taken from the system by using 0.45-μm filters prior to analyses. The concentrations of aniline, COD, NH4+-N, NO2–N, NO3–N, TN, and TP were measured according to the standard methods [Citation18]. The nitrogen removal rate via bio-assimilation was calculated according to a published report [Citation19]. It can be calculated by (CTN1-CTIN1)/CTN0, where CTN0 and CTN1 represent initial and final concentration of TN, respectively, and CTIN1 represents final concentration of inorganic nitrogen (TIN). DO and temperature in the reactor were monitored by DO meter (YSI550A, YSI Inc., USA). The pH of the wastewater in the system were monitored by pH meter (PHS-3 C, Shanghai INESA Scientific Instrument Co., Ltd., China). MLSS and SVI30 were tested as described in a previous research [Citation20]. All the samples were analyzed in triplicates.
Results and discussion
DNPAOs enrichment
DNPAOs which could remove phosphorus by utilizing nitrate and/or nitrite instead of oxygen as electron acceptor to achieve simultaneous reduction of nitrogen and phosphorus [Citation15], was enriched before treating the target wastewater. DNPAOs was successfully accumulated in the reactor initially operated under A/A/O mode. During this stage the average removal efficiencies of COD, NH4+-N, TN, and TP were 88.4 ± 1.5, 91.3 ± 2.2, 73.8 ± 3.8, and 95.2 ± 2.5%, respectively (data not shown). To assess the enrichment efficiency, batch tests were performed at the end of stage 1 (day 30) to estimate the proportion of DNPAOs within all PAOs. After phosphorus was released in anaerobic condition, the specific anoxic/aerobic phosphorus uptake rates were measured and shown in . Since DNPAOs could achieve phosphorus removal under anoxic condition without any extracellular organic substrate [Citation20], DNPAOs were responsible for the phosphorus uptake in anoxic phase of the batch tests in the present study. The high ratio of the specific anoxic phosphorus uptake rate and aerobic phosphorus uptake rate obtained from the batch tests indicated that there was a large proportion of DNPAOs in the system.
Table 2. Specific phosphorus uptake rates in batch test
Performance of the SBR during enhanced nutrient reduction stage
The operating mode of the SBR was shifted to A/O/A mode at the beginning of stage 2, since anoxic condition could contribute to denitrification which could effectively reduce the NO3–N generated from the oxic phase. During stage 2, two different OLRs were evaluated. The removal performances of COD, NH4+-N, TN, and TP are presented in . The COD concentration in the effluent ranged from 13.9 to 47.3 mg L−1 with average removal efficiency of 89.8 ± 3.5% when the OLR was kept at approximately 0.6 kg m−3 d−1 (from day 31 to 45). Subsequently, the OLR of the system was increased to 1.2 kg m−3 d−1 during day 46 to 60. It was found that the increase of OLR did not show remarkable impact on organic removal performance, where the removal efficiency of COD under higher OLR was still over 87.3%. The average effluent concentration was 31.1 ± 9.5 and 33.3 ± 13.5 mg L−1 when the organic loading rate was 0.6 and 1.2 kg m−3 d−1, respectively. Within one typical cycle, the removal efficiency of COD during aeration can be over 70%, indicating the active metabolism of heterotrophic bacterial consortia and the sufficient aeration time in aerobic phase of the present system.
Figure 1. Pollutant removal performance in the system during enhanced nutrient reduction stage (Stage 2)
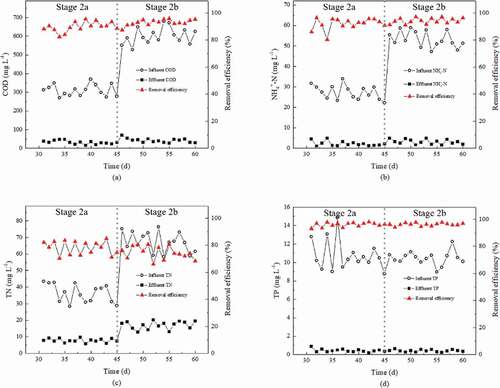
The system also had good removal ability of nitrogen. The effluent concentration of NH4+-N was below 4.9 mg L−1 even when initial NH4+-N concentration was over 55 mg L−1. The average removal efficiency of NH4+-N with initial concentration of 27.3 ± 3.3 mg L−1 and 53.3 ± 3.8 mg L−1 was 92.0 ± 4.1 and 94.2 ± 2.0%, respectively. This was attributed to nitrification process by ammonia oxidation bacteria in aerobic phase. The TN removal efficiency during stage 2 varied from 65.1 to 85.0% with the effluent TN concentration ranging from 5.8 to 20.1 mg L−1. The average TN removal efficiency was 78.1 ± 4.8 and 74.2 ± 4.6%, respectively, when TN concentration in influent was 36.3 ± 5.3 and 67.4 ± 5.9 mg L−1. This was due to both denitrification in the first anaerobic stage by denitrifiers and denitrifying phosphorus accumulation in anoxic phase by DNPAOs. With regards to TP during 30-day period, the outlet TP concentration after 6 h treatment was below 0.88 mg L−1 and the average removal efficiency was 96.1 ± 1.3%. The excellent removal efficiencies of nitrogen and phosphorus in this stage were consistent with some other biological systems for nutrients removal [Citation21,Citation22].
System performance during aniline wastewater treatment
Aniline-containing wastewater is wide-spread and harmful to the environment. Without proper treatment, the NH4+-N released from aniline degradation could also be accumulated. Aniline-containing influent was treated in this stage (A/O/A mode) and the simultaneous removal of aniline, nitrogen and phosphorus in the SBR was found. Two concentrations of aniline with a total of 40-day operation were performed. It was observed that the concentration of aniline could be under detection limit after 6 h cycle of operation at both initial concentrations (i.e. 100 and 200 mg L−1 of initial aniline, ). Meanwhile, COD removal efficiency of the SBR was also excellent, where the outlet COD concentrations were below 45 mg L−1 in most cycles, which resulted in average removal efficiencies of 94.9 ± 1.4 and 94.0 ± 1.3% from day 61 to 80 and 81 to 100 when the influent aniline concentrations were 107.3 ± 12.3 and 213.6 ± 18.3 mg L−1, respectively (). In this study, COD consumption (including both acetate and aniline) mainly took place in oxic phase, while slight COD decrease could still be found in anaerobic and anoxic conditions along with heterotrophic denitrification.
(Stage 3).
Although aerobic aniline biodegradation always leads to NH4+-N increase in aniline-rich wastewater treatment processes and nitrogen removal efficiencies were relatively low in most studies [Citation1], the results in the present work showed satisfactory nitrogen removal at the end of each cycle under A/O/A mode, which is presented in . More than 83% of NH4+-N was removed in most cases within 6-h cycle. At the end of the cycle, there was almost no NO2–N in the effluent. As for TN, the average removal efficiencies from day 61 to 80 and from day 81 to 100 with average influent aniline concentration of 107.3 ± 12.3 and 213.6 ± 18.3 mg L−1 were 83.3 ± 2.8 and 72.5 ± 3.3%, respectively. When comparing the concentration of TN and TIN [the sum of NH4+-N, NO2–N and NO3–N) in the system before and after treatment, it can be found that the concentration of TN was much higher than TIN at the beginning of the cycle while their concentrations were almost the same at the end of the cycle. This indicated that there was nearly no nitrogen-containing organics in residual COD and the nitrogen in aniline was transferred to inorganic form. Besides, nitrogen bio-assimilation could also happen and synthetize some essential cellular components. In the present work, the nitrogen removal rate via bio-assimilation was approximately 10%, estimated according to 19. There was also a significant decrease in TP concentration during 6 h cycle in the presence of aniline, which is shown in . With the two different levels of inlet aniline concentrations (100 and 200 mg L−1], the TP removal efficiency was consistently over 84% during 40 days of operation. The removal of nitrogen was found to be improved and P reduction was also considered in this study compared with some previous reports treating aniline wastewater [Citation4,Citation23].
Figure 3. Nitrogen and phosphorus removal from aniline-containing wastewater with A/O/A mode (Stage 3)
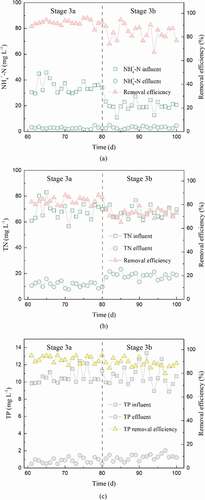
To summary, simultaneous removal of different pollutants occurred with the following process: within each cycle, the majority of aniline and COD were reduced through aerobic biodegradation (oxic phase). The removal rate of initial COD within anaerobic, oxic and anoxic phase were ~15%, 74% and 3%, respectively. NH4+-N removal in the system mainly depended on aerobic nitrification (the residual concentration at the end of oxic phase could be less than 3 mg L−1) through which NH4+-N was converted to NO3–N. NO3–N was further reduced by denitrification during anoxic phase, resulting in remarkable TN reduction (~15% and 34% of the initial TN could be removed within anaerobic and anoxic phase, respectively). PAOs decomposed intracellular polyphosphate and released phosphate in anaerobic condition along with the organic consumption by released energy. Biological phosphorus removal can be explained by two mechanisms: One is aerobic phosphorus uptake (oxic condition) and the other is denitrifying phosphorus removal (anoxic condition) [Citation14]. In this study, phosphorus reduction was observed in both oxic and anoxic phases (~80% and 10% of the initial TP could be removed within oxic and anoxic phase, respectively), which indicated that both PAOs and DNPAOs could coexist in the SBR. In common biological processes, denitrifying bacteria compete with PAOs for carbon sources for denitrification. In the present study, after the accumulated NH4+-N from aniline biodegradation was transferred by nitrification, the enrichment of DNPAOs before treating aniline wastewater could reduce the need for external carbon augmentation and potentially improved simultaneous nitrogen and phosphorus removal in anoxic environment [Citation24]. Therefore, the removal of nitrogen and phosphorus could be improved during aniline wastewater treatment. With the combination of different processes such as nitrification and denitrifying phosphorous accumulation, the SBR was found to have high removal efficiencies of organics, nitrogen and phosphorus during treating aniline wastewater.
Microbial community analysis
The bacterial populations in the sludge samples during the start-up of the SBR system for treating aniline wastewater was determined to analyze the microbial community succession with the variation of working conditions of the reactor. Samples S1, S2, and S3 were collected from different stages of the system (i.e. seeding, enhanced nutrient reduction stage and aniline wastewater treatment stage, respectively) and the numbers of effective sequences in these three samples were 35,169, 37,625, and 44,728, with average lengths of 432, 440, and 436 bp for S1, S2 and S3, respectively. The microbial community diversity varied significantly during the start-up of the SBR, where only 23.9% of total operational taxonomic units were observed to be shared among the three samples.
Regarding the phylum-level distribution (), the prevailed groups in the seed sludge (S1) were Proteobacteria (37.60%), Firmicutes (13.99%), Candidate division TM7 (12.61%), Actinobacteria (11.29%), Bacteroidetes (8.18%), Acidobacteria (4.09%), and Chloroflexi (4.08%). As for Samples S2 and S3, the most abundant cluster was also affiliated to phylum Proteobacteria, representing 73.86 and 39.58% of the whole microbial community, indicating it important roles for wastewater treatment [Citation2; Citation23]. However, the decrease of Proteobacteria after the influent was shifted to aniline wastewater might be attributed to microbial growth inhibition to some microbial groups by the presence of aniline. Besides Proteobacteria, significant abundance variations of other phyla were also observed after the SBR was fed with aniline-containing wastewater. The relative abundances of phyla Bacteroidetes and Firmicutes were higher in stage 3 as compared with stage 1 and 2 since sub-groups within phylum Firmicutes including Bacillus, Clostridium, etc. were found to be able to degrade aromatic compounds such as nitrobenzene and roxarsone [Citation25], and Bacteroidetes species could be responsible for removal of aromatic pollutants and nitrogen [Citation23].
Figure 4. Relative abundances of bacterial groups in the three sludge samples at phylum level (a) and class level (b), and main functional genera in the SBR at different stages (c)
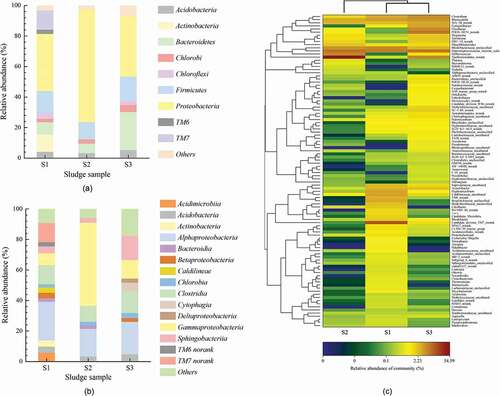
The microbial community structure at class level was also investigated. The main identified classes were described in . In the seed sludge, the most abundant group was Alphaproteobacteria, accounting for 25.34% of the total reads. Besides, Candidate division TM7 norank (12.61%), Clostridia (12.56%), Gammaproteobacteria (7.74%), Acidimicrobiia (5.72%), Sphingobacteriia (4.52%), Actinobacteria (4.05%) and Acidobacteria (4.05%) were also found to be the predominant classes in S1. In sample S2, more than 54.49% of the sequences belonged to class Gammaproteobacteria. Classes Alphaproteobacteria and Clostridia also showed high abundances, the proportions of which were 18.03 and 10.39%, respectively. These top three classes made up 82.92% of the total bacterial populations, suggesting the enrichment of dominant functional groups in the reactor. With respects to sample S3, the most prevalent bacterial group at class level was Alphaproteobacteria (21.22%), followed by Sphingobacteriia (15.78%), Clostridia (14.58%), Gammaproteobacteria (12.21%), Cytophagia (5.30%), Acidobacteria (4.81%), and Chlorobia (3.30%). It was noted that in spite of the uniform of major classes, the proportions of these dominant bacterial clusters in the three samples were remarkably different. For instance, the relative abundance of Gammaproteobacteria strains in S2 was the highest among the three samples while in S3 more bacterial groups belonging to Alphaproteobacteria and Sphingobacteriia were detected. The shift of the most popular Proteobacteria subgroup from Gammaproteobacteria to Alphaproteobacteria after the reactor was fed with aniline-containing influent suggested that class Alphaproteobacteria, which can also be predominant in previous publication related to aniline wastewater treatment [Citation26], had higher tolerance to the presence of aromatic pollutants in the wastewater than Gammaproteobacteria in the present work. In addition, it can be noted that in the present SBR, the proportion increase of phylum Bacteroidetes during treatment of aniline-containing wastewater could be largely attributed to the remarkable increase of its sub-group namely class Sphingobacteriia which could be involved in the aromatic removal [Citation27], and have denitrifying phosphate accumulating potential [Citation28].
shows the predominant functional bacterial population distribution at genus level. Some of the genera were detected in all the three samples, such as Blastocatella, Macellibacteroides, and Caldilineaceae uncultured. Genus Xanthomonadales norank was found to be enriched in sample S2 and S3 during the operation, which could be beneficial for nitrification. After fed with aniline-containing wastewater in stage 3, the microbial community showed an obvious change. Genera such as Blastocatella, Ferruginibacter and Clostridium became prevalent bacteria groups and genus Pseudomonas was enriched along with the addition of aniline. This might be due to their capacity of utilizing aromatics as sole source of carbon, nitrogen and energy. Specifically, some species belonging to Ferruginibacter are found to be able to hydrolyze several kinds of organic pollutants [Citation29]. Therefore, Ferruginibacter were highly enriched when treating aniline wastewater in the present work. Besides species capable of degrading organic matters such as aniline, there were also some sequences detected in sample S3 representing bacteria with other metabolic functions, which could cooperate for pollutant removal in the SBR. For instance, genus Flexibacter, which was detected in a lab-scale anammox membrane bioreactor [Citation30], could reduce nitrate to nitrite and further to nitrous oxide with organic compounds. Genus Comamonas, which could act as denitrifying bacteria [Citation28], was also detected in S3 in the present study. In addition, several species responsible for phosphorus removal under aerobic/anoxic phase such as Aeromonas and Acinetobacter were found in S3. Aeromonas could accumulate phosphorus and Acinetobacter has previously been characterized as DNPAOs. These results indicated that aniline-degrading bacteria, denitrifying bacteria, PAOs as well as DNPAOs could coexist and was enriched in the present system.
Conclusions
Through enrichment of DNPAOs under A/A/O mode and the further operation of A/O/A mode, the SBR could not only remove organic matters (removal efficiency > 91.6%) but also notably reduce NH4+-N released from aniline (removal efficiency > 76.9%). The average effluent concentration of COD, NH4+-N, TN, and TP with influent containing 200 mg L−1 of aniline was 37.6, 3.2, 18.6, and 1.3 mg L−1, respectively and effluent aniline was under detection limit. Functional groups such as Acinetobacter, Comamonas, Ferruginibacter, and Pseudomonas were found to be tolerant to the inhibition effect of aniline and could play important roles for pollutant reduction in the system. The excellent simultaneous removal of organics, nitrogen, and phosphorus in the SBR showed its great application potential for treating aniline-rich industrial effluents.
Acknowledgments
This work was partially supported by the National ‘Twelfth Five-Year’ Plan for Science & Technology Pillar Program [Grant number 2015BAL01B02] and by the National Nature Science Foundation of China [Grant number 5188237].
Disclosure statement
No potential conflict of interest was reported by the author(s).
References
- Dvořák L, Lederer T, Jirků V, et al. Removal of aniline, cyanides and diphenylguanidine from industrial wastewater using a full-scale moving bed biofilm reactor. Process Biochem. 2014;49(1):102–109.
- Jiang Y, Liu Y, Zhang H, et al. Aerobic granular sludge shows enhanced resistances to the long-term toxicity of Cu(II). Chemosphere. 2020;253:126664.
- Jiang Y, Shang Y, Gong T, et al. High concentration of Mn2+ has multiple influences on aerobic granular sludge for aniline wastewater treatment. Chemosphere. 2020;240:124945.
- Fan Y, Chen X, Yao Z, et al. A novel inhibition mechanism of aniline on nitrification: aniline degradation competes dissolved oxygen with nitrification. SciTotal Environ. 2021;770:145205.
- Nguyen TM, Kim J. Sphingobium aromaticivastans sp. nov., a novel aniline- and benzene-degrading, and antimicrobial compound producing bacterium. Arch Microbiol. 2019;201(2):155–161.
- Lee S, Kim G, Yun SH, et al. Proteogenomic characterization of monocyclic aromatic hydrocarbon degradation pathways in the aniline-degrading bacterium Burkholderia sp. K24. Plos One. 2016;11(4):e154233.
- Zhang Y, Luo P, Zhao S, et al. Control and remediation methods for eutrophic lakes in the past 30 years. Water Sci Technol. 2020;81(6):1099–1113.
- Zekker I, Artemchuk O, Rikmann E, et al. Start-up of anammox SBR from non-specific inoculum and process acceleration methods by hydrazine. Water (Basel). 2021;13(3):350.
- Jiang Y, Shi X, Ng HY. Aerobic granular sludge systems for treating hypersaline pharmaceutical wastewater: start-up, long-term performances and metabolic function. J Hazard Mater. 2021;412:125229.
- Zekker I, Raudkivi M, Artemchuk O, et al. Mainstream-sidestream wastewater switching promotes anammox nitrogen removal rate in organic-rich, low-temperature streams. In: Environmental Technology. 2020. p. 1–10.
- Choi D, Kim J, Youn B, et al. Feed distribution based on sensing ammonium concentration after sub-feeding to achieve target effluent nitrogen concentration in sequencing batch reactors. Int Biodeterior Biodegrad. 2018;133:187–193.
- Trojanowicz K, Plaza E, Trela J. Pilot scale studies on nitritation-anammox process for mainstream wastewater at low temperature. Water Sci Technol. 2016;73(4):761–768.
- Li Z, Zhu Y, Zhang Y, et al. Characterization of aerobic granular sludge of different sizes for nitrogen and phosphorus removal. Environ Technol. 2019;40(27):3622–3631.
- Mielcarek A, Rodziewicz J, Janczukowicz W, et al. Effect of the C:N:P ratio on the denitrifying dephosphatation in a sequencing batch biofilm reactor (SBBR). J Environ Sci (China). 2015;38:119–125.
- Mandel A, Zekker I, Jaagura M, et al. Enhancement of anoxic phosphorus uptake of denitrifying phosphorus removal process by biomass adaption. Int J Environ Sci Technol. 2019;16(10):5965–5978.
- Zhang S, Huang Y, Hua Y. Denitrifying dephosphatation over nitrite: effects of nitrite concentration, organic carbon, and pH. Bioresour Technol. 2010;101(11):3870–3875.
- Jiang Y, Wei L, Yang K, et al. Rapid formation of aniline-degrading aerobic granular sludge and investigation of its microbial community succession. J Clean Prod. 2017;166:1235–1243.
- Standard Methods for the Examination of Water and Wastewater. 21st. Washington DC, USA: American Public Health Association/American Water Works Association/Water Environment Federation; 2005.
- Guo Y, Wang Y, Zhang Z, et al. Physiological and transcriptomic insights into the cold adaptation mechanism of a novel heterotrophic nitrifying and aerobic denitrifying-like bacterium Pseudomonas indoloxydans YY-1. Int Biodeterior Biodegrad. 2018;134:16–24.
- Zhang C, Zhang H, Yang F. Diameter control and stability maintenance of aerobic granular sludge in an A/O/A SBR. Sep Purif Technol. 2015;149:362–369.
- Wang X, Zhao J, Yu D, et al. Stable nitrite accumulation and phosphorous removal from nitrate and municipal wastewaters in a combined process of endogenous partial denitrification and denitrifying phosphorus removal (EPDPR). Chem Eng J. 2019;355:560–571.
- Zhang Y, Li M, Zhang Q, et al. Start-up performance of anaerobic/aerobic/anoxic-sequencing batch reactor (SBR) augmented with denitrifying polyphosphate-accumulating organism (DPAO) and their gene analysis. Water Sci Technol. 2018;78(3):523–533.
- Li Y, Zhang Q, Li M, et al. Bioaugmentation of sequencing batch reactor for aniline treatment during start-up period: investigation of microbial community structure of activated sludge. Chemosphere. 2020;243:125426.
- Zhang M, Peng Y, Wang C, et al. Optimization denitrifying phosphorus removal at different hydraulic retention times in a novel anaerobic anoxic oxic-biological contact oxidation process. Biochem Eng J. 2016;106:26–36.
- Guzmán-Fierro VG, Moraga R, León CG, et al. Isolation and characterization of an aerobic bacterial consortium able to degrade roxarsone. Int J Environ Sci Technol. 2015;12(4):1353–1362.
- Jiang Y, Wei L, Yang K, et al. Investigation of rapid granulation in SBRs treating aniline-rich wastewater with different aniline loading rates. SciTotal Environ. 2019;646:841–849.
- Silva Moretto JA, Rueda Furlan JP, Tonelli Fernandes AF, et al. Alternative biodegradation pathway of the herbicide diuron. Int Biodeterior Biodegrad. 2019;143:104716.
- Liu Y, Yuan Y, Li X, et al. Succession of bacterial community in anaerobic–anoxic–aerobic (A2O) bioreactor using sludge fermentation liquid as carbon source. Desalin Water Treat. 2015;54(4–5):1061–1069.
- Tanaka R, Nouzaki K, Navarro RR, et al. Activated sludge microbiome in a membrane bioreactor for treating Ramen noodle-soup wastewater. J Gen Appl Microbiol. 2020;66(6):6.
- Gonzalez-Martinez A, Osorio F, Rodriguez-Sanchez A, et al. Bacterial community structure of a lab-scale anammox membrane bioreactor. Biotechnol Prog. 2015;31(1):186–193.