ABSTRACT
Benzo[a]pyrene (BaP) is widely used as a reference compound when studying the toxicity of Polycyclic aromatic hydrocarbons (PAHs) to natural communities. In this study, 15d after exposure to BaP at the nominal concentrations of 5, 1 and 0.2 μg/L, liver, gill and mantle tissues of Crassostrea gigas and Mytilus coruscuswere taken for BaP uptake assessment, and Cytochrome P450 (CYP450) gene expression level and 7-ethoxyresorufifififin O-deethylase (EROD) activity testing, respectively. CYP450 gene expression level and EROD activity increased significantly with the increase of BaP exposure concentrations. The Significant correlations between BaP uptake and both CYP450 gene expression level and EROD activity indicates that they can be used as a suitable tools for the study of comprehensive levels of biomarker exposed to PAH. There were significant differences in the measurement results of these tissue antioxidant defenses parameters between different treatments.
1. Introduction
Increasing industrial developments and marine shipping traffic near coastal area, and there are concerns about health issues related to seafood pollution. Polycyclic aromatic hydrocarbons (PAHs) was one of the most common organic pollutants in the marine aquatic environments. The common sources of PAHs in environment could be subdivided into natural process and human activities [Citation1]. PAHs had a variety of adverse effects on aquatic organisms, including carcinogenic, immunity, histopathology, and genetic, reproductive and developmental effects [Citation2]. Benzo[a]pyrene (BaP) was a substance of PAH, which is lipophilic and resistant to degradation [Citation3]. BaP had strong mutagenic, teratogenic and carcinogenic effected on different species [Citation4,Citation5].
Single or composite PAHs such as tritium, fuel oil and natural gas could cause a significant decrease in the stability of lysosomal membrane in different tissues of marine mussels (Mytilus spp.) decline in stability, affecting immune and defense against PAHs stressed by altering cellular immune response [Citation6–8]. Most studies had shown that when shellfish were exposed to PAHs, the activity of oxidase kinases or PAHs metabolic enzymes (such as cormorants, scallops, mussels and so on) increases significantly [Citation9]. The activity of similar enzyme under PAHs stresses would show significantly tissue/cell specificity and enzyme response specificity. These specificities were closely related to the function of biological tissues, the biochemical reactions involved in enzymes, and the regulation of enzyme expression [Citation10]. The enzymatic systems including digestive enzymes and antioxidant enzymes, not only played a key role in maintaining the normal physiological metabolic regulation in the body, but were also very sensitive to environmental stress [Citation11]. The damage to organisms at the level of individuals, tissue and cell level was caused by changes in the specific gene expression or genetic structure. Taking BaP as an example, epoxidized benzopyrene (BPDE), a metabolite in living organisms, could be directly combined with nucleic acids to generate DNA adducts, as well as the DNA strands were broken or changed in base sequence. The metabolite BaP as a series genes with important functions (such as proto-oncogenes and tumor suppressor genes), could activate or inhibit expression, which may lead to a series of genotoxicity, and even toxic effects such as carcinogenesis and lethality [Citation12]. Cytochrome P450 family 1 (CYP1) was involved in the biotransformation of PAHs. PAHs could induce CYP1 protein expression and enzyme activity, the latter of which was usually quantified as 7-ethoxyresorufifin O-deethylase (EROD) activity [Citation13]. When the BaP concentration reached a certain level, the activity of EROD would remain at a stable level, indicating that the organism’s metabolic capacity for BaP had reached its maximum at this time [Citation14]. Several studies had demonstrated CYP1-related enzyme activity, protein expression and CYP1-like transcript levels in bivalve mollusks, using these parameters as biomarkers was considered an excellent method for contamination monitoring [Citation15,Citation16], such as BaP hydroxylase and EROD [Citation17]. EROD activity was closely related to the presence of CYP1A, P450-dependent oxidative metabolism leaded to the production of reactive oxygen species (ROS), which was considered to be an important mechanism of pollutant-mediated aquatic toxicity [Citation18]. In molluscs, CYP proteins expression and CYP1-related enzyme activity had been demonstrated by total CYP level, BaP hydroxylase and EROD activity [Citation19].
The purpose of this study was to investigate the response to two bivalve species Crassostrea gigas andMytilus coruscus to the expression of CYP450 gene and EROD activity in the liver, gills and mantles under the influence of BaP. Furthermore, the contents of BaP in the liver, gills and mantle of two bivalve species were also determined to explore the biological responses of the bivalve to bioaccumulation.
2. Materials and methods
2.1. Chemicals
BaP (purity, 99%) was purchased from the Sigma Company, USA; heparin sodium salt, acetone and methanol from Sinopharm Chemical Reagent Co, Ltd., China; and all the chemicals used were analytical grade.
2.2. Animals and experimental exposure
C. gigas and M. coruscus major was obtained from the Zhoushan bivalve farm in Zhejiang Province, China. The mean weight and length of two bivalve species were 102.33 ± 24.68 g and 10.64 ± 2.23 cm (mean ± SD). The bivalves were acclimated to laboratory conditions with a temperature of 20 ± 1°C, salinity of 31.2 ± 1.3‰ and pH 7.9 ± 0.2 for 20 days prior to the experiment in 100 L glass tanks containing seawater treated using sand filtration, kept on a natural daylight cycle. Adored powder of Spirulina platensis (30 mg for each individual per day) was fed with daily during experimental periods.
Samples of C. gigas and M. coruscus were randomly selected and divided into three groups, respectively, each treatments groups of three different BaP concentration groups (5, 1 and 0.2 μg/L) and control group (natural seawater). There were approximately 60 bivalve samples in each group of supplied with oxygen 24 h per day. The seawater was changed a solutions of the same concentration once a day. Eliminate the faces and samples of less vitality in time. A certain amount of independently cultured microalgae was fed twice each day. The density of microalgae was 2 × 104 cell/mL. The stress experiment lasted for 15 days, and samples were taken at 1, 3, 9 and 15 days after the end of the domestication period. The gills, mantles and liver were sampled for each day, including the control group, respectively. The bivalves were sampled and mixed separately for enzyme assaysis.
2.3. Sample preparation
10 g of bivalve tissues for homogenization was taken, saponify with 2 N methanolic KOH (MeOH:H2O, 9:1), and extract with cyclohexane. The extracts were purified through a column containing glass fiber, florisil (deactivated with 3% water), and anhydrous sodium sulfate. The eluates were dried under a stream of nitrogen and dissolved with 1 ml of hexane before analysis [Citation20]. The content of BaP in the liver, mantle and gills fractions was determined by gas chromatography (GC) coupled to mass spectrometry [MS) based on the method described by [Citation21]. The detection limits of BaP in tissues was approximately 50 pg/g for [Citation22].
After the end of each exposure interval, the four bivalves in each treatment were carefully dissected out, and their livers, gills and mantles were washed with cold physiological saline (0.9% NaCl) and weighed. These tissues were homogenized (1:10, w/v) in the chilled physiological saline. The homogenate was centrifuged at 4000 g for 15 mins at 4°C, and the supernatants were collected and diluted to different concentrations to evaluate various biochemical parameters [Citation23].
2.4. Transcriptional analysis of CYP450 in tissue
For CYP450 analysis, the samples of gills, mantles and livers were washed with double distilled water and dissected. The determination method of CYP450 activity was measured according to Vasantha-Srinivasan et al. [Citation24]. RT-qPCR was used to detect the expression levels of gills, mantles and liver damage-related genes. The primers sequences are shown as follows .
Table 1. Name and sequence of the target gene amplified with primers
2.5. In vivo live-imaging EROD assay
According to Danion [Citation25], the EROD activity in three tissues was measured as described by microplate fluorescence spectrophotometry. The methoxyfuran fluorescence was measured by the resorufin at 60s intervals over a 13 min total scan time. The enzyme activity was expressed as pmol/min/mg of protein. EROD activity describes the rate of the CYP1A1 and 1B1 mediated deethylation of the substrate 7-ethoxyresorufin to form the product resorufin, and can be quantified by fluorescence detection [Citation26]. All samples were assayed in duplicate.
2.6. Statistical analysis
For each data set, the assumptions of analysis of variance (ANOVA), normality and equal variance, were checked using Shapiro Wilks and Levene tests, respectively. If the data set to meet the hypothesis, a one-way ANOVA is performed and was conducted and an apost-hocmean comparison test (Tukey’s test) is performed in a significant place. If the data set does not meet the assumptions, Kruskal–Wallis and Mann Whitney (one-tail) tests are used to determine significant differences. Pearson and Spearman correlation tests were used to analyze the correlation between biomarkers and BaP. All statistical analyses were conduct data alpha level of 0.05.
3. Results and discussion
3.1. BaP uptake in liver, gill and mantle tissues of two bivalve species
The BaP was rapidly absorbed, and these compounds could be detected in most tissues after the introduction of the hydrocarbon (). showed the standard deviation and the range of values for the BaP uptake experiments; 4 bivalves of two species were taken from each time period and analyzed separately. The distribution of BaP content in different tissues of C. gigas and M. coruscus was consistent. From the data in , it showed that the BaP accumulation in the three groups of two bivalve species was basically higher than that in the control group, and with the extension of the experimental time, the content of the outer membrane gradually increases, and significant difference with the BaP content of the gill tissues and liver tissues. The important accumulation of BaP was in gills, mantles and livers may be related to the functions of these organs [Citation27]. Mussels feed by filtering seawater, the process was as follows that the water flows through the gills firstly, and then the largest particles settle on the outer membrane, and particles of appropriate sized entered the digestive organs [Citation28]. PAHs dissolved in water or adsorbed on suspended particles can enter tissue with water, which bring the pollutants to enter the tissues. Due to the different biochemical components and physiological characteristics of different tissues, the BaP content of each tissue was also different [Citation29]. The gills were covered with a layer of dividing epithelial cells, which could easily contact the toxic substances scattered in the water body. At the same time, it could spread through the venom in a large area [Citation30], so the content of PAHs in the gills fluctuates greatly. Comparing with other tissues, the visceral tissue contained a large number of enzymes capable of metabolizing PAHs into substituent-containing compounds, so its PAHs matrix content was low; PAHs entered the internal tissues attached to the outer epithelium, and the main emission of absorbing membranes which was performed by exudation of blood cells and secretion of shells [Citation27]. This effect was weaker than that of enzymes, causing more BaP to accumulate in the outer membrane [Citation31].
Table 2. The concentration of BAP in different tissues of Crassostrea gigas and Mytilus coruscus.
3.2. CYP450 gene expression level and EROD activity in gill, mantle and liver tissues of Crassostrea gigas and Mytilus coruscus
The CYP450 gene expression level of C. gigas and M. coruscus and the statistical results after exposure were shown in . BaP exposure induced CYP450 gene expression level under all exposure conditions. There was no significant difference in the increased CYP450 gene expression level between the two bivalve species after 1–15 days stage exposure the same concentration BaP (p > 0.05). CYP450 gene expression of C. gigas and M. coruscus exposed to the BaP increased with concentrations. It is worth to note that in the two high concentration groups treatments (5 and 1 μg/L), the CYP450 gene expression level increased at 1–9 days period, as well as the level decreased of 15-day experiment stage, and the level value was significantly higher than that of the control groups (p < 0.05). On the contrary, an increase was noted in the lowest treatment groups (0.2 μg/L) throughout the experiment, no significant increase in the CYP450 gene expression level compared to control groups. In liver and gill, it is not used as the mantle, the CYP450 gene expression was obviously induced throughout the experiment.
Figure 1. The effects of BaP on the CYP450 relative gene expression level of Crassostrea gigas.
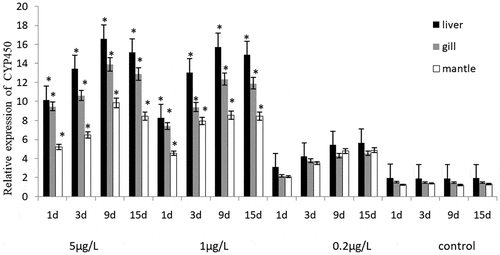
Figure 2. The effects of BaP on the CYP450 relative gene expression level of Mytilus coruscus.
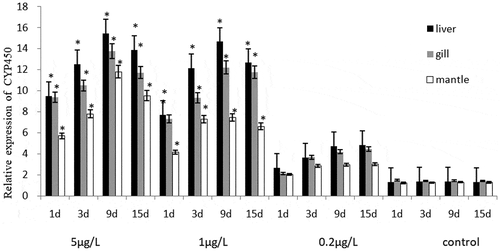
The CYP450 gene expression level and EROD activity of C. gigas and M. coruscus showed similar trends, and the EROD activities of the liver, gill and mantle was evaluated after exposure (). Significant induction to the liver and gill tissues EROD activity was observed in two bivalve species to exposure the highest and middle concentrations of BaP, except for the BaP 0.2 μg/L group. Regarding the effects of two high concentrations of BaP (5 μg/L and 1 μg/L) on the oxidative defense of the biochemical expression of EROD activity concentration, their levels increased by more than one and a half fold increase corresponding control groups. Compared with the control group, the EROD activities of 0.2 μg/L BaP groups were increased slightly after 1–15 days, and the EROD activity of the two bivalve species was significantly induced in exposed to BaP from 1 to 5 μg/L for 1–9 day, followed with decreased at 15-day stage. There was no significant difference in gills, liver and mantles EROD activity between the two bivalve species under the exposed to the same concentration BaP though out the experiment. There was no significant difference between the EROD activities of liver and gill groups, but the EROD activities of the two tissues were higher than that of mantle tissues.
3.3. Correlation between EROD activity and CYP450 gene expression and BaP uptake
Linear regressions analysis showed that there was an extremely high correlation between the tissue BaP uptake and the EROD activity, as well as the tissue BaP uptake and CYP450 gene expression level in the liver, gill and mantle (). Pearson product moment was R2 = 0.914 ~ 0.981 for EROD (R2 = 0.864 ~ 0.940) activity and CYP450 gene expression (R2 = 0.835 ~ 0.962), both as function of the tissue BaP uptake, respectively. BaP can cause active oxygen and oxidative stress in the vital organs of bivalves. In the present study, the changes trends of CYP450 gene expression level and CYP450 gene expression level in three tissues of two bivalve species were basically the same, which indicated that PAHs can induce CYP1 protein expression and enzyme activity, the latter is usually EROD [Citation13].
Table 3. Linear regressions of EROD activity and CYP450 as function of BaP uptake; and CYP450 gene expression as function of EROD activity
In this study, the correlation coefficient between quantitative BaP and two biomarkers (EROD activity and CYP450 gene expression) was higher in liver tissues. The activity of similar enzyme under PAHs stress showed obvious tissue/cell specificity and enzyme response specificity. These specificities were closely related to the function of biological tissues, the biochemical reactions involved in enzymes, and the regulation of enzyme expression [Citation10].
The CYP450 gene expression level was significantly positive correlations between the concentration of EROD activity (p < 0.05), and was significantly positively correlated with the spontaneous responses to environmental pollutants (). The functional relationship between EROD activity was R = 0.910 ~ 0.972 (R2 = 0.829 ~ 0.945) for CYP450 gene expression level. Vertebrate cytochrome P450 1(CYP1) enzymes metabolize endogenous and xenobiotic compounds and usually demonstrated a substrate-inducible response. EROD was a commonly used method to determine the CYP1 enzymes activity in these organisms. The EROD activity observed in gill microsomes of oyster C. gigas correlated with CYP1-like 1 and CYP1-like 2 transcription levels [Citation32]. The EROD activity and CYP450gene expression of the experimental groups were higher than those in the control group, showing a certain dose-effect relationship. During the 1–9d experiment period, the EROD activity and CYP450 expression increased significantly of both concentrations BaP (5 μg/L and 1 μg/L) groups (p < 0.05) and they were consequence of the induction of CYP450 in the transcription of P4501A1 mRNA rapidly activated, which promoted protein synthesis and significantly improves the results of EROD activity with the rapid enrichment of BaP in tissues. EROD activity in cells was a well established in vitro and in vivo biomarker of aryl hydrocarbon receptor (AhR)-mediated induction of CYP1A and a sensitive indicator of specific planar polycyclic aromatic hydrocarbons (PAHs) and structurally related compounds [Citation33,Citation34]. Although BaP induction was still present, the EROD activity and CYP450 expression of the two experimental groups (5 μg/L and 1 μg/L) decreased gradually with increasing time after 9 days. When the BaP concentration reached a certain level, the activity of EROD would be maintained at a stable level, indicating that the organism’s metabolic capacity for BaP had reached its maximum at this time [Citation14]. Rees et al. [Citation35], observed in Salmo salar exposure that the introduction of β-naphthoflavone (BNF) into corn oil resulted in limited introduction of CYP1mRNA by environment pollutants. The production of P4501A1 mRNA transcripts may have reached a kinetic limit of present experiment. Moreover, BaP could cause changes in the configuration of P4501A1 of organisms, which could cause lipid peroxidation of the liver and other tissues damage [Citation36Citation37], while the expression of CYP1Al mRNA was inhibited from reducing the EROD activity of the tissues. However, during the entire experimental period, the EROD activity and CYP450 gene expression of the three tissues with the lowest concentration experimental groups (0.2 μg/L) continued to increase, and they were still higher than those in the control group. This indicated that the tissues of the two bivalve species were suffered a certain degree of toxic damage, but this damage had not yet reached the critical value of causing substantial damage as the toxic metabolic system was still functioning.
4. Conclusions
The results of the present study demonstrated that C. gigas and M. coruscus respond satisfactorily as a biological indicator of BaP exposure. The present study showed CYP450 gene expression level and EROD activity in liver, gills and mantles of C. gigas and M. coruscus. Although the EROD activity was low, it indicated that a CYP450 like enzyme probably catalyzes the 7-ER deethylation. The employment of the CYP450 gene expression level and EROD activity on the liver, gills and mantles of two bivalve species, respectively, provided an effective tool for studying the effects of this exposure. EROD activity and CYP450 gene expression level of two bivalve species exposed to the BaP increased with concentrations. Oxidation defense index was significantly positively correlated with the BaP tissue uptake. Although EROD activity and CYP450 were traditional enzymes biomarkers of PAH, there were many other sensitive biomarkers that can be used as research focus on PAH pollution stress. The environment in which aquatic organisms live is more complicated. In practice, the PAHs exposure is multiple, some studies about combined toxic effects are more valuable. We will pay attention to study the more sensitive biomarkers and the combined toxic of other pollutants in the future research.
Ethical approval
There are no involving human participants of all procedures performed in this study. This article does not contain any studies with human participants or animals performed by any of the authors.
Informed consent
Informed consent was obtained from all individual participants included in the study. Additional informed consent was obtained from all individual participants for whom identifying information is included in this article.
Disclosure statement
No potential conflict of interest was reported by the author(s).
Additional information
Funding
References
- Haritash AK, Kaushik CP. Polycyclic aromatic hydrocarbons (PAHs) and environmental health. Our Earth. 2006;3(3):1–7.
- Driscoll SBK, Mcardle ME, Menzie CA, et al. A framework for using dose as a metric to assess toxicity of fish to PAHs. Ecotoxicol Environ Saf. 2010;73(4):486–490.
- Song Q, Zheng P, Qiu L, et al. Toxic effects of male Perna viridisgonad exposed to BaP, DDT and their mixture: a metabolomic and proteomic study of the underlying mechanism. Toxicol Lett. 2015;240(1):185–195.
- Chen H, Diao X, Zhou H. Tissue-specific metabolic responses of the pearl oyster Pinctada martensii exposed to benzo[a]pyrene. Mar Pollut Bull. 2018;131(Pt A):17–21.
- Thompson KL, Picard CR, Chan HM. Polycyclic aromatic hydrocarbons (PAHs) in traditionally harvested bivalves in northern British Columbia, Canada. Mar Pollut Bull. 2017;121(1–2):390–399.
- Avio CG, Gorbi S, Milan M, et al. Pollutants bioavailability and toxicological risk from microplastics to marine mussels. Environ Pollut. 2015;198:211–222.
- Gomiero A, Volpato E, Nasci C, et al. Use of multiple cell and tissue-level biomarkers in mussels collected along two gas fields in the northern Adriatic Sea as a tool for long term environmental monitoring. Mar Pollut Bull. 2015;93(1–2):228–244.
- Hannam ML, Bamber SD, Galloway TS, et al. Effects of the model PAH phenanthrene on immune function and oxidative stress in the haemolymph of the temperate scallop Pecten maximus. Chemosphere. 2010;78(7):779–784.
- Li L, Jiang M, Shen X. Variability in antioxidant/detoxification enzymes of Sinonovacula constricta exposed to benzo[a]pyrene and phenanthrene. Mar Pollut Bull. 2016;109(1):507–511.
- MariaVL, Bebianno MJ. Antioxidant and lipid peroxidation responses in Mytilus galloprovincialis exposed to mixtures of benzo(a)pyrene and copper. Comp Biochem Physiol C Toxicol Pharmacol. 2011; 154(1):56–63.
- Li ZH, Zlabek V, Grabic R, et al. Effects of exposure to sublethal propiconazole on intestine-related biochemical responses in rainbow trout, Oncorhynchus mykiss. Chemico-biol Interactions. 2010;185(3):241–246.
- Van Duursen MBM, Sanderson JT, Van Den Berg M. Cytochrome P450 1A1 and 1B1 in human blood lymphocytes are not suitable as biomarkers of exposure to dioxin-like compounds: polymorphisms and interindividual variation in expression and inducibility. Toxicol Sci. 2005;2015(85):703–712.
- Siebert MN, Mattos Jacó J, Piazza CE, et al. Characterization of ethoxyresorufin O-deethylase activity (EROD) in oyster Crassostrea brasiliana. Comp Biochem Physiol Part B Biochem Mol Biol. 2017a;203:115–121.
- Liu D, Pan L, Li Z, et al. Metabolites analysis, metabolic enzyme activities and bioaccumulation in the clam Ruditapes philippinarum exposed to benzo[a]pyrene. Ecotoxicol Environ Saf. 2014;107:251–259.
- Lüchmann KH, Clark MS, Bainy ACD, et al. Key metabolic pathways involved in xenobiotic biotransformation and stress responses revealed by transcriptomics of the mangrove oyster Crassostrea brasiliana. Aquatic Toxicol. 2015;166:10–20.
- Zanette J, Jenny MJ, Goldstone JV, et al. Identification and expression of multiple CYP1-like and CYP3-like genes in the bivalve mollusk Mytilus edulis. Aquatic Toxicol. 2013;128–129:101–112.
- Zhang Y, Song J, Yuan H, et al. Biomarker responses in the bivalve (Chlamys farreri) to exposure of the environmentally relevant concentrations of lead, mercury, copper. Environ Toxicol Pharmacol. 2010;30(1):19–25.
- Monari M, Foschi J, Matozzo V, et al. Investigation of EROD, CYP1A immunopositive proteins and SOD in haemocytes of Chamelea gallina and their role in response to B[a]P. Comp Biochem Physiol C Toxicol Pharmacol. 2009;149(3):382–392.
- Seabra Pereira CD, Abessa DMS, Choueri RB, et al. Ecological relevance of Sentinels” biomarker responses: a multi-level approach. Mar Environ Res. 2014;96:118–126.
- Balcıoğlu EB. Assessment of polycyclic aromatic hydrocarbons (PAHs) in mussels (Mytilus galloprovincialis) of Prince Islands, Marmara Sea. Mar Pollut Bull. 2016;109(1):640–642.
- Cai YF, Pan LQ, Miao JJ. In vitro study of the effect of metabolism enzymes on benzo(a)pyrene-induced DNA damage in the scallop Chlamys farreri. Environ Toxicol Pharmacol. 2016;42:92–98.
- Cacciatore F, Bernarello V, Boscolo B, et al. PAH (Polycyclic Aromatic Hydrocarbon) bioaccumulation and PAHs/shell weight index in, Ruditapes philippinarum, (Adams & Reeve, 1850) from the Vallona lagoon (northern Adriatic Sea, NE Italy). Ecotoxicol Environ Saf. 2018;148:787–798.
- Ana Beatriz AR, Cássia OF, Cláudia H, et al. Assessment of PAHs occurrence and distribution in brown mussels (Perna perna Linnaeus 1758) subject to different levels of contamination in Brazil. Reg Stud Mar Sci.2017;14:145–151.
- Vasantha-Srinivasan P, Chellappandian M, Senthil-Nathan S, et al. A novel herbal product based on Piper betle and Sphaeranthus indicus essential oils: toxicity, repellent activity and impact on detoxifying enzymes GST and CYP450 of Aedes aegypti Liston (Diptera: culicidae). J Asia-Pacific Entomol. 2018;21(4):1466–1472.
- Danion M, Floch SL, Lamour F, et al. Effects of in vivo chronic exposure to pendimethalin on EROD activity and antioxidant defenses in rainbow trout (Oncorhynchus mykiss). Ecotoxicol Environ Saf. 2014;99:21–27.
- Chahin A, Peiffer J, Olry JC, et al. EROD activity induction in peripheral blood lymphocytes, liver and brain tissues of rats orally exposed to polycyclic aromatic hydrocarbons. Food Chem Toxicol. 2013;56(2):371–380.
- Zuloaga O, Prieto A, Usobiaga A, et al. Polycyclic aromatic hydrocarbons in intertidal Marine Bivalves of Sunderban Mangrove Wetland, India: an approach to bioindicator species. Water Air Soil Pollut. 2009;201(1–4):305–318.
- Doyle JJ, Ward JE, Mason R. An examination of the ingestion, bioaccumulation, and depuration of titanium dioxide nanoparticles by the blue mussel (Mytilus edulis) and the eastern oyster (Crassostrea virginica). Mar Environ Res. 2015;110:45–52.
- Schφyen M, Allan IJ, Ruus A, et al. Comparison of caged and native blue mussels (Mytilus edulis, spp.) for environmental monitoring of PAH. PCB and Trace Metals Marine Environ Res. 2017;130:221–232.
- Grintzalis K, Georgiou CD, Dailianis S. Total thiol redox status as a potent biomarker of PAH-mediated effects on mussels. Mar Environ Res. 2012;81:26–34.
- Foster P, Chacko J. Minor and trace elements in the shell of Patella vulgata (L.). Mar Environ Res. 1995;40(1):70–76.
- Siebert MN, Mattos JJ, Toledo-Silva G, et al. Candidate cytochrome P450 genes for ethoxyresorufin O -deethylase activity in oyster, Crassostrea gigas. Aquatic Toxicol. 2017b;189:142–149.
- Fent K, Bätscher R. Cytochrome P4501A induction potencies of polycyclic aromatic hydrocarbons in a fifish hepatoma cell line: demonstration of additive interactions. EnvironToxicol Chem.2000;19(8):2047–2058.
- Whyte JJ, Jung RE, Schmitt CJ, et al. Ethoxyresorufifin-O-deethylase (EROD) activity in fish as a biomarker of chemical exposure. Crit Rev Toxicol. 2000;30:347–570.
- Rees CB, Mccormick SD, Vanden Heuvel JP, et al. Quantitative PCR analysis of CYP1A induction in Atlantic salmon (Salmo salar). Aquatic Toxicol. 2003;62(1):67–78.
- Sol EM, Porte C, Albaig ESJ. Hydrocarbons, PCBs and DDT in the NW Mediterranean deep-sea fish Mora moro. Deep Sea Res Part I: Oceanogr Res Papers. 2001;48(2):495–513.
- Zheng R, Chen H, Bo J, et al. Joint effects of crude oil and heavy metals on the gill filament EROD activity of marbled rockfish Sebastiscus marmoratus. Ecotoxicol Environ Saf. 2016;132:116–122.